Advancements and Implications of Protein Sequencing
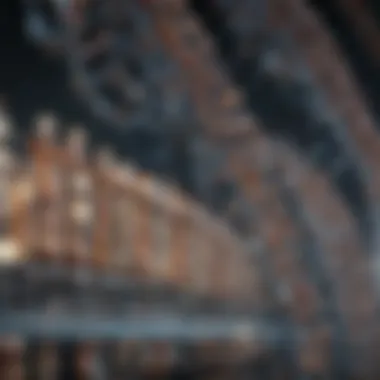
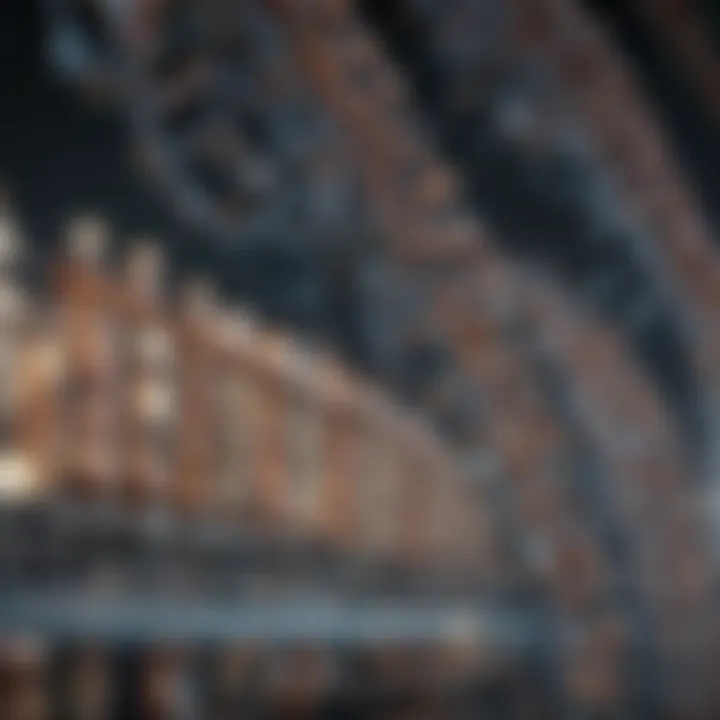
Intro
The realm of protein sequencing technology has transformed significantly in recent decades. Understanding protein structures and functions is central to many fields, including medicine and biotechnology. With advancements in sequencing technology, researchers can now investigate complex proteins more precisely and rapidly.
Protein sequencing allows scientists to decode the building blocks of proteins, which are essential for a variety of biological processes. This work is foundational to developing therapies and understanding diseases at a molecular level. As we delve deeper, it is vital to explore the historical evolution, current methodologies, and implications of this technology.
Research Overview
Summary of Key Findings
Protein sequencing technology has undergone substantial improvements over the years. Key methodologies, such as mass spectrometry and next-generation sequencing, have increased both speed and accuracy in determining protein sequences. Studies show that the integration of these technologies enables scientists to analyze proteins in their native environments, advancing our understanding of their roles in various biological systems.
Moreover, recent research has highlighted how protein sequencing can aid in personalized medicine, allowing for tailored treatments based on individual proteomic profiles. Advances have also been made in biosensors and diagnostic tools, showcasing the versatility of protein sequencing beyond basic research.
Importance of the Research in Its Respective Field
The significance of protein sequencing cannot be overstated. In healthcare, it plays a pivotal role in drug discovery and development. By understanding the precise makeup of proteins involved in diseases, researchers can discover new drug targets. This leads to innovative therapies that are more effective and have fewer side effects.
In biotechnology, the implications extend to areas such as synthetic biology and enzyme engineering. Proteins can be designed for specific functions, which can revolutionize industries from biofuels to agriculture.
Environmental science also benefits from these advancements. Understanding microbial proteins can lead to bioremediation strategies, helping to address pollution and environmental concerns.
Methodology
Description of Analytical Methods Used
The primary methods employed in protein sequencing include mass spectrometry and liquid chromatography coupled with tandem mass spectrometry (LC-MS/MS). Mass spectrometry involves ionizing protein samples and measuring the mass-to-charge ratios of the ions. This allows for precise identification of protein fragments, which can be matched to known sequences in databases.
Next-generation sequencing techniques have also become increasingly popular. These methods involve fragmenting the protein and sequencing the resulting peptides, enabling a comprehensive overview of the proteome. This is particularly valuable for studying complex mixtures of proteins.
Sampling Criteria and Data Collection Techniques
Sample preparation is critical in protein sequencing. Proteins must be extracted from cells or tissues with minimal degradation. This often involves using buffers that stabilize the proteins and prevent protease activity. For instance, using RNase-free reagents ensures the integrity of the samples.
Data collection typically involves generating large datasets, which can then be analyzed using bioinformatics tools. These tools play a vital role in interpreting complex data, identifying protein interactions, and understanding biological significance.
A critical aspect of advancements in protein sequencing technology is the ability to analyze vast datasets efficiently. This has enabled discoveries that were previously unattainable.
Stay tuned as we further explore specific methodologies, applications, and the future landscape of protein sequencing technology.
Intro to Protein Sequencing
Protein sequencing is a crucial area in molecular biology that enables researchers to decipher the amino acid composition of proteins. Understanding these sequences provides insights into protein function, structure, and interaction dynamics within biological systems. With the surge in proteomic research and the development of effective therapeutic strategies, the importance of protein sequencing cannot be overstated. This section elaborates on the foundational concepts of protein sequencing, its significance in biological research, and its relationship to various advanced methodologies.
Definition and Importance
The term "protein sequencing" refers to the process of determining the exact order of amino acids in a protein molecule. This knowledge is pivotal for numerous applications, including drug development, disease diagnostics, and the design of new biotechnological tools. Given that proteins are fundamental to all biological processes, having access to their sequences allows researchers to understand how proteins function and how they can be manipulated for therapeutic purposes.
Moreover, deciphering the sequence of proteins aids in identifying post-translational modifications that can affect their stability and activity. Advances in protein sequencing technology have significantly enhanced our ability to analyze complex protein mixtures, enabling a more comprehensive view of cellular functions.
As the field evolves, the implications of accurate protein sequencing technologies become increasingly critical. They not only influence experimental design but also affect the interpretation of results, contributing to breakthroughs in personalized medicine and tailored drug therapies.
Historical Context
To grasp the current advancements in protein sequencing, it's essential to reflect on the historical development of the field. The journey began in the early 1950s when Frederick Sanger introduced a method for sequencing proteins, primarily through his work on insulin. His work laid the foundation for amino acid sequencing, garnering him two Nobel Prizes, one in Chemistry and another in Physiology or Medicine.
The evolution progressed with the advent of Edman degradation in the 1950s, which allowed sequential identification of amino acids from the N-terminus of proteins. This methodology was widely adopted, but it had limitations regarding the length of peptides that could be accurately sequenced.
In the subsequent decades, mass spectrometry emerged as a game-changing technology. It enabled the analysis of larger and more complex proteins. During the 1990s, the development of tandem mass spectrometry paved the way for high-throughput sequencing methods. Today, next-generation sequencing represents a significant advancement, allowing the sequencing of entire proteomes rather than just individual proteins.
"The field of protein sequencing is not just about understanding structure; it is about unlocking the potential of proteins in health and disease."
Given the dramatic pace of advancements, the evolution of protein sequencing technologies illustrates the interplay between scientific inquiry and technological innovation, which continues to shape our understanding of biology.
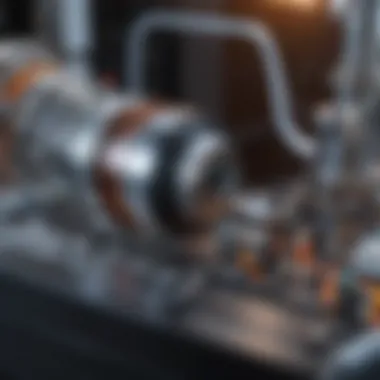
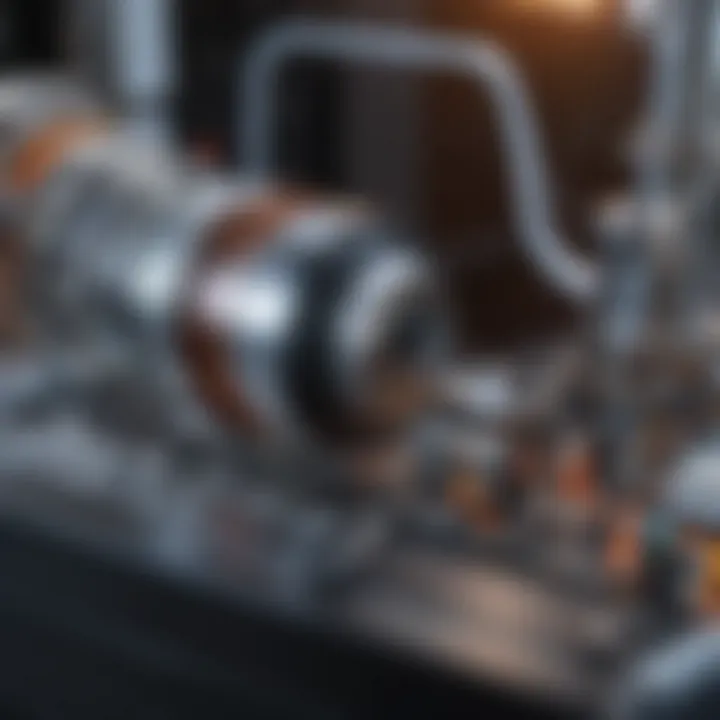
Overview of Protein Structure
Understanding protein structure is essential in the landscape of protein sequencing technology. It aids researchers in deciphering how proteins function, interact, and evolve. Protein structure not only influences application in medical diagnostics and drug development but also provides key insights in environmental science. Grasping the nuances of protein architecture enriches the interpretation of sequencing data and allows for more precise applications in various fields.
Amino Acids and Their Roles
Amino acids serve as the building blocks of proteins. There are 20 unique amino acids that combine in various sequences to form polypeptide chains. Each amino acid has distinct properties that influence how it behaves in a protein. For instance, hydrophobic amino acids tend to avoid water, while hydrophilic ones interact with it. This intrinsic behavior affects the protein’s overall structure, stability, and function.
The sequences of these amino acids dictate the folded shape of a protein, impacting its interactions and activities. Understanding the properties of amino acids is critical in protein design and therapeutic advancements, such as creating targeted drugs that interact effectively with specific proteins in the body.
Levels of Protein Structure
Primary Structure
The primary structure of a protein refers to its linear sequence of amino acids. This aspect is fundamental because it determines all other levels of structure. A small change in sequence can drastically alter a protein’s function.
The primary structure is significant for understanding genetic coding and mutation effects. In this article, the focus on the primary structure allows for insights into disease mechanisms caused by aberrations in amino acid sequences.
Key characteristic: It is straightforward and unambiguous.
Advantage: Provides clear information about specific sequences, vital for targeted research.
Secondary Structure
Secondary structure describes local folded shapes that form within a protein due to hydrogen bonding, primarily in the backbone. The most common types are alpha helices and beta sheets. These structures enhance a protein's stability and flexibility.
The secondary structure enhances understanding of protein functions by organizing motifs that can be common across different proteins.
Key characteristic: It gives a glimpse into the protein's folding patterns.
Advantage: Helps predict the behavior of proteins and their interactions in various conditions.
Tertiary Structure
The tertiary structure encompasses the overall three-dimensional shape of a protein. It arises from interactions between R-groups of the amino acids, which include ionic bonds, hydrogen bonds, hydrophobic interactions, and disulfide bridges. This level is crucial as it dictates how proteins interact with each other and with ligands.
Tertiary structure is often considered the functional structure of the protein, as most biological activities occur at this level. Understanding this helps in designing drugs that target specific protein functions or mutations.
Key characteristic: The unique 3D arrangement.
Advantage: Enables precise understanding of protein mechanisms, essential for biotechnology applications.
Quaternary Structure
Quaternary structure refers to the assembly of multiple polypeptide chains into a single functional protein complex. Many proteins need this arrangement to be functional. Hemoglobin serves as a classic example, where its ability to transport oxygen relies upon its quaternary structure.
Understanding the quaternary structure is vital in elucidating complex protein interactions that occur in cellular environments.
Key characteristic: Multiple subunits working seamlessly together.
Advantage: Essential for understanding cooperative binding and regulatory mechanisms in proteins.
By mastering the various levels of protein structure, researchers can unlock vast potential within the field of protein sequencing. This knowledge informs numerous applications, from diagnostics to therapeutic interventions.
Key Technologies in Protein Sequencing
Understanding the key technologies in protein sequencing is vital for grasping how this field has evolved and its broader implications. Modern sequencing methods have revolutionized our ability to analyze and interpret protein structures and functions, providing insights into biological processes and disease mechanisms.
Mass Spectrometry
Mass spectrometry (MS) is one of the cornerstone technologies in the field of protein sequencing. It operates by measuring the mass-to-charge ratio of ions, allowing for detailed analysis of protein molecules. MS offers high sensitivity and specificity when detecting proteins, making it invaluable in proteomics. The technology provides both qualitative and quantitative data, enabling researchers to identify proteins and determine their abundance in complex mixtures.
Some benefits of mass spectrometry include:
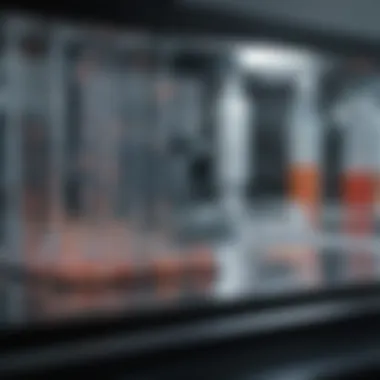
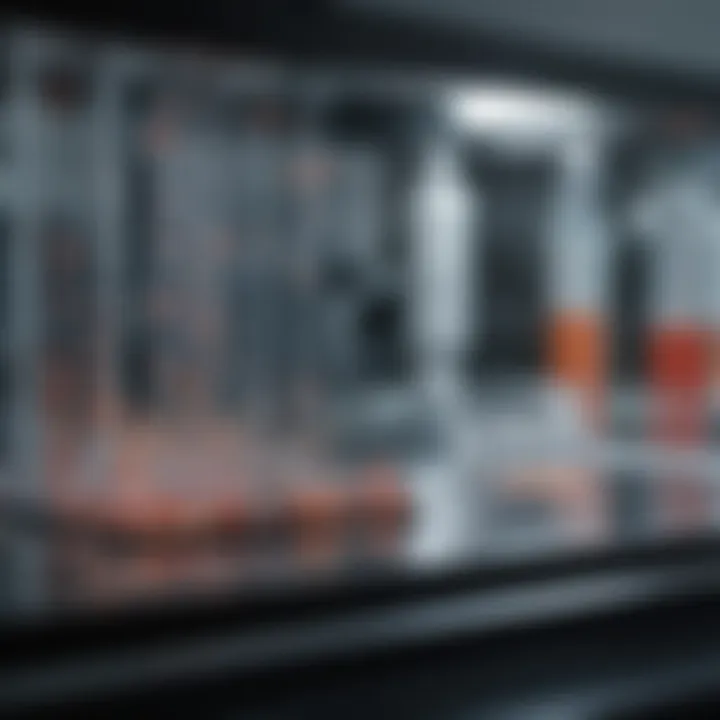
- Speed of Analysis: It can quickly analyze samples, delivering results in a shorter time frame compared to traditional methods.
- Versatility: It is suitable for a wide range of samples, including those from various biological sources.
- High Resolution: The ability to separate molecules based on their mass enhances accuracy.
Despite its advantages, mass spectrometry also faces challenges. Sample preparation can be time-consuming and requires technical expertise. Additionally, the interpretation of mass spectra can be complex. It demands a thorough understanding of biochemistry and analytical techniques to make accurate conclusions.
Next-Generation Sequencing
Next-Generation Sequencing (NGS) has transformed protein sequencing by allowing for massive parallel sequencing of DNA, which can then be translated into protein sequences. This method has significantly lowered the cost of sequencing and increased throughput. NGS technologies, such as Illumina and Ion Torrent, are widely used for their speed and accuracy.
Key aspects of NGS include:
- High Throughput: NGS can process millions of sequences simultaneously, vastly improving the efficiency of sequencing.
- Comprehensive Coverage: It allows for sequencing entire genomes or specific regions, leading to a deeper understanding of protein diversity.
- Rapid Evolution: Ongoing advancements continue to enhance the capability and efficiency of NGS platforms.
However, NGS does come with challenges. The sheer volume of data it generates can overwhelm researchers. Effective bioinformatics tools are necessary to analyze and interpret this data. Additionally, quality control during sequencing must be maintained to ensure reliable outcomes.
Edman Degradation
Edman degradation is a well-established method for sequencing proteins, particularly useful when analyzing shorter peptides. This technique sequentially removes amino acids from the N-terminus of a peptide, allowing for the identification of each amino acid in order. While it has been largely supplanted by newer technologies, Edman degradation still holds significance in specific contexts.
Characteristics of Edman degradation include:
- Direct Sequencing: This method provides a direct approach to determining the amino acid sequence, which can be beneficial for smaller proteins.
- Historical Significance: It laid the groundwork for the development of more advanced technologies, highlighting its importance in the evolution of protein sequencing.
- Simplicity: Edman degradation is generally easier to execute and requires less sophisticated equipment compared to mass spectrometry or NGS.
Nonetheless, Edman degradation also has limitations. It is not suitable for large proteins due to incomplete sequences. Moreover, the method can be slower when analyzing extensive datasets. Its use today is often in tandem with other technologies to confirm results or gather specific information on peptide fragments.
Applications of Protein Sequencing
The application of protein sequencing technology has become increasingly significant in various fields, primarily due to its potential to deepen our understanding of biological systems. Protein sequencing plays a vital role in the study of proteomics, which involves the large-scale analysis of proteins, their sequences, and functional roles. As research progresses, the advantages of protein sequencing become more evident, showcasing its importance in areas such as medical diagnostics, drug development, and environmental monitoring.
Medical Diagnostics
In medical diagnostics, protein sequencing offers a powerful tool for understanding diseases at the molecular level. By analyzing the protein compositions in body fluids, doctors can gain insights into the presence of specific biomarkers that indicate various conditions. For instance, proteins linked to cancer can be identified through sequencing, allowing for earlier and more accurate diagnoses. This specificity can lead to more tailored treatment plans, improving patient outcomes.
Additionally, protein sequencing facilitates the identification of pathogens. In cases of infectious diseases, isolating and sequencing the proteins of the infectious agents helps in understanding their structure and how they interact with human proteins. Targeted therapies can then be developed, making the treatment process more efficient. As researchers continue to explore this field, the potential for enhanced diagnostic tools only grows.
Drug Development
Protein sequencing is instrumental in the drug development process. By sequencing proteins related to specific diseases, researchers can identify target proteins for new therapeutic agents. Understanding the structure and function of these proteins allows for rational drug design, which increases the likelihood of developing effective treatments.
Moreover, drug efficacy and safety profiling depend on understanding protein interactions. Protein sequencing can identify potential off-target effects, which can reduce adverse reactions in patients. This precision in drug development not only leads to better treatment options but also potentially reduces the cost and time involved in bringing a drug to market. As technological advancements continue, the integration of protein sequencing in drug development will likely expand, fostering innovation in therapeutic applications.
Environmental Monitoring
The utility of protein sequencing extends beyond healthcare; it also plays a crucial role in environmental monitoring. Protein analysis helps in assessing the health of ecosystems by providing data on biodiversity. Sequencing proteins from various organisms can reveal the presence of specific species, including those that might be endangered or invasive.
Environmental scientists utilize protein data to understand how biodiversity affects ecosystem stability and resilience. For example, identifying specific protein markers in organisms can serve as indicators of environmental stress caused by pollutants. Utilizing these indicators aids in tracking ecological changes, thus informing conservation efforts.
"The future of protein sequencing technology holds great promise, shaping fields from medicine to environmental science."
The integration of protein sequencing across these fields encourages a multidisciplinary approach that underscores its relevance in addressing some of the most pressing challenges today. The collective growth in understanding gained from these applications will be central to shaping future research and innovation.
Ethical Considerations in Protein Sequencing
The field of protein sequencing technology holds significant promise across various industries, including medicine and biotechnology. However, this progress brings forth a range of ethical considerations that merit careful examination. As researchers and professionals venture deeper into the complexities of proteomics, the treatment of genetic and protein data raises important questions about privacy and the ethical use of such information.
Privacy Concerns
In light of the technological advancements in protein sequencing, privacy emerges as a paramount issue. The ability to decipher proteins involved in various biological processes can reveal intimate details about an individual’s genetic makeup. This capability invites scrutiny regarding who has access to this sensitive information. Concerns regarding unauthorized sharing or misuse of genetic data are prevalent, particularly in commercial settings where profit motives might conflict with individual privacy rights.
Individuals often assume that their genetic and protein information is secure, but this is not always the case. Data breaches or lax security protocols can expose private information, resulting in potential consequences for the individuals concerned. The risk of discrimination based on genetic data is also a pressing issue, especially in sectors such as health insurance and employment. Therefore, developing stringent regulatory frameworks becomes essential to protect individuals from potential harm.
Implications of Genetic Data Usage
The implications of using genetic data are multifaceted and complex. On one hand, leveraging protein sequencing for medical advancements can lead to groundbreaking personalized treatments and enhanced disease prevention strategies. On the other hand, the ethical ramifications of how this data is utilized cannot be overlooked.
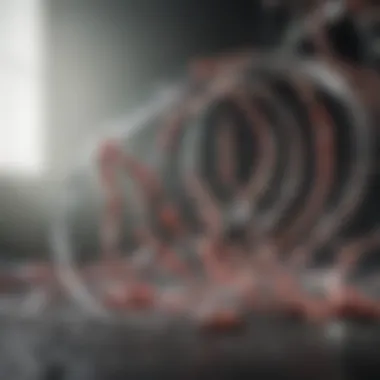
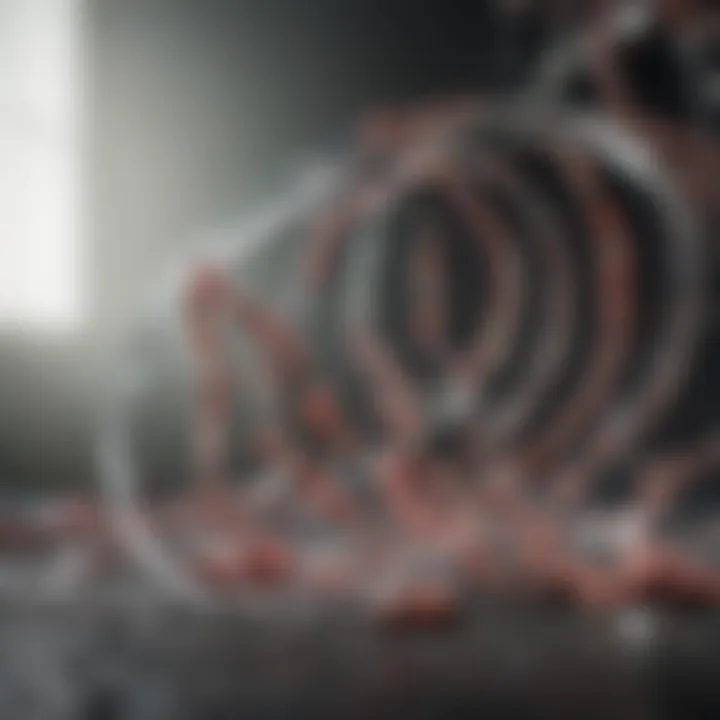
Key considerations include:
- Consent and Transparency: Researchers must ensure informed consent is obtained from participants. Individuals should be fully aware of how their data will be used and the potential risks involved.
- Ownership of Data: Questions arise about who owns the genetic data collected through protein sequencing. Is it the individual, the researcher, or the institution? This ambiguity needs clarity to prevent conflicts and misuse.
- Potential for Discrimination: There is a significant concern regarding genetic data impacting social or economic opportunities. For instance, if employers gain access to protein sequencing data, they may unjustly discriminate against individuals predisposed to certain health conditions.
"While the potential of protein sequencing is profound, it is equally vital to navigate the ethical landscape cautiously to avoid adverse consequences for individuals and society."
Challenges in Protein Sequencing Technology
The field of protein sequencing technology has witnessed remarkable advancements over the past few decades. However, numerous challenges still hinder the full realization of its potential. Understanding these challenges is crucial because they directly impact the accuracy, speed, and applicability of sequencing methods in both research and clinical settings.
Technical Limitations
Protein sequencing technologies, despite their improvements, are subject to various technical limitations. One major limitation is the inherent complexity of protein structures and functions. Proteins are not only diverse in their sequences but also undergo various modifications post-translation, making them difficult to characterize fully. Techniques like mass spectrometry, while advanced, often struggle with the detection of low-abundance proteins or those with complex structures.
Moreover, resolution and sensitivity vary among technologies. Some methods may not accurately resolve the absolute abundance of proteins, which is critical in quantitative proteomics.
Despite advances, the technical challenges in protein sequencing remain significant barriers to routine use in some clinical applications.
Integrating multiple sequencing methods is often needed to increase reliability, which leads to increased costs and complexity in workflows. The need for specialized equipment and skilled personnel also contributes to accessibility issues, particularly in resource-limited settings.
Data Interpretation Issues
The challenge of interpreting sequencing data is another significant hurdle. Protein sequencing generates vast amounts of data, making management and analysis daunting. This deluge of information requires sophisticated software tools and bioinformatics approaches for accurate interpretation. However, discrepancies can arise, leading to challenges in validation. For example, spectral data collected from mass spectrometry must be compared against reference libraries, and mismatches can yield incorrect conclusions about protein identities and quantities.
Additionally, different sequencing technologies have their own unique data formats and requirements. This inconsistency can complicate data integration across platforms. Researchers must ensure rigorous data handling protocols to avoid misinterpretations that can lead to erroneous scientific conclusions or even impact clinical decisions.
In summary, while protein sequencing technology is advancing, these challenges underscore the need for ongoing research and development in the field. Addressing technical limitations and improving data interpretation methods will be essential to maximize the technology's potential in real-world applications.
Future Perspectives
The advancements in protein sequencing technology will likely redefine our understanding of biology and enhance the efficiency of various sectors. This evolving field holds potential for not only improving current methodologies but also introducing novel applications that can profoundly affect medicine, environmental science, and biotechnology. Addressing future perspectives is essential to comprehend how these advancements can catalyze innovation and shift paradigms in multiple domains.
Advancements on the Horizon
In the near future, we can expect several significant advancements in protein sequencing technologies. One of the most promising areas is the integration of artificial intelligence and machine learning with existing sequencing methods. These technologies will improve data analysis and interpretation, making it faster and more accurate. Enhanced algorithms could facilitate the identification of protein structures and functions with unprecedented precision. For example, AI-driven tools may analyze complex datasets in proteomics, revealing insights that current methods might miss.
Moreover, the development of portable and miniaturized sequencing devices is on the rise. Such devices could allow researchers to conduct protein analysis outside of traditional lab environments, making sequencing more accessible and practical in remote locations. This could transform how environmental monitoring is conducted, aiding in real-time assessments of biomes and ecosystems.
Another potential advancement involves the refinement of single-cell protein sequencing. Refining this technique can yield deeper insights into cellular diversity and the role of proteins in various biological processes. Understanding these dynamics at a single-cell level could fundamentally alter therapeutic strategies, especially in personalized medicine.
Potential Impact on Scientific Research
The future advancements in protein sequencing will significantly influence scientific research across various fields. By improving the accuracy and efficiency of sequencing methodologies, researchers will uncover intricate cellular mechanisms. New findings can shed light on disease pathways that were previously unclear, enhancing the development of targeted therapies.
In drug development, faster and more reliable protein sequencing can accelerate the discovery of new drug candidates. This would lead to more efficient preclinical studies, ultimately reducing time and costs associated with bringing new therapies to market. Researchers can better understand drug interactions at the molecular level, tailoring interventions based on an individual's proteomic profile.
"The continuous evolution of protein sequencing technologies promises to illuminate hidden biological complexities and drive research in ways we are just beginning to understand."
Furthermore, as sequencing becomes more integrated with computational biology, the insights gained from large datasets can fuel innovative hypotheses and experimental designs. Researchers can explore protein functions and interactions on a large scale, fostering collaborations across disciplines.
Finale
The conclusion in this article is vital because it encapsulates the developments and future direction of protein sequencing technology. This field is invaluable not only for its scientific merit but also for the societal implications it carries. By reflecting on key advancements, challenges, and ethical considerations discussed previously, this section serves to reinforce the significance of protein sequencing’s role in modern science.
Key elements include the remarkable progress in methodologies such as mass spectrometry and next-generation sequencing. These advancements have dramatically enhanced our understanding of proteomics, impacting fields like medicine and biotechnology. Researchers are now able to almost instantly analyze complex protein samples, enabling quicker diagnostic capabilities and more effective drug designs.
Additionally, the conclusion highlights the implications of this research for personalized medicine. Tailoring treatment to fit individual genetic profiles can lead to vastly improved patient outcomes. Thus, it is not merely an academic pursuit; it translates into real-world benefits that affect millions.
Summary of Key Points
- Technological Advancements: The introduction of innovative techniques has transformed protein sequencing accuracy and efficiency.
- Real-World Applications: Breakthroughs in drug development and disease diagnostics underscore the practicality of these advancements.
- Ethical Concerns: Privacy and data handling remain critical issues that must be addressed as technology evolves.
- Future Prospects: Continuous advancements promise greater integration of protein sequencing in various scientific disciplines.
The ongoing evolution of protein sequencing technology reshapes our approach to understanding biological processes, ultimately pushing the boundaries of what science can achieve.
Call to Action for Researchers
Researchers are called upon to engage actively with evolving protein sequencing methodologies. This involves not just utilizing existing technologies but also innovating and refining them. Collaboration with interdisciplinary teams can lead to enhanced applications and address current technical limitations.
Moreover, staying informed about ethical considerations is essential. Researchers should contribute to discussions on best practices for data privacy and responsible usage of genetic data.