Exploring Biopolymers: Structure, Function and Uses
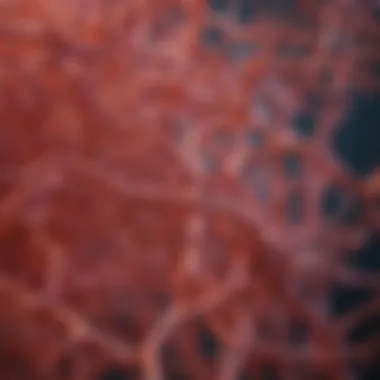
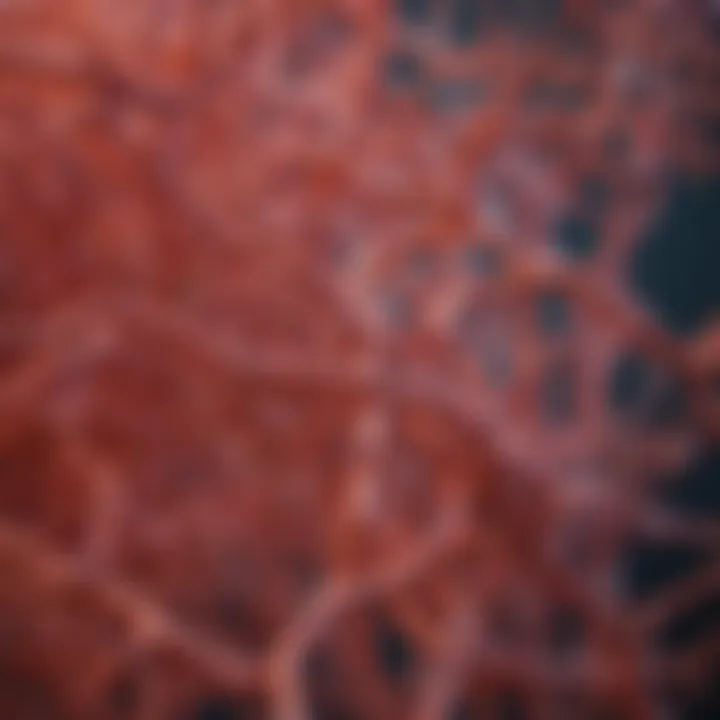
Intro
Biopolymers have emerged as a cornerstone of biological systems, wielding significant sway over myriad processes that define life. Whether it's the robust structure of cellulose found in plant cell walls or the intricate sequence of amino acids in proteins, these naturally occurring macromolecules are instrumental to sustaining ecosystems and facilitating countless biological functions. But what exactly sets biopolymers apart, and why are they gaining so much attention in contemporary research and application realms?
This exploration divulges into the captivating intricacies of biopolymers, from their diverse structures to their functional roles in living organisms. As we peel back the layers of these fascinating compounds, we will delve into their pressing significance in materials science, medicine, and even environmental sustainability. Through a detailed lens, we aim to unravel the various types, synthesis pathways, and the cutting-edge advancements in bioengineering, showcasing how biopolymers are poised to reshape industries and contribute to sustainable development initiatives.
As we progress, the information will not just skim the surface; instead, we will engage with detailed examples and case studies to elucidate the transformative potential of biopolymers. In doing so, we hope to offer a comprehensive understanding that satisfies the inquisitive minds of students, researchers, educators, and professionals alike.
Key Points of Discussion
- The diverse structures of biopolymers and their biological implications.
- Understanding the synthesis processes that govern biopolymer formation.
- Applications across various fields, from technological innovations to environmental sustainability.
- Emerging trends and future possibilities in biopolymer research.
By the end of this article, readers should not only grasp the fundamental aspects of biopolymers but also appreciate their overarching relevance in driving forward scientific discovery and societal advancements.
Understanding Biopolymers
Biopolymers are a fundamental aspect of life and have gained attention due to their multifaceted roles in nature as well as their potential applications in technology and medicine. Their importance is not just confined to biology; it extends into various scientific domains, influencing materials science, environmental sustainability, and health sciences. A strong foundation in understanding biopolymers can unlock doors to innovative research and applications.
One significant benefit of studying biopolymers is the insights it offers into natural processes. This understanding can inspire the design of synthetic materials that mimic natural systems—often leading to sustainable alternatives to traditional polymers. By comprehending their structures and functions, researchers can make strides in creating materials that not only perform well but also minimize environmental impact.
There are key considerations to keep in mind about biopolymers. First, their diverse structures—ranging from complex carbohydrate chains to intricate proteins—allow them to perform specific functions critical to life. Second, their ability to degrade under natural conditions highlights their potential role in reducing plastic waste when applied in industrial contexts.
"The study of biopolymers bridges the gap between natural science and innovative technology."
Therefore, cultivating a solid understanding of biopolymers sets the stage for effectively utilizing their properties in a variety of applications. Let's dive a little deeper into this fascinating topic.
Defining Biopolymers
Biopolymers can be simply defined as large molecules composed of repeating structural units, known as monomers, that are produced by living organisms. Unlike synthetic polymers, which can often be derived from petroleum, biopolymers derive from natural sources. This includes polysaccharides, proteins, nucleic acids, and lipid-based structures. Each of these components presents a unique architecture that allows them to play specific roles within biological systems.
Primarily, biopolymers serve key functions such as energy storage, structural integrity, and information transfer. For instance, polysaccharides like starch and cellulose are fundamental in energy storage and structural roles respectively, while proteins are instrumental in catalyzing biochemical reactions and providing cellular structure.
Historical Context
Understanding the historical journey of biopolymers reveals how our knowledge has evolved. The exploration of biopolymers can be traced back centuries. Early civilizations utilized natural biopolymers without fully realizing their significance. For example, the use of natural gums in adhesives or the discovery of milk proteins for cheesemaking.
In the late 19th century, pioneering scientists began to dissect the structure of biopolymers, laying the groundwork for modern biochemistry. The identification of DNA's double helix structure in the early 20th century marked a pivotal moment in the understanding of biopolymers, emphasizing their role in hereditary information and biological function. This newly minted knowledge catalyzed further research, leading to the current advances in biotechnology and genetic engineering, where biopolymers are being engineered to solve pressing global challenges.
Today, ongoing research continues to explore how biopolymer applications can be expanded, enhancing both scientific knowledge and practical solutions to modern problems. Thus, our grasp of biopolymers has not only transformed scientific understanding but has also paved the way for innovations that stand to impact various facets of life.
Types of Biopolymers
Understanding the types of biopolymers is crucial for grasping their roles and potential applications across various fields. Biopolymers are diverse molecules that can be categorized mainly into four groups: polysaccharides, proteins, nucleic acids, and lipid-based polymers. Each type holds unique structural features and functional properties that contribute to their significance in biological systems as well as their utility in technology and industry. Recognizing these types allows researchers and practitioners to explore innovative ways to harness their benefits, from developing biodegradable materials to enhancing medical therapies. In this section, we’ll delve into each type and elucidate their characteristics and significance.
Polysaccharides
Structure and Function
Polysaccharides consist of long chains of monosaccharides linked together. This structure provides them with great versatility and stability, allowing them to serve various functions in living organisms. For instance, cellulose, a predominant structural component in plant cell walls, showcases a linear arrangement of glucose units, imparting rigidity and strength to the plant structure.
A unique quirk about polysaccharides lies in their ability to take on multiple forms. They can be linear or branched, which is favorable for storage or structural functionality. In this article, these characteristics underscore not only their importance in nature but also their potential in creating sustainable materials. Given the rising concern over plastic pollution, polysaccharides can offer biodegradable alternatives that break down efficiently, presenting a clear benefit for both ecology and industry.
Examples in Nature
In nature, examples of polysaccharides abound, ranging from chitin in the exoskeleton of insects to starch in cereals. Starch has a remarkable property: it serves as an energy reservation for plants and is easily digestible, making it a popular dietary component globally.
The unique feature of polysaccharides, their diverse functional roles—such as energy storage, structural integrity, and even signaling—illustrates their necessity in both ecological dynamics and human applications. These diverse functionalities present advantages for industries seeking natural resources that not only fulfill specific roles but do so with eco-friendliness in mind.
Proteins
Amino Acid Sequences
Proteins are composed of long chains of amino acids, and the sequence of these amino acids is pivotal to determining the protein's structure and, subsequently, its function. This sequence behaves much like a blueprint, dictating whether a protein will act as an enzyme, structural material, or transporter.
A key characteristic of amino acid sequences is the variety of combinations possible. With 20 different amino acids to work with, the potential for diverse protein functions is enormous. In industry, the ability to design proteins by manipulating these sequences opens avenues for creating tailored enzymes for specific biochemical reactions or therapies designed for targeted diseases.
Enzymatic Roles
Enzymes are a subset of proteins that catalyze biochemical reactions, significantly boosting the rate at which reactions occur. They are essential for numerous metabolic processes and are therefore honed in various biotechnological applications, from developing pharmaceuticals to biofuels.
The unique feature of enzymes is their specificity; each enzyme typically catalyzes a single type of reaction. This specificity can be both a benefit and a drawback; while it allows fine-tuning of processes, any change in environment may inhibit their activity. The advantages of harnessing enzymes for industrial applications cannot be overstated, as they often work under milder conditions than non-biological catalysts, leading to energy and cost savings.
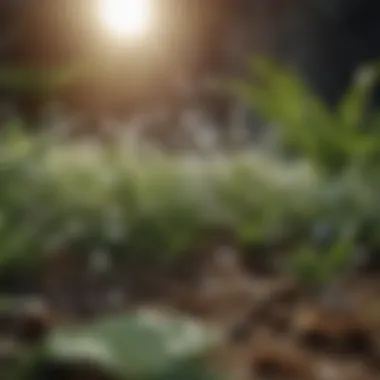
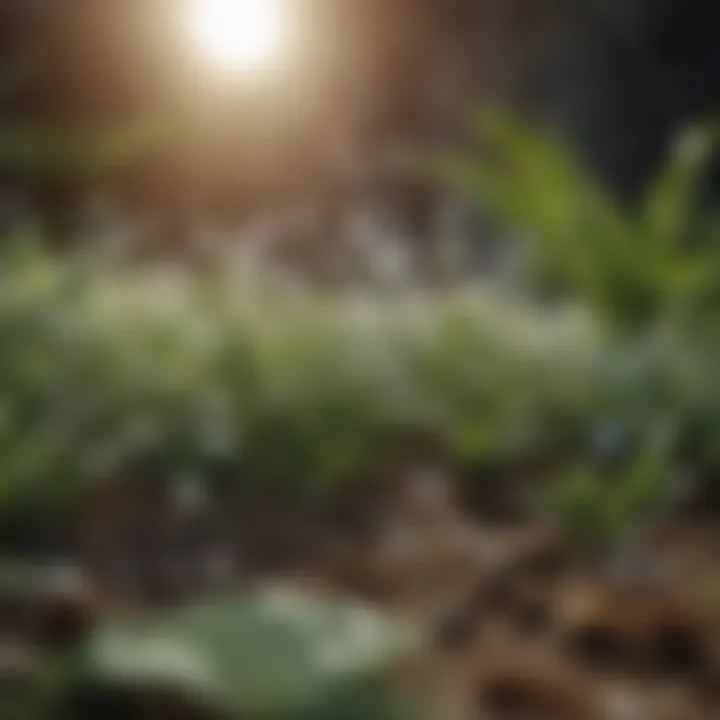
Nucleic Acids
DNA vs RNA
Nucleic acids, namely DNA and RNA, serve as carriers of genetic information. DNA is double-stranded and known for its stability, while RNA is often single-stranded and plays multiple roles in protein synthesis and regulation.
A distinguishing factor of DNA is its structure; specifically, the double helix formation, which protects the genetic information against degradation. RNA, however, is more versatile due to its ability to fold into various shapes, facilitating its functions such as messenger RNA or ribosomal RNA roles in protein synthesis. Understanding this differentiation provides insight into their distinct yet complementary functions in cellular processes.
Genetic Information Storage
The role of nucleic acids in genetic information storage is profound. DNA can be thought of as the blueprint of life, encoding the instructions necessary for the development and functioning of all living organisms.
The ability of DNA to replicate itself is one of its most vital features; this permits inheritance across generations. However, unlike DNA, RNA serves a more transitory function, relaying the messages encoded in DNA to facilitate protein creation. This dual functionality in genetic information processing underlines the importance of both nucleic acids in biological systems, with implications for genetic engineering and biotechnology.
Lipid-Based Polymers
Membranes and Functionality
Lipid-based polymers are integral to building cell membranes and play essential roles in cellular functionality. They contribute to the formation of lipid bilayers, which serve as barriers and facilitators of communication between the cell and its environment. The amphipathic nature of lipids means they possess both hydrophobic and hydrophilic properties, enabling these structures to maintain stability in aqueous surrounding.
Membranes made from lipid-based polymers are not merely protective barriers. They also host a plethora of proteins that facilitate the transport of materials into and out of cells. Understanding these functionalities aids in exploring drug delivery systems and creating bioactive materials that interact harmoniously with biological tissues.
Biological Significance
The biological significance of lipid-based polymers extends beyond mere structural roles. They are involved in signaling pathways and energy storage. For example, phospholipids are key players in intracellular signaling, while triglycerides are stored energy reserves.
Recognizing the unique role of lipid-based polymers proves beneficial not only in the study of biological systems but also in formulating therapies targeting specific pathways or developing bioengineered materials that mimic natural processes. The focus on their biological significance highlights the potential of these polymers in the design of more effective medical treatments.
Understanding these different types of biopolymers provides a foundation for exploring their multifaceted roles in various applications. Their natural origins, accompanied by evolving technology, promise tremendous opportunities for future innovations.
Biopolymer Synthesis
Biopolymer synthesis plays a crucial role in understanding the functionality and applicability of biopolymers. This section dives into the significance of how these macromolecules are formed, whether naturally or synthetically. The processes behind their synthesis not only highlight natural biological mechanisms but also reflect advancements in technology that facilitate innovative uses in various fields.
One of the most compelling aspects of biopolymer synthesis is how it balances nature with technology. By unraveling the ways in which these polymers are produced, researchers can exploit their unique properties for applications ranging from medical uses to environmental solutions.
Natural Synthesis Processes
Enzymatic Pathways
Enzymatic pathways represent a fascinating element of natural synthesis. In this context, enzymes perform as biological catalysts, expediting crucial reactions without being consumed in the process. This specific feature is important because it means that these reactions can occur under mild conditions, such as normal temperatures and pressures, making them both energy-efficient and environmentally friendly.
The key characteristic of enzymatic pathways is their specificity. Each enzyme is tailored to react with particular substrates, leading to the synthesis of distinct biopolymers. This reduces the formation of unwanted by-products, thereby increasing overall yields. This aspect is particularly beneficial since it aligns with the growing demand for cleaner production methodologies in various industries.
However, there are unique features within enzymatic pathways that can create challenges. For example, enzyme stability can vary significantly under different environmental conditions, potentially affecting production efficacy. Additionally, the need for complex regulatory mechanisms to control these pathways can add layers of difficulty to large-scale applications.
Role of Microorganisms
Microorganisms have long been heralded as the unsung heroes in the world of biopolymer synthesis. These tiny life forms play an essential role in producing various biopolymers, including polysaccharides and proteins. The rich diversity found in microbial life means that they can be harnessed to create a wide range of biopolymers that are often hard to synthesize through chemical means.
A major advantage of using microorganisms is their rapid growth and adaptability. They can be cultivated in controlled environments to optimize the yield of a desired biopolymer, making them a popular choice for many research initiatives. Typically, they operate effectively on a variety of substrates, allowing for the use of waste materials, which promotes sustainability.
On the flip side, this method is not without its challenges. The fermentation processes need careful monitoring to avoid contamination and ensure the desired product is synthesized efficiently. Furthermore, scaling up these methods from laboratory to industrial scale can introduce complexity and unpredictability in terms of production consistency.
Synthetic Methods
Chemical Synthesis Techniques
Chemical synthesis techniques have significantly broadened the horizon on how biopolymers can be produced. This approach enables scientists to create polymers that might not necessarily exist in nature, offering endless possibilities for engineering new materials. One great aspect of chemical synthesis is its scalability; chemists can design reactions in ways that are easier to control, leading to high levels of reproducibility.
The flexibility offered by chemical synthesis makes it an appealing choice for creating biopolymers with tailored properties. By manipulating various reaction conditions, such as temperature and pH, researchers can adjust polymer characteristics to suit specific applications. This is often seen in industries where precise specifications are non-negotiable, such as in the pharmaceutical sector.
Despite these advantages, chemical synthesis techniques can come with their own set of hurdles, particularly regarding environmental impact. Many traditional methods use harmful reagents, resulting in toxic by-products that necessitate careful disposal procedures. There's also the need for significant energy input during the reactions, raising questions about the sustainability of such processes.
Biochemical Approaches
Biochemical approaches represent a bridge between natural and synthetic methods for biopolymer production. These techniques often employ engineered microorganisms or enzymes to create biopolymers. By utilizing living systems, researchers aim to replicate the efficiency of natural synthesis while manipulating molecular structures to enhance functionality.
Key characteristics of biochemical approaches include their adaptability and production efficiency. They can often be fine-tuned in real-time, allowing researchers to modify processes based on immediate feedback. This flexibility is paramount in research and industrial settings where innovation is key.
Nonetheless, there are downsides. Biochemical methods can be quite complex, requiring advanced knowledge of biochemistry and molecular biology. This complexity can hinder straightforward scaling up for commercial production. Furthermore, ensuring the stability of genetically modified organisms can pose ethical and safety dilemmas.
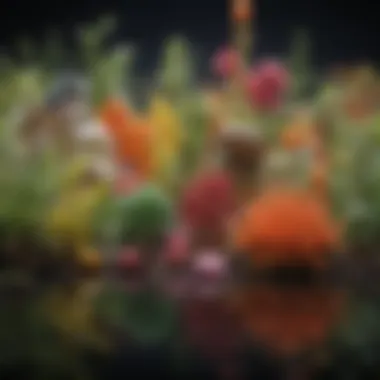
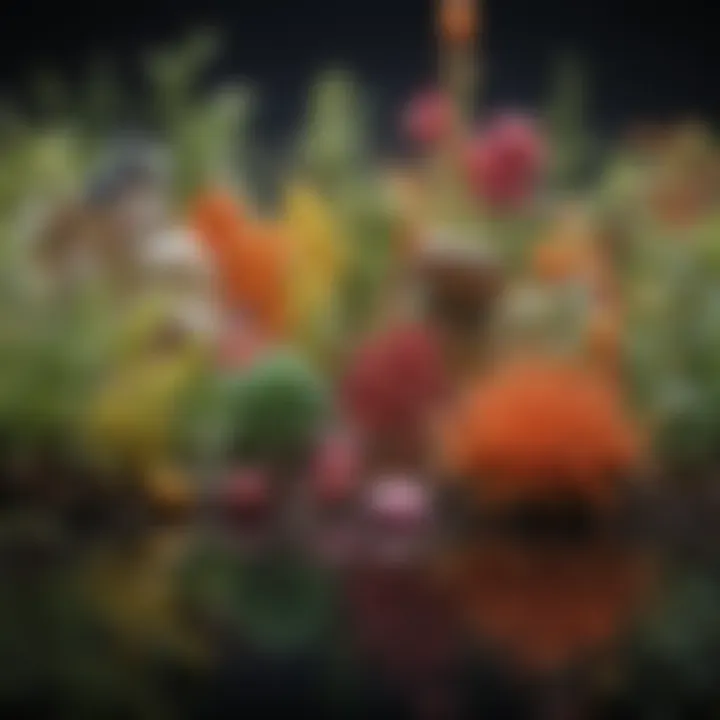
Biopolymer synthesis, whether through natural processes or thoughtful synthetic methods, is an evolving field that holds great potential for the future. Understanding the intricacies of these processes can not only enhance our comprehension of life itself but also pave the way for extraordinary innovations in various industries.
Properties of Biopolymers
Understanding the properties of biopolymers is crucial for harnessing their full potential across various fields such as medicine, environmental science, and materials engineering. These properties dictate how biopolymers interact with other substances and their suitability for specific applications. The blend of physical and chemical properties determines their usefulness, biodegradability, and how effectively they function in real-world settings. Analyzing these characteristics helps researchers and industry professionals develop better biopolymer-based solutions, aligning with both performance criteria and sustainability goals.
Physical Properties
Mechanical Strength
Mechanical strength refers to the ability of a biopolymer to withstand an applied force without breaking. This property is particularly vital, as it contributes to the structural integrity in applications such as drug delivery systems, scaffolding in tissue engineering, and various materials in packaging. One key characteristic is tensile strength, which measures how much force a biopolymer can endure while being stretched.
Many biopolymers, like collagen, are favored for their exceptional tensile strength, making them a popular choice in the development of medical implants. A unique feature of mechanical strength is its ability to be tailored through various processing methods that can alter the polymer's arrangement and interaction with other molecules.
While high mechanical strength offers many advantages, it can also lead to challenges, such as brittleness in certain conditions. Thus, while stronger is often better, striking a balance is essential to ensure functionality without compromise.
Thermal Stability
Thermal stability defines how well a biopolymer can maintain its properties at elevated temperatures. This aspect is significant in many applications where exposure to heat is inevitable. Poly(lactic acid) is one such biopolymer, which is extensively used in packaging due to its good thermal stability. It retains its mechanical properties even at higher temperatures, making it a preferred choice in diverse settings.
One essential characteristic to consider is the glass transition temperature (Tg), which indicates the temperature at which the biopolymer shifts from a hard, glassy state to a softer, more flexible state. A notable feature of thermal stability is its impact on processing conditions during manufacturing; high thermal stability allows for various fabrication techniques without degrading the material.
However, there are also drawbacks. Biopolymers may sometimes require additives to enhance their thermal stability, which could impact natural biodegradability. Finding solutions that bolster thermal resistance while retaining ecological benefits is an ongoing challenge.
Chemical Properties
Reactivity and Degradability
Reactivity and degradability are crucial components when considering biopolymers for specific applications. Biopolymers like chitosan are known for their high reactivity, allowing them to form bonds with numerous chemical agents. This reactivity is pivotal in areas like drug delivery, where the polymer must interact effectively with therapeutic compounds to release them in a controlled manner.
A notable characteristic is their ability to degrade biologically, breaking down into harmless byproducts when exposed to microbial action or moisture. This characteristic is highly beneficial in reducing landfill waste and pollution. One unique aspect of degradable polymers is their capacity to adapt to varying environments; for instance, certain chitosan derivatives can degrade more slowly in acidic conditions, prolonging their activity in specific applications.
On the downside, high reactivity may necessitate additional controls to prevent unwanted reactions outside intended contexts. Therefore, understanding reactivity is key to leveraging biopolymers effectively.
Solubility Characteristics
Solubility characteristics of biopolymers determine their behavior in biological and environmental contexts. For instance, gelatin possesses high solubility in water and can easily form hydrogels, making it highly suitable for applications in food science and drug delivery. One essence of solubility is its influence on the biopolymer's bioavailability, affecting how well it interacts with other substances in applications.
A prominent feature of solubility in biopolymers is the concept of amphiphilicity, where portions of the polymer can interact with both hydrophilic and hydrophobic substances. This dual nature allows for enhanced applications in emulsifiers or drug delivery systems, where working with a range of compounds is essential.
The advantages here are clear; solubility can enhance the efficacy of biopolymers and their rates of absorption in organisms. However, excessive solubility could be a disadvantage, especially in packaging, where a too-soluble material may lead to degradation during storage. Finding the right balance is crucial in determining optimal applications.
Understanding the properties of biopolymers allows for innovative applications that optimize functionality while addressing pressing sustainability concerns.
Ultimately, a deeper dive into the properties of biopolymers sheds light on their complex nature and highlights their importance in diverse fields. From sustaining human health to reducing environmental impact, the characteristics explored here pave the way for advancements in both technology and ecological responsibility.
Applications of Biopolymers
The significance of biopolymers can’t be overstated, as they are increasingly recognized for their diverse applications across various sectors. Their inherent properties such as biodegradability, biocompatibility, and versatility make them invaluable in industries ranging from healthcare to agriculture. This section will shine a light on the ways these natural macromolecules are currently being utilized and emphasize the benefits they bring to our world. The ability of biopolymers to mimic natural biological processes gives them a strong edge, especially in medical applications and sustainable practices.
Medical Use
Drug Delivery Systems
The realm of drug delivery is undergoing a transformation with the integration of biopolymers. These naturally derived substances often serve as carriers, facilitating the targeted release of medications. A key characteristic of drug delivery systems utilizing biopolymers is their ability to encapsulate drugs effectively while maintaining stability. Many pharmacists choose biopolymers for their inherent biocompatibility, ensuring that there are minimal adverse reactions with human tissues.
One unique feature of these systems is controlled release capability, which allows medications to be delivered over a sustained period. This significantly enhances the therapeutic efficacy and patient compliance. However, challenges remain, like the possibility of degradation before reaching the target site, which raises questions about optimization and reliability in various medical contexts.
Tissue Engineering
In tissue engineering, biopolymers play a pivotal role in creating scaffolds that support cell growth and tissue regeneration. Their porous nature is a fundamental quality that attracts researchers and practitioners alike, as it allows for the proper diffusion of nutrients and hormones. This aspect contributes immensely to their popularity in regenerative medicine.
Biopolymers can be tailored to mimic the structural and mechanical properties of natural tissues. Their unique biodegradability ensures that as new tissue forms, the scaffold gradually dissolves, leaving behind the newly formed structure. That said, while flexibility and adaptability are advantages, there is the concern of variable degradation rates that can affect the outcome of the tissue repair process.
Environmental Applications
Biodegradable Plastics
Biodegradable plastics represent one of the most significant breakthroughs in addressing the global plastic pollution crisis. The emergence of biopolymers has heralded a shift toward materials that can break down naturally, unlike conventional plastics that linger in the environment for centuries. Their renewable nature stands out, making them a preferred choice for eco-conscious manufacturers.
One distinctive feature of biodegradable plastics is their source material, often derived from starch, cellulose, or other natural substances. This characteristic not only aids in soil enrichment upon degradation but also significantly cuts down on the reliance on petroleum-based products. Yet, potential drawbacks loom, particularly regarding their production costs and performance characteristics compared to traditional plastics. These dilemmas warrant careful consideration as industries navigaate their implementation.
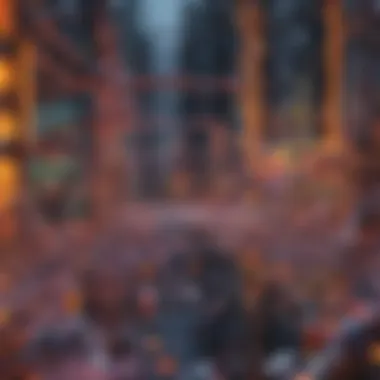
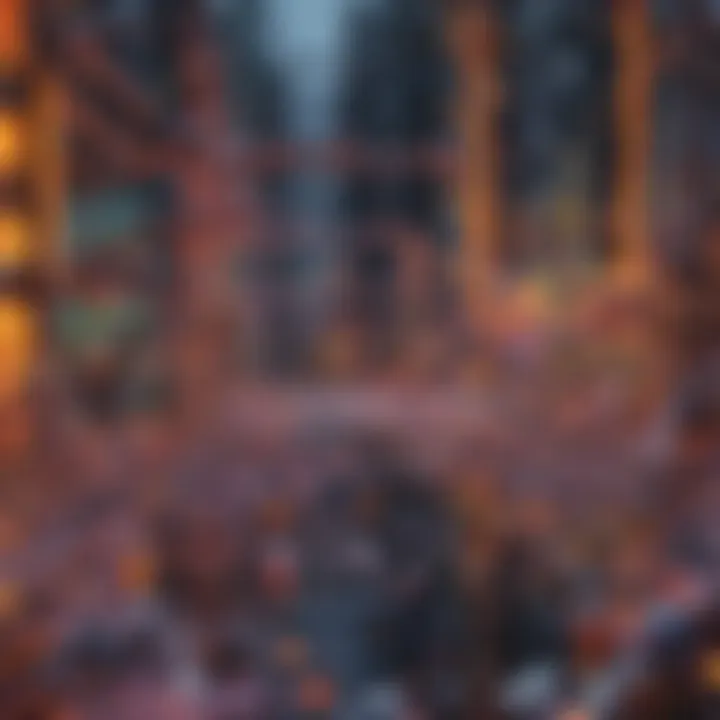
Waste Management
In waste management, biopolymers offer innovative solutions that align with sustainability goals. Their natural decomposition process enhances waste treatment methods, providing a practical avenue for reducing landfill overflow. Biopolymers can be integrated into systems that compost organic waste effectively, thus facilitating the return of nutrients to the soil.
A striking feature of biopolymers in waste management is their ability to absorb contaminants, which could lead to cleaner waste streams. This is a notable benefit for communities striving to minimize environmental harm. However, the efficiency of biopolymer-based waste solutions can be inconsistent, raising the question of their scalability and long-term cost-effectiveness in waste handling operations.
Food Industry
Food Preservation Techniques
Food preservation has seen significant advancements through the application of biopolymers. Many food scientists promote the use of natural biopolymers as effective preservatives, primarily due to their antimicrobial properties. This method is gaining traction due to its potential to substitute synthetic preservatives, aligning better with consumer preferences for natural ingredients.
What sets these techniques apart is their ability to form edible films around food products, enhancing shelf life by reducing oxygen permeability. While biopolymers show remarkable promise, there are concerns about sensory qualities in food products, such as texture and taste, that can be impacted by film application.
Nutritional Value Enhancement
Biopolymers also play a role in enhancing the nutritional value of food. They can act as carriers for vitamins, minerals, and bioactive compounds, improving their availability to consumers. A notable characteristic of this enhancement is the fortification of everyday items like yogurt and beverages with natural biopolymer formulations.
This exceptional feature supports health benefits and adds value to food processes. Nonetheless, it is imperative to note the balance between fortification and potential nutrient interactions, which need careful assessment to maximize the efficiency of these nutritional enhancements.
Biopolymers in Agriculture
Soil Structure Improvement
Biopolymers are gaining notoriety in agriculture for their role in enhancing soil structure. They improve the soil's water retention capacity, crucial in regions prone to drought. This aspect is particularly beneficial for farmers seeking sustainable practices that boost productivity without depleting resources.
An intriguing feature of biopolymer effects in soil is their ability to form aggregates, promoting a healthier ecosystem for plant roots. However, concerns arise about the long-term effects and the costs associated with their application, highlighting the need for ongoing research on optimal methodologies.
Controlled Release Fertilizers
Controlled release fertilizers, crafted with biopolymers, represent an innovative approach to fertilization. They are designed to release nutrients slowly over time, thereby improving nutrient uptake by plants while minimizing losses to leaching. A primary characteristic of these biopolymers is efficiency, offering farmers a way to reduce the frequency of application.
This innovative design leads to sustained nutrient availability, which can enhance crop yields. The downside, however, is that formulators must ensure the fertilizers remain effective under various environmental conditions, a challenge that needs to be managed thoughtfully to ensure viability in diverse agricultural practices.
Future Directions in Biopolymer Research
The realm of biopolymer research is ever-evolving, capturing the attention of scientists, industry leaders, and environmentalists alike. As we forge ahead into a more sustainable future, advancements in biopolymer technologies become increasingly important. Not only do they offer innovative solutions in various sectors like medicine, agriculture, and environmental management, but they also present unique opportunities for harnessing the natural capabilities of these materials. This section will delve into innovative manufacturing techniques, the nuanced art of biopolymer engineering and design, and the critical sustainability considerations that accompany these advancements.
Innovative Manufacturing Techniques
With the push for more sustainable practices and efficient production methods, innovative manufacturing techniques are at the forefront of biopolymer research. Techniques like 3D bioprinting, for instance, have revolutionized how biopolymers are utilized, allowing for precise control over material properties and structures. This not only enhances performance but also minimizes waste during production. Techniques that incorporate both biological and synthetic elements allow for greater flexibility in material properties, leading to customizable solutions tailored for specific uses. As a result, researchers benefit from optimizing the morphology and distribution of biopolymers, aligning their characteristics more closely with their intended applications.
Biopolymer Engineering and Design
Tailored Properties
Developing biopolymers with tailored properties opens a treasure trove of possibilities. This aspect of engineering allows for a customization that suits particular applications, from medical devices to packaging materials. The key characteristic of tailored properties lies in their adaptability; they can be engineered to have specific mechanical, thermal, or chemical profiles, depending on the requirements. This significance makes them a popular choice in various industries, offering a competitive edge by integrating unique functionalities that standard materials might lack. However, the challenge remains in balancing these tailored properties with cost-effectiveness and regulatory compliance, requiring meticulous consideration during the design phase.
Targeted Applications
Within the scope of biopolymer engineering, targeted applications further refine how these materials meet industry needs. This specificity is what makes these polymers highly effective; for example, polymers engineered for drug delivery can have surface modifications that enhance their interaction with biological systems. The capacity for precise optimization is not just beneficial but crucial as it paves the way for advancements in personalized medicine and environmental sustainability. On the flip side, there can be drawbacks, such as the extra time and resources required for research and development to achieve these targets.
Sustainability Considerations
Life Cycle Assessments
Life Cycle Assessments (LCA) are invaluable when evaluating the sustainability of biopolymers. They provide a comprehensive view that encompasses the entire lifecycle of a product—from raw material extraction to production, usage, and eventual disposal. This holistic approach is crucial for identifying the environmental hotspots during a product's lifecycle, enabling researchers to develop strategies for minimizing adverse impacts. Employing LCA in biopolymer research emphasizes the importance of sustainability, helping to ensure that innovations are not only effective but also environmentally sound. The challenge, however, lies in the complexity of environmental impacts and the difficulty in obtaining accurate data for every aspect of the lifecycle.
Reducing Environmental Impact
Reducing environmental impact is not merely a trend; it is a necessity in the face of climate change and ecological degradation. Identifying avenues to minimize waste, energy usage, and greenhouse gas emissions during biopolymer production contributes significantly to sustainability. Techniques like using renewable resources or biodegradable content help in creating a positive feedback loop where the utilization of biopolymers leads to less environmental burden. Their unique feature, however, is that while they provide superior degradability compared to traditional plastics, they must be designed carefully to maintain functionality. The challenge is two-fold: achieving performance without sacrificing the planet, and ensuring that products made from biopolymers are indeed disposed of responsibly to complete the cycle of sustainability.
The End
The exploration of biopolymers is pivotal in understanding their vast potential and benefits across various fields. This article covered not just the definitions and historical contexts of these remarkable macromolecules but also heralded their application in medicine, environmental sustainability, and agriculture. Highlighting the intricate structures and the unique functions of polysaccharides, proteins, nucleic acids, and lipid-based polymers offers insight into why they are essential components of life.
Recap of Key Insights
Each type of biopolymer brings its own set of characteristics that influence its role in biological processes and industrial applications.
- Polysaccharides serve as energy storage and structural elements in plants, playing vital roles in cell walls and energy metabolism.
- Proteins are perhaps the most versatile, with roles ranging from enzymatic activity to structural support in tissues. It's hard to overstate their importance in the development of life forms.
- Nucleic acids, specifically DNA and RNA, facilitate the intricate dance of genetic information, which is foundational to cellular function and diversity.
- Lipid-based polymers not only maintain cellular integrity through membranes but also contribute to energy storage and signaling pathways.
In terms of their synthesis methods, both natural processes, such as enzymatic pathways, and synthetic techniques play significant roles in producing these biopolymers. Innovations in production methods are something researchers continuously explore, enabling more efficient and environmentally friendly manufacturing techniques.
The Future of Biopolymers
As we move forward, biopolymers present untold possibilities. With growing momentum in sustainability, the future of biopolymers appears bright. Here are a few key points to consider:
- Innovative manufacturing approaches are emerging, incorporating biotechnological advances to create biopolymers with tailored properties that meet specific needs in health, industry, and environmental conservation.
- Biopolymer engineering opens avenues for developing materials that not only perform under strain but do so in a biodegradable manner, addressing issues of pollution and waste management.
- Sustainability remains at the forefront of research and application, with life cycle assessments becoming a standard in evaluating the environmental impact of new materials. The goal is to substantially limit harmful effects on ecosystems while promoting natural degradation.