Exploring the Composition and Applications of Lithium

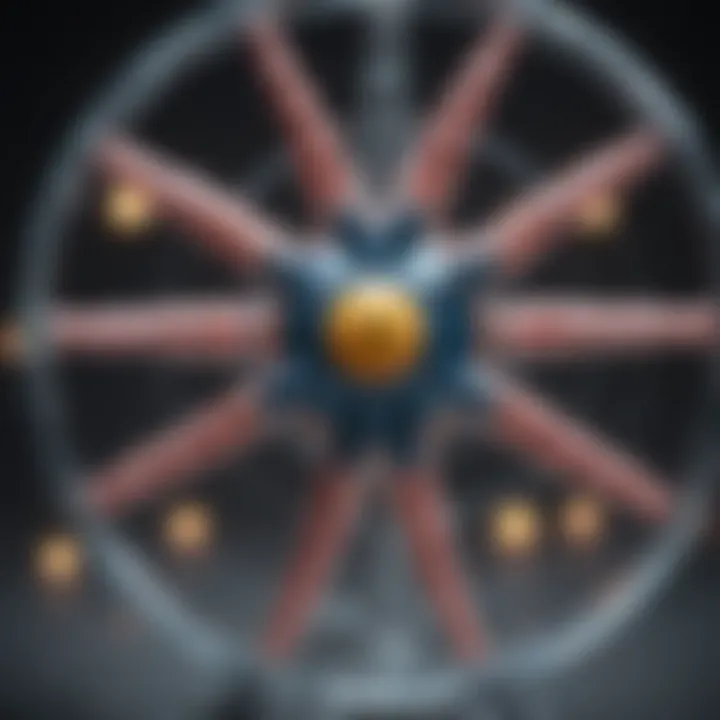
Intro
Lithium, known for its light weight and impressive electrochemical properties, stands out in the realm of materials crucial for modern technology. As the globe increasingly turns towards renewable energy and the electrification of transport, understanding lithium's fundamental elements has never been more essential.
This article aims to dissect the intricacies surrounding lithium, shedding light on its atomic structure, the variations in its isotopes, and the methods used for its extraction. Not only do we aim to uncover the science underpinning lithium, but we also seek to explore its significance within various industries, from electronics to pharmaceuticals.
Understanding lithium goes beyond the surface; it taps into crucial themes like sustainability, energy efficiency, and the role of natural resources in contemporary science. With that, let's delve into the research overview.
Preface to Lithium
Lithium stands out as a key player in today's technology-driven world. The surge in demand for lithium, particularly in energy storage solutions, makes an understanding of its fundamentals not just beneficial but essential. This section serves as an introduction to the fascinating properties and pivotal role that lithium fulfills in various applications. From powering electric vehicles to serving as a mood stabilizer in pharmaceuticals, this element is integral to modern advancements.
Within this discussion, we will look at the specific aspects of lithium that highlight its significance and utility. The exploration ranges from defining lithium clearly—differentiating it from other elements—to examining its historical journey through time. This should provide a contextual basis for understanding the element, as well as how it has become intertwined with contemporary requirements and technology.
Definition of Lithium
Lithium is a soft, silvery-white alkali metal, represented by the chemical symbol Li on the periodic table. It has an atomic number of 3, making it one of the lightest metals available. Notably, lithium has remarkable properties that distinguish it from other metals, including a low density and high electrochemical potential, making it invaluable in batteries.
The element's most common naturally occurring forms are as minerals such as spodumene and lepidolite. Due to its high reactivity, lithium does not exist freely in nature; rather, it's often found bonded to other elements in compounds. It stands out for its ability to provide energy density that far exceeds that of conventional battery materials, making it a primary choice in the lithium-ion battery technology that powers today's devices.
Historical Context
The journey of lithium began in the early 19th century when it was first isolated in 1817 by Swedish chemist Johan August Arfwedson. The discovery came about while studying petalite, a lithium-containing mineral. This marked the beginning of lithium's recognition in the scientific community. However, it wasn't until the mid-20th century, particularly during the Cold War era, that the true potential of lithium was harnessed, paving the way for its application in military technology.
The turning point for lithium usage came in the 1970s with the development of lithium-ion batteries, which heralded a new era in portable electronics. As mobile devices and electric cars started to hit the market, lithium's significance skyrocketed, and it became a hot commodity on the global stage. Its role in not only improving energy storage but also in enhancing the efficiency of various technologies highlights its importance. Today, as we face the challenges of climate change and the need for sustainable energy solutions, lithium's relevance continues to grow, making it a focal point of much ongoing research and innovation.
"Lithium is not just a component; it’s transforming our energy landscape, carrying us into a more sustainable future."
Understanding lithium, from its definition to its historical significance, sets the groundwork for exploring its atomic structure, natural occurrence, and myriad applications.
Atomic Structure of Lithium
The atomic structure of lithium gives insight into its various properties and behaviors, especially when it comes to its applications in technology and science. Understanding lithium's atomic composition is crucial, as it lays the groundwork for comprehending its reactivity and role in a myriad of chemical processes. The arrangement of protons, neutrons, and electrons not only dictates lithium's interaction with other elements but also defines its unique standing in the realm of alkali metals.
Nuclear Composition
Lithium's nuclear composition consists of three protons and, depending on its isotope, either three or four neutrons. This simple yet elegant structure is what sets the stage for lithium's unique characteristics. The presence of three protons categorizes lithium as an alkali metal—elements known for their high reactivity, especially with water.
Lithium's isotopes play a significant role in determining its stability. The most common isotope, lithium-6, is abundant and stable, making it particularly useful in various applications, including nuclear fusion reactions and certain medical treatments. Conversely, lithium-7, another isotope, has its own significance, particularly in the realm of nuclear science. The understanding of these isotopes provides a deeper insight into lithium’s versatile nature.
Electron Configuration
Lithium's electron configuration is quite straightforward: it has an atomic number of 3, which allows it to hold three electrons. The configuration is represented as 1s² 2s¹. The first two electrons fill the inner shell, while the third resides in the second shell. This configuration not only makes lithium reactive but also highlights its tendency to lose that one outer electron rather easily.
This ease of electron donation explains why lithium does not linger in nature for long. It swiftly reacts—forming positive ions as it seeks stability, often combining with other elements to form various compounds. The behavior of lithium in its electron configuration underscores its importance as a key player in organic chemistry and battery technology.
Atomic Mass and Isotopes
The atomic mass of lithium is approximately 6.94 u, reflecting both its stable isotopes. However, it’s crucial to examine its isotopes separately for a more nuanced understanding.
Stable Isotopes of Lithium
Lithium exists primarily in two stable forms: lithium-6 and lithium-7. These isotopes contribute significantly to both scientific research and practical applications. Lithium-6, with its smaller neutron count, is often utilized in nuclear fusion and in the production of tritium.
"The stability of these isotopes makes them invaluable selections in fields ranging from pharmaceuticals to energy."
On the other hand, lithium-7 finds its utility in nuclear reactors and as a coolant in fusion reactors. It has other applications in mental health medications too, especially for bipolar disorder, due to its therapeutic effects.
Radiogenic Isotopes and Their Significance
Radiogenic isotopes of lithium, like lithium-8, arise from the decay of heavier elements and offer unique insights into geochemical processes. While these isotopes are less stable, their production and detection can reveal substantial information about conditions in which lithium forms, such as within certain types of stars or in geological processes.
The insights gained from studying radiogenic isotopes can inform our understanding of elemental distributions in various environments. Plus, the potential for using these isotopes in tracing elements in geological formations underscores their relevance. Their varying stabilities compared to lithium's stable counterparts present distinct challenges and opportunities when it comes to research.
In summary, the atomic structure of lithium—from its nuclear make-up to its electron configuration—reveals a lot about the element's reactivity and usefulness in modern applications. Understanding its stable and radiogenic isotopes adds further depth to this exploration, illustrating why lithium continues to be an essential area of study in chemistry and materials science.
Position of Lithium in the Periodic Table
Understanding lithium's position in the periodic table is crucial for grasping its chemical behavior and its applications. Lithium is seated comfortably in group 1, which is home to the alkali metals. This positioning affects its reactivity, bonding tendencies, and other fundamental properties, making it a pivotal element in both theoretical and applied chemistry.
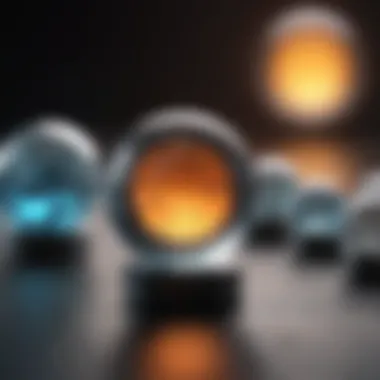
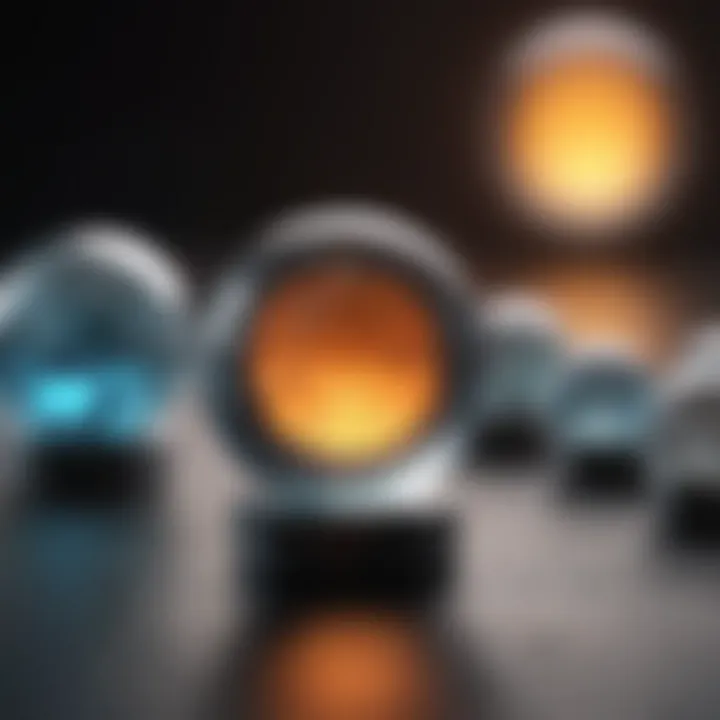
Group and Period Characteristics
Lithium occupies the first group of the periodic table, specifically as the third element of this group. With an atomic number of 3, its placement indicates that it has one electron in its outermost shell, echoing the typical characteristic of alkali metals which is to be highly reactive.
- Metallic Nature: As a metal, lithium exhibits a silvery-white luster and is softer than most metals. It can be cut with a knife, adding a tactile dimension to its atomic structure.
- Electrical Conductivity: Like other alkali metals, lithium is a good conductor of electricity due to its free-moving outer electron. This property leads to its essential use in batteries.
- Chemical Reactivity: Being highly reactive, lithium combines easily with many nonmetals and is flammable, particularly in the presence of moisture. It reacts rapidly with water, forming lithium hydroxide and releasing hydrogen gas. This reactivity is significantly higher than that of other alkali metals when considering its smaller size and charge density.
These attributes highlight lithium's distinct persona within its group and affirm its foundational role in chemical reactions.
Relation to Other Alkali Metals
When we contemplate lithium’s relationship with its fellow alkali metals such as sodium, potassium, and rubidium, a few key differences become evident.
- Reactivity Patterns: Lithium is less reactive than sodium and potassium, exhibiting unique behaviors related to its smaller atomic radius. The trend generally shows that as we move down the group, reactivity increases; this trend is a critical consideration for chemists when selecting alkali metals for specific applications.
- Electronegativity: Lithium has a higher electronegativity compared to its sibling elements in group 1. This affects how lithium forms compounds and participates in chemical reactions.
- Applications: Lithium is somewhat unique because its lower atomic weight and size enable it to be particularly useful in applications that require lightweight materials, such as aerospace technology or high-performance batteries.
"Lithium shines in the world of modern technology while holding onto ancient characteristics binding it to its alkali counterparts."
Overall, while lithium might seem like just another member of its group, its unique properties and nuances set it apart from other alkali metals, paving the way for its vital role in both industry and technology.
Occurrence of Lithium in Nature
Understanding how lithium occurs in nature is fundamental to grasping its significant role in various industries. Lithium is not found freely in its elemental state due to its high reactivity; rather, it exists in mineral and brine sources, which vary in extraction methods and availability. Exploring these natural deposits provides insights into lithium's use and its overall impact on modern technology.
Natural Deposits
Mineral Sources
Mineral sources of lithium come primarily from pegmatitic ores like spodumene, lepidolite, and petalite. Spodumene, for example, is a key mineral and has a rich lithium concentration, making it the go-to choice for lithium extraction in many mining operations. The uniqueness of mineral deposits lies in their solid-state occurrence, which provides advantages like concentration of lithium, reduced processing times, and overall higher yields of lithium.
However, mining these minerals can have environmental consequences, such as habitat disruption and water pollution. Striking a balance between extraction efficiency and environmental protection remains a considerable challenge in the field.
Brine Sources
In contrast to minerals, brine sources are more about extracting lithium from salt lake deposits. This method typically involves pumping brine from underground reservoirs, allowing it to evaporate in large ponds under the sun. This form of extraction is often considered beneficial as it tends to use less energy and has a lower carbon footprint compared to mining solid minerals. Moreover, brine sources provide high concentrations of lithium, which again aids in the efficiency of extraction processes.
However, the downside of brine extraction lies in the prolonged evaporation process and risk of contaminating local water supplies. This poses a threat not only to ecosystems but also to communities that depend on these water sources.
Geochemical Behavior
Lithium's behavior in the geochemical context is quite intriguing. Being a light alkali metal, lithium behaves differently in various natural environments, including its solubility and mobility in groundwater or soil.
- Mobility: Lithium ions can easily move through rock and soil, affecting its distribution in the environment.
- Reactivity: It interacts with other ions, forming compounds that can either be beneficial or detrimental in certain contexts—especially when considering soil and water quality factors.
Understanding the geochemical behavior can pave the way for more sustainable extraction practices and help manage natural resources more effectively. In summary, exploring lithium's natural deposits not only lays the groundwork for its extraction methods but also opens up discussions about environmental responsibilities and sustainability in mining practices.
Extraction Methods of Lithium
Extraction methods for lithium are crucial to understanding how this element is harvested for various applications, particularly in the production of batteries. As the demand for lithium continues to surge, the methods by which we extract it are equally gaining focus. A thorough comprehension of these techniques sheds light on their efficiencies, environmental impact, and relevance in meeting the growing market needs.
Mining Techniques
The mining techniques used to extract lithium can be classified into two main categories: open-pit mining and underground mining. Each has its own distinct advantages and challenges, contributing differently to the overall extraction process.
Open-Pit Mining
Open-pit mining is characterized by its method of removing large amounts of earth to access lithium-rich minerals near the surface. This technique is extensively used for lithium extraction due to several reasons.
One of the key benefits of open-pit mining is the relatively lower cost compared to other methods. Since the lithium-containing ores are more accessible, this method can yield significant quantities of lithium at a lower operational cost. Moreover, the process allows for better inspection of rock formations, which enhances safety measures and operational efficiency.
However, the process doesn't come without its downsides. The environmental impact of open-pit mining is a pressing concern. The destruction of natural habitat, soil erosion, and pollution risks associated with runoff can’t be overlooked. Thus, while it is a popular choice for economical extraction, its environmental footprint remains a topic of debate.
Underground Mining
Underground mining, on the other hand, involves digging tunnels to reach deposits located deeper in the earth. This method is typically employed for extracting lithium when the ore is not viable from the surface.
A major advantage of underground mining is its potential to reduce surface impact. By confining the extraction to below ground, the method helps preserve the landscape and minimize direct environmental degradation. This is particularly significant in sensitive ecological regions where open-pit mining might cause substantial harm.
Despite these advantages, underground mining has a higher operational cost. The need for extensive ventilation systems, safety measures, and specialized equipment can escalate expenses significantly. Furthermore, accessing lithium at deeper levels may require more advanced technologies and can introduce more complex geological challenges.
Processing of Lithium Ores
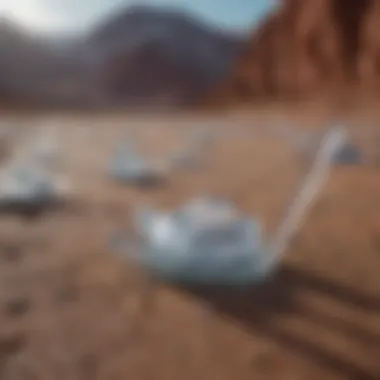
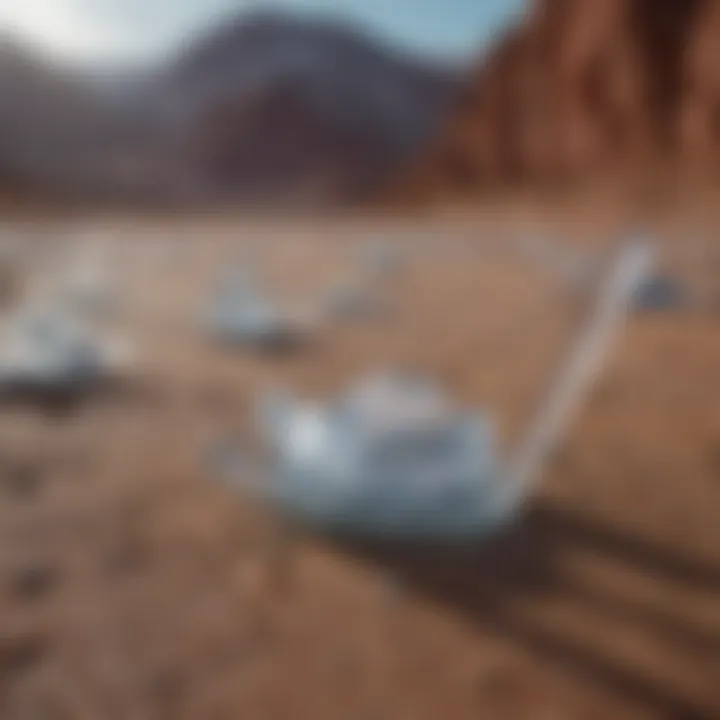
Once harvested, lithium ore must undergo a processing phase to separate lithium from other materials. This involves crushing the ore and utilizing various chemical processes, often including flotation, leaching, and roasting. The goal here is to produce lithium concentrates that are ready for further refining or conversion into lithium compounds suitable for marketplace needs.
Evaporation Techniques in Brine Extraction
Evaporation techniques are particularly prevalent in extracting lithium from brine sources, such as salt flats. This process revolves around flooding evaporation ponds where brine can be left to evaporate, concentrating the lithium as water dissipates. The simplicity and cost-effectiveness of this method make it attractive, especially in regions with hot, sunny climates. However, the time required for evaporation and the potential for contamination with other salts are significant factors that can influence overall yield.
Lithium extraction methods collectively play a vital role in not just getting this essential resource into the market but doing so in a manner that balances economic viability and environmental sustainability.
Chemical Properties of Lithium
Understanding the chemical properties of lithium is essential as they play a crucial role in its applications and interactions in various contexts. In this section, we'll dissect lithium's behavior with water, its reactions with halogens, and its significance in organometallic chemistry. The characteristics of lithium not only help in grasping its fundamental role in scientific endeavors but also allow us to appreciate its wide-ranging implications in modern technology.
Reactivity with Water
Lithium's reactivity with water is a notable feature that distinguishes it from many other metals. When lithium is placed in water, it reacts vigorously to produce lithium hydroxide and hydrogen gas. This reaction is often less violent compared to other alkali metals like sodium and potassium; nonetheless, it's still significant. The balanced chemical equation for this reaction can be written as:
The formation of lithium hydroxide, a strong base, contributes to the unique properties of lithium. This establishes that lithium doesn't just react with water, but it also alters the chemical environment, producing alkaline conditions. The hydrogen gas released can be ignited, further emphasizing the energetic nature of the reaction. Thus, this interaction is pivotal not only in demonstrating lithium’s reactivity but in applications such as chemical synthesis and energy storage contexts.
Reaction with Halogens
Lithium exhibits a strong affinity for halogens, forming ionic compounds that show critical characteristics important in various fields. When lithium reacts with halogens, a notable example would be with chlorine, forming lithium chloride, represented as follows:
In this reaction, lithium donates its one valence electron to chlorine, resulting in a polar ionic bond. Lithium chloride then becomes a vital substance, utilized in many industrial applications, including chemical hydration, and as a flux in metallurgy. The stability and high melting point of lithium halides underscore their significance in organometallic chemistry and material sciences.
Lithium in Organometallic Chemistry
When exploring lithium's role in organometallic chemistry, the focus lies in its ability to form stable organolithium compounds, which serve as ideal reagents in organic synthesis. These compounds, like butyllithium, exhibit unique properties like high reactivity and selectivity.
Organolithium compounds are notable for being strong bases and nucleophiles, making them instrumental in many synthetic pathways. They can effectively react with carbonyl compounds, alkyl halides, and other electrophiles, helping maintain a steady workflow in organic laboratories. Their reactions typically lead to the formation of new carbon-carbon bonds, which is paramount for constructing complex organic molecules.
Moreover, their use in polymerization reactions, particularly in producing specialty polymers, underscores their relevance in advanced materials research.
A deeper understanding of lithium’s reactivity with water, halogens, and its role in organometallic chemistry provides insights into its versatility and significance in both laboratory and industrial settings.
Applications of Lithium
Lithium's multifaceted applications extend well beyond mere academic curiosity; they are at the heart of many modern innovations and industries. As the demand for energy storage solutions rises, lithium has carved out its place, especially in the realm of batteries. This section delves into the specific roles lithium plays in technology, healthcare, and aerospace, showcasing its strengths and the implications of its use.
Role in Lithium-Ion Batteries
Lithium's Role in Energy Storage
When we talk about lithium in the context of energy storage, the conversation often gravitates towards lithium-ion (Li-ion) batteries. These batteries have become the backbone of portable electronics and electric vehicles. The key reason for lithium’s prominence is its lightweight nature, which allows for high energy densities—crucial for powering devices where every ounce counts.
Moreover, lithium's electrochemical properties enable these batteries to have a significant charge capacity. When discharged, lithium ions move from the anode to the cathode through an electrolyte, allowing for efficient energy transfer. This unique feature not only amplifies battery life but also hastens charging times, making lithium-ion batteries a popular choice in various applications. However, there are chatters about thermal stability, where overheating can lead to hazards, demanding continual improvements in safety measures.
Impact on Modern Electronics
The impact of lithium on modern electronics is profound; it has reshaped how we think about portable technology. From smartphones to laptops and tablets, the presence of lithium-ion batteries provides a stable, long-lasting power source that meets the needs of our fast-paced world. The key characteristic here is their high charge retention and the ability to support rapid discharge rates, often necessary for demanding tasks.
Despite its advantages, lithium's role in electronics does carry downsides. There is ongoing scrutiny regarding the sourcing of lithium, particularly with regard to environmental impacts from mining practices. This reality underscores the critical need for companies to adopt sustainable practices as technology evolves.
Use in Pharmaceuticals
Lithium's versatility doesn't stop at technology. In the field of mental health, lithium carbonate has long been prescribed as a treatment for bipolar disorder. By affecting neurotransmitter release in the brain, lithium can stabilize mood swings, making it a valuable component in psychiatric medicine. The specific aspect of lithium in this context has been its neuroprotective properties, which help in preventing mood episodes. Despite its efficacy, the narrow therapeutic range presents challenges; monitoring is crucial to avoid toxicity. Therefore, careful administration and ongoing studies continue to shape its guidelines in psychiatry.
Applications in Aerospace and Defense
In aerospace and defense, lithium compounds find their way into various applications, from primary battery systems for critical operations to lighter metals in structural components. The aerospace industry highly values lithium-aluminum alloys for their low weight and high strength, helping to improve fuel efficiency.
The unique advantage here is that lithium enhances performance without significantly increasing costs. Yet, it demands careful management due to its reactivity, especially under specific environmental conditions. How industries address these challenges will define the future of lithium in these high-stakes fields.
"Lithium has solidified its role as a driving force behind innovations that rely on efficient, portable energy solutions."
As we explore lithium’s various applications, it becomes clear that its implications touch on sustainability and technological evolution—a balancing act as industries look towards the future.
Environmental and Economic Considerations
The intersection of environmental and economic factors regarding lithium is intricate and crucial. Lithium has become imperative not only for technological advancements but also for the long-term sustainability of these resources. Understanding these considerations open the door to a more comprehensive view of hopeful outcomes and unavoidable challenges tied to lithium mining and usage, making it a vital area of focus.
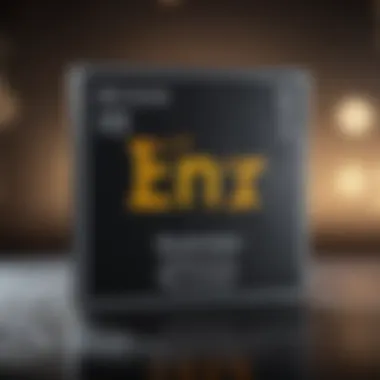
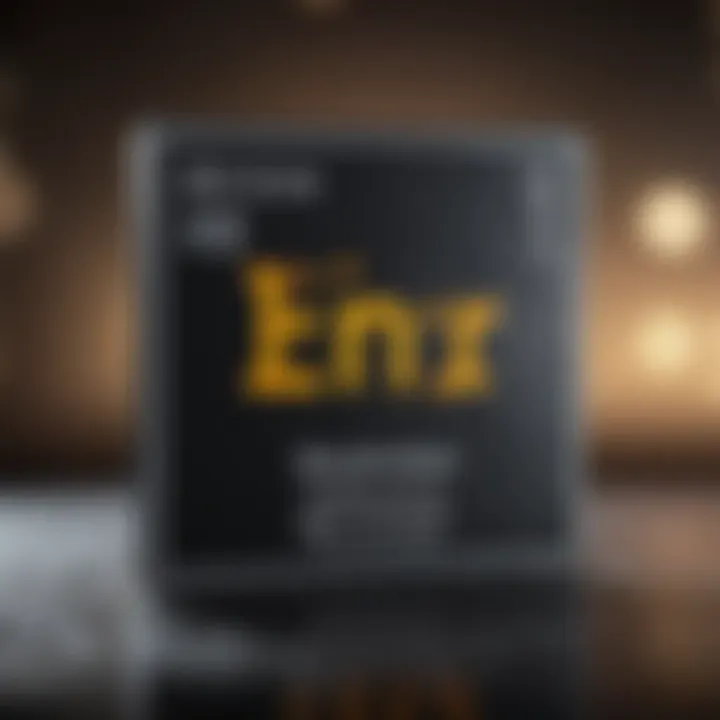
Sustainability of Lithium Mining
The sustainability of lithium mining carries great weight in discussions about its overall impact. Mining operations, especially in places like the Salar de Uyuni in Bolivia or the Lithium Triangle in South America, have raised eyebrows concerning water consumption and ecosystem disruption. These regions are characteristically arid, where water is a precious commodity. The extraction processes often draw significant amounts from local water resources, essential for wildlife and communities.
Efforts to mitigate environmental impacts are critical. Industries are looking to improve operational fluidity by focusing on circular economy principles. This approach suggests reducing waste and recycling lithium materials from battery production and disposal. It means creating a battery lifecycle that is not just profitable, but also mindful of minimizing the ecological footprint.
Market Dynamics and Pricing
The economic landscape around lithium is dynamic and heavily influenced by technological trends, global policies, and consumer demands. Understanding these fluctuations aids in grasping how lithium maintains its relevance in the modern world.
Global Demand for Lithium
In recent years, the global demand for lithium has skyrocketed, largely propelled by the increasing adoption of electric vehicles (EVs) and renewable energy storage. This surge is a direct response to the worldwide push toward greener solutions to combat climate change. Countries, especially China and the United States, are ramping up production to meet their targets for carbon neutrality.
One key characteristic of this demand is its volatility. With the buzz around EVs and battery technologies, it seems like lithium is everywhere at the moment, making it a decidedly hot commodity. While this demand creates vast economic opportunities, it’s critical to consider how quickly it can fluctuate based on market trends, technological innovations, and policy changes.
- Advantages of this soaring demand include:
- Heightened investment in lithium mining.
- Potential job creation in mining and battery production.
- Accelerated research into sustainable extraction methods.
However, it's crucial to be cautious. The stakes are high, and sudden shifts in technological preferences or economic downturns can put extensive pressure on lithium's market stability.
Future Trends in Lithium Prices
The future trends in lithium prices are pivotal not just for investors but also for industries reliant on this element. Many forecasters suggest that prices will remain elevated for the foreseeable future driven by persistent demand for electric vehicles and energy storage solutions. Batteries are the backbone of this demand—without them, the transition to a low-carbon economy may stall.
One distinctive feature of predicting lithium prices is the integration of sustainable practices with market needs. As companies adopt environmentally friendly mining methods and emphasize recycling, this may lead to more stable prices over time.
- Unique aspects of future lithium pricing include:
- Increased entry of secondary markets looking to recycle lithium from old batteries.
- Potential geo-political influences as nations vie for lithium resources.
The road ahead is undoubtedly paved with both challenges and opportunities, making it vital for stakeholder to have a firm grip on these dynamics. As technology evolves, the expectations and stability of lithium prices will increasingly transform.
"Navigating the tangled web of lithium's market dynamics requires foresight and adaptability, as both environmental and economic considerations shape its future."
In summation, understanding the environmental implications of lithium mining and its fluctuating market dynamics provides insight into this essential element's role in a sustainable technological future.
Future of Lithium Research
As the world continues to shift towards sustainable energy solutions, the future of lithium research emerges as a critical area of study. This focus on lithium is driven by its essential role in powering batteries, particularly in electric vehicles and renewable energy storage systems. Given the increasing global demand for lithium-ion batteries, innovations in research are not only crucial for enhancing battery performance but also for addressing broader ecological and economic concerns.
Innovations in Lithium Battery Technology
Innovations in lithium battery technology are paving the way for significant breakthroughs. Researchers are exploring various avenues to improve energy density, longevity, and charging speed. For instance, one area of focus is the development of solid-state batteries. Unlike traditional lithium-ion batteries that use liquid electrolytes, solid-state batteries employ solid electrolytes, which can potentially increase energy storage capacity while reducing flammability risks.
Moreover, advancements in lithium-sulfur batteries are also making waves, offering the promise of higher theoretical capacity compared to conventional lithium-ion configurations. This type of technology harnesses the qualities of sulfur, more abundant than cobalt, thus reducing reliance on finite resources. In addition, ongoing research into recycling processes for lithium batteries is gaining momentum. Properly recycling lithium batteries can ensure that valuable materials are not wasted, thus sustaining supply chains and reducing environmental impact.
Alternative Sources of Lithium
With the demand for lithium on the rise, researchers are turning to alternative sources of lithium to diversify supply and mitigate environmental impacts. This includes tapping into unconventional deposits like seawater. It is estimated that seawater contains millions of tons of lithium; however, the extraction process is currently not economically viable. Researchers are working on cost-effective methods to extract lithium from seawater, which could revolutionize how we source this vital element.
Another promising alternative comes in the form of recovered lithium from aquifers and geothermal brines. Some geothermal power plants are equipped with technologies to extract lithium while generating renewable energy. This dual-purpose approach could serve both sustainable energy production and lithium recovery, presenting a compelling solution for future demand.
Additionally, mining from artisanal sources and urban mining—the process of reclaiming lithium from used electronics—presents an innovative avenue. As more electronic devices flood the market, urban mining becomes crucial for tapping into existing lithium resources while promoting a circular economy.
"The future of lithium research is not just about finding more lithium; it’s about developing smarter, safer, and sustainable ways to use it."
Through these innovative approaches and continued research, the future of lithium holds exciting potential for meeting energy demands while maintaining a balance with environmental stewardship. The more we uncover about lithium, the better positioned we will be in addressing the myriad challenges that come with a rapidly evolving technological landscape.
Closure
Wrapping up the exploration of lithium, it's essential to reflect on why its composition matters in today's scientific landscape. Lithium is more than just a simple element; it's a cornerstone for various high-tech applications, primarily in energy storage systems like lithium-ion batteries. These batteries have become a fixture in our electronic devices, from smartphones to electric vehicles, highlighting lithium’s fundamental role in modern technology.
Summary of Key Points
In this article, we navigated through several critical aspects that depict the story of lithium:
- Atomic Structure: Dissecting its nucleus and electron configuration illuminates why lithium behaves the way it does in chemical reactions.
- Occurrence: Understanding how and where lithium is found—be it mineral or brine sources—collectively shapes its accessibility.
- Extraction and Chemical Properties: The dynamics of extraction methods are pivotal for meeting the escalating demand for lithium, which has skyrocketed due to its application in batteries.
- Applications: We explored its critical roles across various domains, including pharmaceuticals and aerospace, emphasizing its versatility.
- Future Landscape: Innovations in technology signal a promising horizon, underlining the importance of sustainable practices in mining and production.
Final Thoughts on Lithium's Role in Modern Science
As we glance into the future, lithium stands as a symbol of the crossroads between scientific advancement and practical application. With the world pushing towards greener technologies, lithium's lightweight yet potent nature makes it indispensable. There's a delicate dance of balancing its extraction with ecological considerations, not just for the immediate stakeholders, but for global sustainability.
Lithium isn’t merely a chemical element; it’s a catalyst propelling us into an era defined by efficiency. Its continued study will likely uncover even more profound impacts on technology and our everyday lives.
In summary, lithium's composition and properties serve as a critical framework for understanding its significance in shaping contemporaneous and future technologies with potential to revolutionize energy storage and utilization.