Chemical Chromatography: Principles and Applications
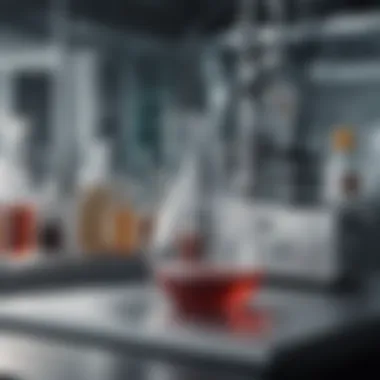
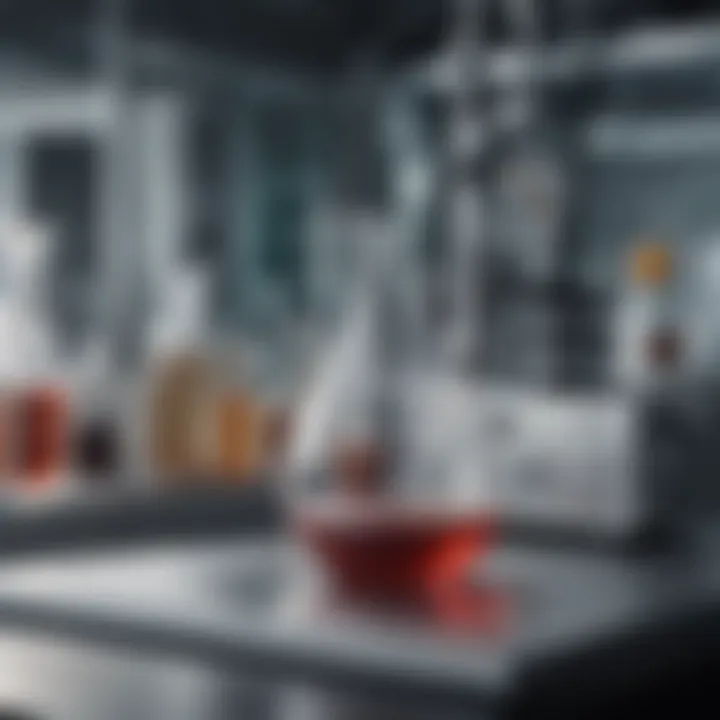
Intro
Chemical chromatography is a pivotal analytical technique that plays a fundamental role in various fields, including chemistry, biochemistry, and environmental science. Its core function is the separation of different components within a mixture, facilitating a clearer understanding of complex chemical interactions and compositions. By isolating individual compounds, researchers can analyze properties, concentrations, and behaviors that would otherwise remain obscured in a complex mixture.
The significance of chromatography extends beyond basic lab applications. It finds usage in pharmaceutical development, forensic science, environmental monitoring, and food safety. As industries evolve, so too does the need for more sophisticated and efficient chromatographic techniques.
Chromatography's applications are underpinned by its principles, which include partitioning between stationary and mobile phases, influencing how substances are separated based on their chemical and physical properties. Understanding these concepts equips researchers and professionals with tools to explore diverse analytical challenges and phenomena in their respective domains.
In this article, we will explore the underlying principles, various methodologies, and practical applications of chemical chromatography. We will consider how modern advancements are shaping the technique's future, ensuring its relevance in contemporary research and industry.
Research Overview
Summary of key findings
Chemical chromatography aids in the precise separation and analysis of mixture components. Key findings in recent studies show an increase in efficiency and sensitivity of chromatographic methods due to new technologies. Advancements such as high-performance liquid chromatography (HPLC) and gas chromatography-mass spectrometry (GC-MS) illustrate how instruments have evolved over time to enhance analytical capabilities.
Diverse methodologies, including liquid chromatography and gas chromatography, provide robust frameworks suitable for different types of analyses. Applications can range from determining alcohol levels in beverages to identifying pollutants in water samples.
Importance of the research in its respective field
The research in chemical chromatography is central to progress in many scientific fields. In pharmaceuticals, for example, it is essential for the quality control of drugs, ensuring that compositions comply with regulatory standards. In environmental science, chromatography helps in monitoring pollutants and assessing environmental health.
As regulatory frameworks tighten and demand for accuracy increases, the importance of this analytical method cannot be overstated. Understanding chromatography and its applications fosters a data-driven approach to solving real-world challenges.
Methodology
Description of the analytical methods used
Different chromatographic techniques have specific methodologies that define how separation occurs. In liquid chromatography, a liquid mobile phase travels through a stationary phase packed in a column. The interactions between the analyte and the stationary phase dictate the retention time, which is key for separation.
Gas chromatography, on the other hand, uses a gaseous mobile phase. Samples are vaporized and carried through a column where separation occurs based on volatility and partitioning in stationary phases. These methodologies enable precise analysis tailored to the properties of the substances being examined.
Sampling criteria and data collection techniques
Successful chromatography hinges on proper sampling and data collection. Sample integrity is crucial. Thus, careful selection of sampling criteria helps mitigate errors caused by contamination or degradation.
Data collection techniques vary between chromatographic methods. For instance, HPLC often uses UV detectors to analyze the separated components as they elute from the column. This allows for real-time monitoring of the separation process. Data retrieved can be quantified using advanced software that matches retention times to known standards, ensuring accuracy in results.
Prolusion to Chemical Chromatography
Chemical chromatography serves as a cornerstone in analytical chemistry, playing a vital role in the separation and analysis of complex mixtures. Understanding the fundamentals of this technique is essential for students, researchers, and professionals alike. Chemical chromatography enables accurate identification and quantification of components within a mixture, which is invaluable across various scientific fields, including pharmaceuticals, environmental science, and food safety.
The importance of studying chemical chromatography stems from its wide-ranging applications and its ability to produce reliable data. It is used in numerous industries for quality control, product development, and forensic analysis. Furthermore, this technique contributes significantly to the development of new drugs, allowing for the effective formulation and testing of therapeutic agents.
As we delve deeper into the nuances of chemical chromatography, we will examine its foundational principles, different methodologies, and their practical applications. It is also crucial to consider the historical context and advancements that have shaped modern chromatography practices. Through this exploration, the relevance and significance of chromatography in contemporary research and industry will become clear.
Definition and Overview
Chemical chromatography is an analytical technique that separates components of a mixture based on differential interactions with stationary and mobile phases. The basic premise involves passing a mixture through a medium that facilitates separation, enabling components to elute at various rates, which can then be analyzed separately. In general, the stationary phase remains fixed, while the mobile phase traverses through it, carrying the components of the mixture along.
Key features of chemical chromatography include its versatility, precision, and the capacity to handle diverse sample types. There are several types of chromatography, including gas chromatography, liquid chromatography, and thin-layer chromatography, each suited for different applications and types of samples. As a fundamental technique in laboratories worldwide, it pervades various analytical tasks and is essential for achieving quality results.
Historical Development
The development of chemical chromatography is marked by significant milestones that have influenced its evolution into the sophisticated technique used today. The term 'chromatography' was first introduced by the Russian scientist Mikhail Tsvet in the early 20th century as he employed the technique for the separation of plant pigments. During this time, Tsvet utilized a stationary phase of calcium carbonate and a liquid mobile phase, demonstrating the fundamental principles of separation.
In the decades that followed, advancements in chromatography were facilitated by the integration of new materials and technologies. The introduction of gas chromatography in the 1950s opened up new avenues for volatile compound analysis, while liquid chromatography techniques underwent considerable enhancements, leading to the development of High-Performance Liquid Chromatography (HPLC) in the 1970s. HPLC allowed for improved resolution, sensitivity, and speed in analyses, making it a standard method in laboratories today.
Recently, the emergence of Ultra-Performance Liquid Chromatography (UPLC) and Supercritical Fluid Chromatography (SFC) has further advanced the field, providing even higher separation efficiency and broader applicability. This historical trajectory underscores the ongoing evolution of chromatography as a response to the growing demands for precision and capability in analytical chemistry.
Basic Principles of Chromatography
Understanding the basic principles of chromatography is essential for grasping how complex mixtures can be analyzed and separated. This section focuses on various mechanisms that underpin chromatography, including the dedicated roles played by adsorption, partitioning, ion exchange, and size exclusion. Each mechanism offers unique characteristics pivotal to its effectiveness in particular applications. This fundamental knowledge provides a solid foundation for those engaged in chemical analysis across different contexts, emphasizing the relevance of chromatography in both academic and industrial settings.
Separation Mechanisms
Adsorption
Adsorption is a widely-used mechanism in chromatography. This method relies on the adherence of molecules to a solid surface. The effectiveness of adsorption varies based on the properties of both the stationary phase and the analytes. One key characteristic of adsorption is its selectivity—certain compounds will bind more tightly than others, allowing for separation. It is a popular choice due to its effectiveness in separating a variety of substances. However, it can be influenced by factors such as temperature and composition of the mobile phase, presenting both advantages and challenges in its application.
Partitioning
Partitioning refers to the distribution of analytes between two immiscible liquid phases, often involving one solid phase and one liquid phase. This method is characterized by its high sensitivity to slight differences in molecular structure, thus enhancing its effectiveness in separating closely related compounds. Partitioning is beneficial particularly in liquid chromatography, where it is vital for separating organic mixtures. Yet, it does have limitations in dealing with highly polar compounds or very large macromolecules, which may not partition effectively.
Ion Exchange
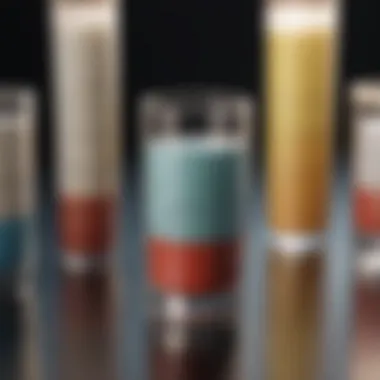
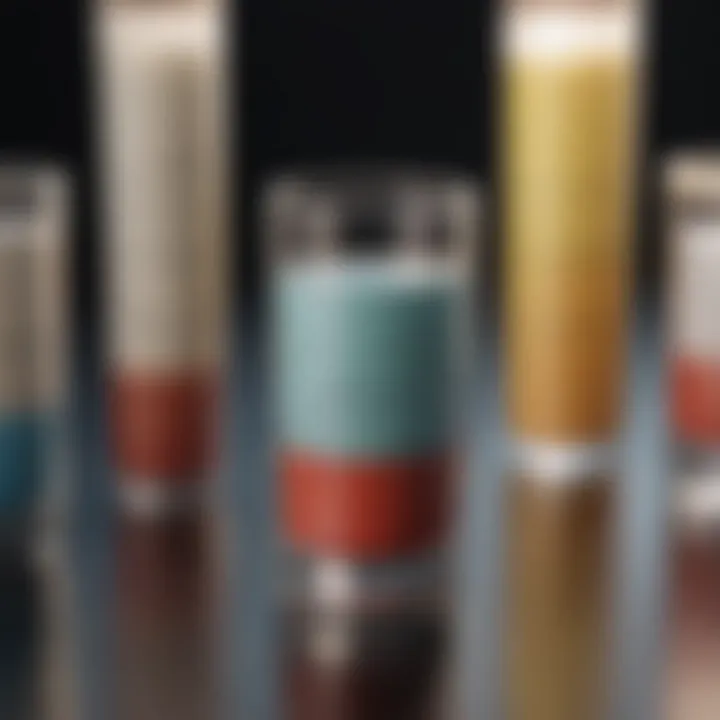
Ion exchange chromatography utilizes the electrostatic interactions between charged analytes and functional groups on a stationary phase. The main feature of ion exchange is its ability to separate charged particles. This makes it an effective technique for analyzing amino acids, peptides, and inorganic ions. Its popularity stems from its ability to handle complex mixtures with high resolution. On the downside, it requires careful control of pH and ionic strength to optimize separations, which can complicate method development.
Size Exclusion
Size exclusion chromatography (SEC) separates molecules based on their size. The process involves passing a solution through a porous medium, where smaller molecules enter the pores and take longer to elute while larger molecules pass rapidly around them. One of the key characteristics of size exclusion is its gentle handling of sensitive biomolecules, making it ideal for proteins and polymers. While SEC does not rely on chemical interactions, it has the disadvantage of lower resolution for closely-sized molecules, which can limit its applications in certain cases.
Retention Factor
The retention factor, often denoted as R_f, is a crucial parameter in chromatography. It measures the relative time a compound spends in a stationary phase compared to a mobile phase. This factor is instrumental in assessing the efficiency of the separation process. The value can help predict the identity of the compound, based on how quickly it moves through the chromatographic system. Understanding and calculating the retention factor is integral for researchers and professionals working with different chromatographic techniques.
Types of Chromatography
Understanding the various types of chromatography is fundamental for appreciating its applications. Each methodology serves specific requirements in separation, making it an essential area of study for students, researchers, and professionals alike. By comprehending these techniques, one can leverage the advantages and limitations inherent to each type for optimal results in respective fields.
Gas Chromatography
Principles and Operation
Gas chromatography uses a gaseous mobile phase to transport samples through a column. The process generally involves injecting a liquid sample into a heated chamber where it vaporizes. The vapor then travels through a column filled with solid or liquid stationary phase, causing different components to separate based on their volatilities and interactions with the stationary phase.
This method is especially useful in analyzing volatile organic compounds. Its main characteristic is speed; gas chromatography offers rapid analysis, making it a beneficial choice in numerous industries, particularly in environmental and petrochemical applications. The ability to achieve high resolution is an unique feature that sets it apart from several other methods, although it requires samples to be volatile, which may limit its applicability.
Applications and Limitations
Gas chromatography finds extensive application in fields ranging from environmental monitoring to food safety. An important characteristic is its capability to separate and analyze complex mixtures with great sensitivity. Additionally, it is an excellent choice for routine analyses due to its reliability and precision. The ability to couple with mass spectrometry further enhances its utility for identifying unknown compounds. However, its limitation lies in the requirement for sample volatility, meaning non-volatile substances cannot be analyzed, restricting its use in certain contexts.
Liquid Chromatography
High-Performance Liquid Chromatography (HPLC)
High-performance liquid chromatography utilizes a liquid mobile phase, providing a good alternative for analyzing non-volatile and thermally unstable compounds. This method achieves high resolution and precision, making it critical in pharmaceutical development and quality control. HPLC's key characteristic is its versatility; it can effectively separate a wide array of chemical substances, including complex mixtures. One unique feature is its ability to analyze smaller sample volumes, which is an advantage in research settings where sample availability may be limited. However, its disadvantage includes longer analysis times compared to gas chromatography, which can be a consideration in high-throughput environments.
Ultra-Performance Liquid Chromatography (UPLC)
Ultra-performance liquid chromatography is a more advanced version of HPLC, using smaller particle sizes for higher efficiency. This results in faster separations and greater resolution. The key characteristic of UPLC is the reduced run times, which improves productivity significantly. UPLC is a beneficial choice in research labs requiring rapid analysis without compromising resolution. The unique feature lies in its ability to handle high pressures, which allows for much shorter columns and faster analytical runs. However, the need for specialized instruments and operational conditions represents a disadvantage, as it can entail higher costs and maintenance demands.
Thin-Layer Chromatography
Principle of Operation
Thin-layer chromatography involves a stationary phase coated on a flat plate or sheet. A sample is applied on one end, and a solvent moves through the stationary phase, carrying the sample components. The key characteristic of this method is simplicity; it requires minimal equipment and is easy to perform. This makes it a beneficial choice for educational purposes and quick qualitative assessments. The unique feature is that results can often be visualized through simple staining techniques, allowing for easy interpretation. However, its disadvantage is lower resolution relative to other chromatographic techniques, limiting its effectiveness in precise separation processes.
Quantitative Analysis
Quantitative analysis in thin-layer chromatography provides a straightforward approach for measuring component concentrations based on the distance traveled by each compound compared to a standard. The key characteristic here is its accessibility; this method is easy for beginners. Its simplicity makes it a beneficial choice for teaching labs or introductory chemistry courses. The unique feature is that it allows for rapid screening without extensive training. On the downside, the methods available for quantification may lead to variability and less accuracy compared to more precise techniques, such as HPLC.
Supercritical Fluid Chromatography
Mechanisms and Benefits
Supercritical fluid chromatography utilizes supercritical fluids, often carbon dioxide, as the mobile phase. This offers distinct advantages, such as lower viscosity and higher diffusivity compared to liquids, facilitating faster separations. The key characteristic of this technique is its efficiency combined with lower solvent consumption. Its beneficial choice primarily lies in its environmental impact since it reduces solvent waste significantly. A unique feature is the flexibility regarding temperature and pressure that can enhance selectivity. However, a disadvantage is the necessity for specialized equipment that can withstand the high pressures required.
Comparison with Liquid Chromatography
The comparison of supercritical fluid chromatography to traditional liquid chromatography reveals several important distinctions. While both methods separate compounds effectively, supercritical methods can achieve similar results with less solvent and reduced analysis time. A significant characteristic is the tunability of the solvent properties in supercritical conditions, which adds versatility. This makes it a beneficial choice for particular applications where solvent properties are crucial. The unique feature of less hazardous waste generation makes it attractive from an environmental standpoint. However, the higher operational costs and complex setup can deter its adoption in routine analysis, putting it at a disadvantage against more established liquid chromatography techniques.
Instrumentation in Chromatography
Instrumentation in chromatography is a critical aspect that affects the accuracy and efficacy of the separation processes. High-quality instrumentation enables researchers to achieve reliable results, making it essential for both analytical and preparative functions. The evolution of technology in this domain has significantly improved the capabilities of chromatographic systems, allowing for more precise analysis and varied application.
Components of Chromatography Systems
Sample Prelude
Sample introduction is the first step in the chromatography process. It involves bringing the sample into the chromatographic system for analysis. This component is crucial as it directly influences the integrity of results. A key characteristic of sample introduction is its variety, which includes methods like manual injection, auto-samplers, and on-line methods.
One beneficial aspect of using automated sample introduction is the reduction of human error. Automation minimizes the risks of sample handling inconsistencies that can lead to inaccurate data. Unique features of this approach include programmability and reproducibility. However, a drawback can be the initial cost and setup complexity. Another consideration is the potential limitation on sample types, as certain methodologies may not suit all sample matrices.
Separation Columns
Separation columns are another fundamental component of chromatography systems. They are responsible for the actual separation of constituents within a mixture based on their physical or chemical properties. The key characteristic of separation columns lies in their packing material and geometry, which determine the efficiency of the separation process.
A popular choice among researchers is the use of high-performance separation columns, particularly in liquid chromatography. These columns offer improved resolution and reduced analysis time. A unique feature is their ability to handle complex sample matrices, which enhances the scope of analyses possible. Yet, there can be disadvantages such as increased back-pressure and the need for specialized handling due to fragility.
Detectors
Detectors play a pivotal role in chromatography by identifying and quantifying the separated components post-separation. The key characteristic of detectors involves their sensitivity and specificity. Various types of detectors exist, such as UV-Vis, fluorescence, and mass spectrometric detectors, each offering advantages depending on the application.
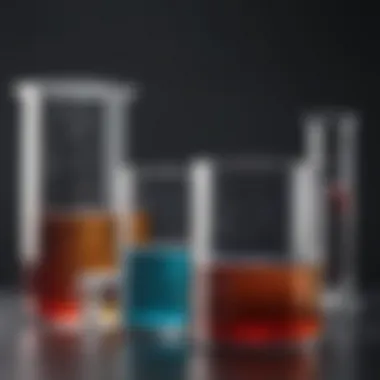
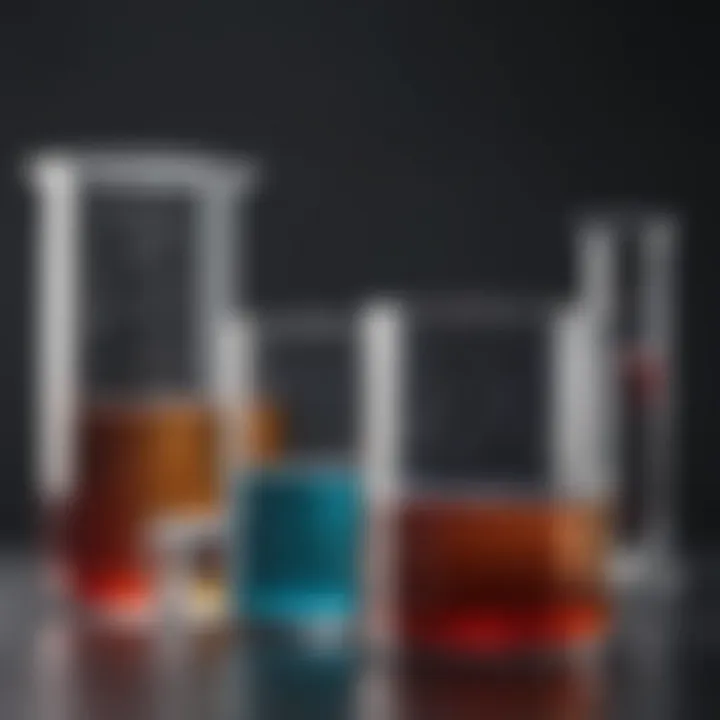
Detectors like mass spectrometry are increasingly beneficial due to their ability to characterize complex compounds at low concentrations effectively. A unique feature of these detectors is their capacity to provide detailed structural information, which is invaluable in both research and industry. However, one downside can be the high operational costs and maintenance requirements.
Data Analysis Techniques
Data analysis in chromatography is paramount for interpreting the results obtained from various chromatographic methods. The techniques employed here significantly enhance the understanding of sample compositions and their respective concentrations.
Peak Identification
Peak identification involves recognizing the distinct peaks on a chromatogram, which correspond to different components in the sample. A crucial aspect of this process is the resolution and specificity of the chromatogram. Peak identification is particularly advantageous as it allows for straightforward differentiation of compounds within a mixture.
Unique features include the automated software tools that facilitate peak analysis, significantly reducing manual interpretation errors. However, one of the predominant challenges can arise in complex samples where overlapping peaks may complicate proper identification.
Quantification Methods
Quantification methods are employed to determine the concentration of the identified peaks. One of the key characteristics here is the precision of numerical results. These methods provide a quantitative measure of analytes, which is vital in regulatory compliance and quality assurance processes.
Unique to quantification methods are the calibration curves which are developed using standards. They allow for accurate extrapolation of concentrations from unknown samples. Nevertheless, the necessity of standards introduces challenges, especially in situations where standards are not readily available or differ significantly from the sample matrix.
Understanding the instrumentation and analysis techniques not only enhances chromatographic efficiency but also broadens the scope of applications across various scientific fields.
Applications of Chemical Chromatography
Chemical chromatography is an essential analytical method in many fields. It allows for the separation and identification of complex mixtures, making it invaluable in research and industry. This technique serves multiple purposes, from quality assurance in manufacturing to environmental monitoring. The versatility of chromatography encourages its adoption across various disciplines, contributing to advancements in technology and safety.
In Pharmaceutical Industry
Drug Development
Drug development is one crucial area where chemical chromatography plays a vital role. In this process, it helps in identifying the chemical composition of new drugs. A key characteristic of drug development is the necessity for precision. Chromatographic methods ensure that pharmaceutical compounds are within strict purity and potency guidelines. This reliability makes chromatography a popular choice in the development phase of drugs.
A unique feature of this aspect is its ability to analyze small sample sizes effectively. This is particularly advantageous because it facilitates the testing of new drugs without wasting valuable resources. However, the complexity of some drug formulations can pose challenges during the analysis.
Quality Control
Quality control is another significant application of chromatography in the pharmaceutical industry. This process is essential for ensuring that drugs meet the required standards before reaching consumers. The main characteristic of quality control is the systematic evaluation of drug products. Such testing prevents the distribution of defective or harmful products. Chemical chromatography provides precise results that validate product safety and efficacy.
One unique advantage of quality control through chromatography is its capacity for continuous monitoring. This is especially crucial for large-scale production, as it permits timely detection of any deviations. However, the cost of implementing advanced chromatographic systems can be a limitation for smaller manufacturers.
Environmental Analysis
Pollutant Detection
Pollutant detection is an essential application of chemical chromatography. This process plays a critical role in environmental monitoring and protection. The key characteristic here is the ability to pinpoint toxic substances in air, soil, and water samples. Effective pollution control is beneficial for public health and safety, making this application particularly relevant today.
A unique aspect of pollutant detection through chromatography is its sensitivity. Many chromatographic techniques can detect parts per billion of hazardous materials. However, sample preparation can be tedious, sometimes requiring extensive methodology before analysis can commence.
Water Quality Testing
Water quality testing is another vital application of chemical chromatography. This process ensures that drinking water is safe and free from harmful contaminants. The key characteristic of water quality testing is the focus on both chemical and biological parameters. The accuracy of results obtained via chromatography supports public health initiatives and compliance with legal standards.
One unique advantage of chromatography in this context is its ability to analyze multiple contaminants simultaneously. This makes the process efficient, although maintaining consistency in sampling conditions can be challenging and resource-intensive.
Food Safety Testing
Contaminant Analysis
Contaminant analysis in food safety is a prominent application of chemical chromatography. This technique helps detect and quantify various chemicals that might pose health risks. The key characteristic of contaminant analysis is the rising concern surrounding food safety for consumers.
A unique feature of this application is its capability to analyze various types of food products. This makes it adaptable for different commodities, from processed foods to fresh produce. Yet, the challenge lies in differentiating between natural and synthetic contaminants, which can complicate interpretations of results.
Flavor Profile Analysis
Flavor profile analysis is another significant application in the realm of food safety testing. Here, chromatography aids in identifying and quantifying flavor compounds in food products. The main characteristic of this analysis is that it enhances product development and consumer satisfaction. This aspect helps companies create better products by understanding consumer preferences.
A unique advantage is that such analysis can lead to innovative developments in food technology. However, the subjective nature of flavor can introduce variability in results, complicating the evaluation process.
Forensic Science
Substance Identification
Substance identification in forensic science is a critical application of chromatography. This process aids in forensic investigations by providing clear evidence for legal proceedings. Its key characteristic is the ability to analyze complex mixtures, such as those found in toxicology cases.
One unique feature of substance identification is the precision it offers in determining substances present in a sample. This leads to reliable results that can withstand judicial scrutiny. However, the extensive training required to interpret chromatographic data can be a barrier for some practitioners.
Toxicology Reports
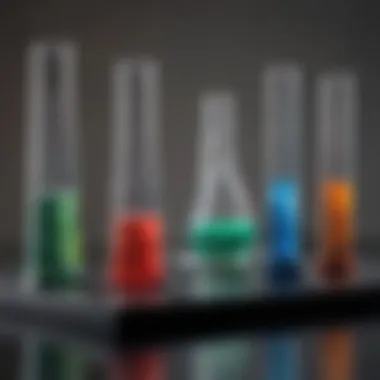
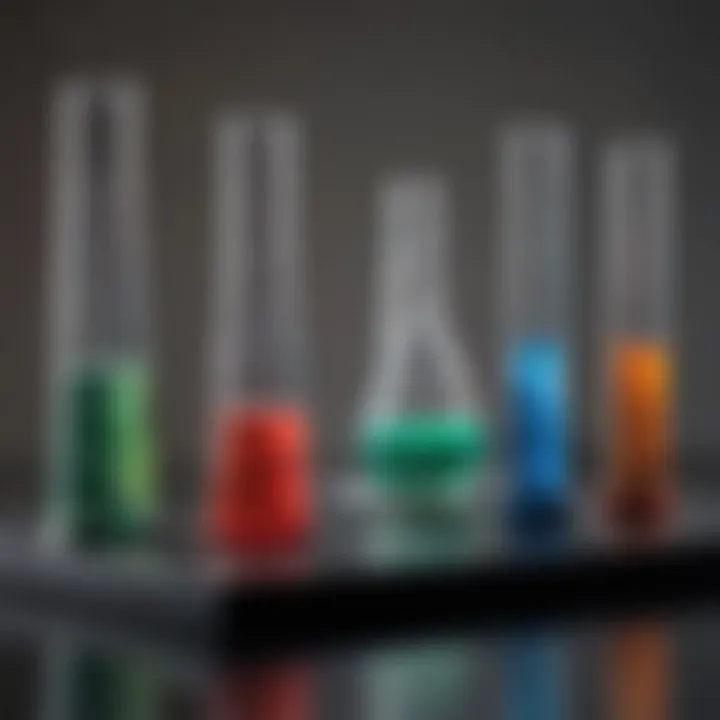
Toxicology reports represent another essential application in forensic science. These reports provide vital information about exposure levels to various toxins or drugs. The key characteristic of toxicology reports is their role in understanding the effects of substances on human health.
A unique aspect of using chromatography in toxicology is its rapid turnaround time for test results. This is crucial in investigations where timing is important. However, advancing technology demands ongoing training for forensic professionals to keep up with new methods and techniques.
Overall, chemical chromatography is crucial across various sectors, enhancing safety, quality, and understanding of complex materials. Its adaptability to different applications underscores its importance in both research and practical contexts.
Emerging Trends in Chromatography
Emerging trends in chromatography reflect the evolution of this important analytical technique. These new approaches and technologies are designed to enhance efficiency, sensitivity, and sustainability in various applications. Understanding these trends is essential for professionals who wish to stay ahead in the field. The focus on miniaturization, integration with other analytical methods, and the adoption of green practices are reshaping the landscape of chromatography today.
Miniaturization of Chromatographic Techniques
Miniaturization has become a significant trend in chromatography, driven by the demand for faster analyses and reduced reagent consumption. Smaller column sizes and volumes are now available, making it easier to perform high-throughput analyses. These techniques consume less solvent and generate less waste, which is important in the context of environmental sustainability. Applications in pharmaceutical and environmental analysis show promise, where sample size and speed are critical. Developments in microfluidics have led to more efficient separation and detection processes, allowing for on-chip analysis. Importantly, these advancements enable researchers to study complex mixtures more effectively, improving data quality and reliability.
Integration with Mass Spectrometry
The integration of chromatography with mass spectrometry (MS) is transforming analytical approaches across many disciplines. This combination allows for the identification and quantification of compounds with high sensitivity and specificity. When used in tandem, chromatography separates the components of a sample, while mass spectrometry provides detailed information about molecular weight and structure. This hybrid technique is particularly beneficial in pharmaceutical research, environmental monitoring, and proteomics. The increased accuracy and rapid analysis times foster more reliable results and extensive data interpretation. Moreover, the use of automated systems in this integration has streamlined workflows in laboratories.
Green Chromatographic Practices
With an increasing emphasis on sustainability, green chromatographic practices are gaining traction. These approaches focus on reducing the environmental impact of chromatography through eco-friendly solvents and minimizing waste. Techniques such as supercritical fluid chromatography utilize carbon dioxide instead of hazardous solvents, lowering toxicity and risk. The development of biodegradable materials for stationary phases is also a part of this trend. By prioritizing green chemistry principles, researchers can contribute to a more sustainable future while maintaining analytical performance. These practices not only align with global efforts toward sustainability but also enhance the reputation of research institutions committed to environmental responsibility.
Challenges and Limitations
Chemical chromatography is an essential analytical tool; however, it faces several challenges and limitations that must be addressed to improve its efficacy and applicability. Understanding these issues is crucial for chemists and researchers who rely on chromatography for accurate results. This section delves into three primary challenges: sensitivity and detection limits, complex sample matrices, and cost and accessibility. Each of these areas presents unique considerations that can impact the effectiveness of chromatographic techniques.
Sensitivity and Detection Limits
Sensitivity in chromatography refers to the ability to detect low concentrations of analytes in a sample. Many applications, such as environmental monitoring or pharmaceutical development, often require high sensitivity for accurate measurements. The detection limits vary across different chromatography techniques, such as gas chromatography compared to liquid chromatography. Improvements in detector technology, like mass spectrometry, have enhanced the sensitivity of traditional methods, but challenges still exist.
Low sensitivity might result in undetected compounds, leading to incomplete analysis. This is especially problematic in cases where trace levels of a substance could have significant implications, such as in forensic applications or toxicology reports. Therefore, researchers must carefully select appropriate techniques and optimize conditions to ensure that detection limits meet the necessary requirements for their specific field.
Complex Sample Matrices
Many real-world samples consist of complex mixtures with multiple components. This can complicate the separation process in chromatography. Biological samples, such as blood or tissue, and environmental samples, such as soil or water, often present particularly difficult matrices. The presence of co-eluting substances can obscure the detection of target analytes and complicate quantitative analysis. As a result, impurities from the sample matrix can affect both the retention time and resolution of the separation.
To overcome these challenges, various sample preparation techniques are utilized, including solid-phase extraction and liquid-liquid extraction. These methods aim to clean up the sample and concentrate the analytes of interest prior to analysis. Despite such advancements, complexity in sample matrices remains a major limitation facing chromatographers.
Cost and Accessibility
The cost of chromatographic equipment and the consumables required for operation can be a significant barrier for many laboratories and research institutions. For example, high-performance liquid chromatography (HPLC) systems, while powerful and effective tools, often come with substantial initial investments and ongoing maintenance costs. The requirement for skilled operators and training further adds to the financial burden.
Moreover, not all researchers have equal access to chromatography techniques. This is especially true in resource-limited settings or in developing regions where modern analytical equipment might not be available. Addressing these challenges requires innovative solutions, such as the development of more affordable, portable chromatography systems that can provide reliable results without compromising quality.
Future Directions in Chromatography
Chemical chromatography has become an indispensable tool in various fields. As technology advances, it opens new avenues for research and application. Understanding the future directions in chromatography is crucial for those who work with this analytical method. Researchers, educators, and professionals in the field need to recognize ways chromatography might evolve. This awareness can lead to more accurate analyses and innovative research methodologies.
Innovative Separation Technologies
The field of chromatography is constantly evolving. Innovative separation technologies are key in increasing efficiency and accuracy of analyses. For instance, recent advancements focus on improving the speed and selectivity of separations. Techniques such as electrochromatography and microfluidics have emerged. These methods allow for miniaturized, high-throughput analyses that were not possible before.
Furthermore, advances in stationary phase materials, including nanomaterials, offer enhanced interactions with target compounds. This can lead to better resolution of complex mixtures. Researchers are increasingly focused on creating more robust and versatile systems. This exploration benefits industries from pharmaceuticals to environmental analysis, where precision is critical.
- Nanomaterials: Enhance interactions in separation processes.
- Microfluidic devices: Allow for rapid analysis of samples.
- Electrochromatography: Offers faster separation times.
AI and Automation in Chromatography
Artificial Intelligence and automation have the potential to transform chromatography. By integrating AI, researchers can analyze vast amounts of data quickly. Machine learning algorithms can assist in pattern recognition, leading to quicker identification of substances. This technology can also optimize separation parameters in real time for improved performance.
Automation facilitates the reproducibility of results. Automated systems can significantly reduce human error in sample prep and analysis. The push towards greater automation also means that laboratories can operate more efficiently, allowing scientists to focus on interpreting results.
- Data analysis: Machine learning improves identification and quantification.
- Process automation: Reduces errors and increases throughput.
- Real-time optimization: Adapts methods based on current data.
Future advancements in chromatography not only promise better precision but also the potential for new applications in emerging sectors like personalized medicine and synthetic biology.
End
The conclusion of this article highlights the extensive role chemical chromatography plays in various scientific fields. It is vital to understand how this analytical technique not only serves to separate components within a mixture but also facilitates deeper insights into complex systems. The diverse methodologies discussed throughout this work illustrate the adaptability of chromatography, presenting it as a fundamental tool across pharmaceuticals, environmental science, food safety, and forensic analysis.
Summary of Key Points
- Wide Application: Chemical chromatography is crucial in multiple industries including pharmaceuticals, environmental monitoring, and food safety.
- Separation Techniques: Various methods such as gas chromatography and liquid chromatography have unique principles and applications, showcasing the versatility of the technique.
- Emerging Trends: The field is evolving with trends like miniaturization and integration with mass spectrometry, pointing toward a future where chromatography becomes even more efficient and accessible.
- Instrumentation: The discussion surrounding the instrumentations such as detectors and data analysis methods is important in understanding how results are achieved and interpreted.
The Significance of Chromatography in Science
Chemical chromatography stands as a pillar in modern analytical chemistry. Its significance lies in the ability to provide precise and reliable separation of components, which is essential in various scientific explorations. This technique not only aids in quality control processes but supports innovative research by suggesting how substances interact in complex matrices.
Moreover, the integration of sophisticated technologies such as artificial intelligence and automation hints at a transformative future for the analytic sciences. Consequently, the relevance of chromatography in unraveling chemical compositions cannot be overstated, as it keeps propelling scientific inquiry to new heights.
"Chemical chromatography is not just a separation technique; it is a bridge to understanding the chemical world in a more detailed manner."