CRISPR and crRNA: Impact on Gene Editing Landscape
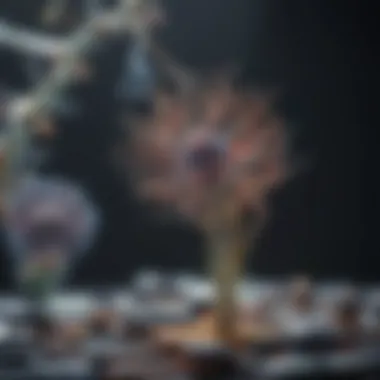
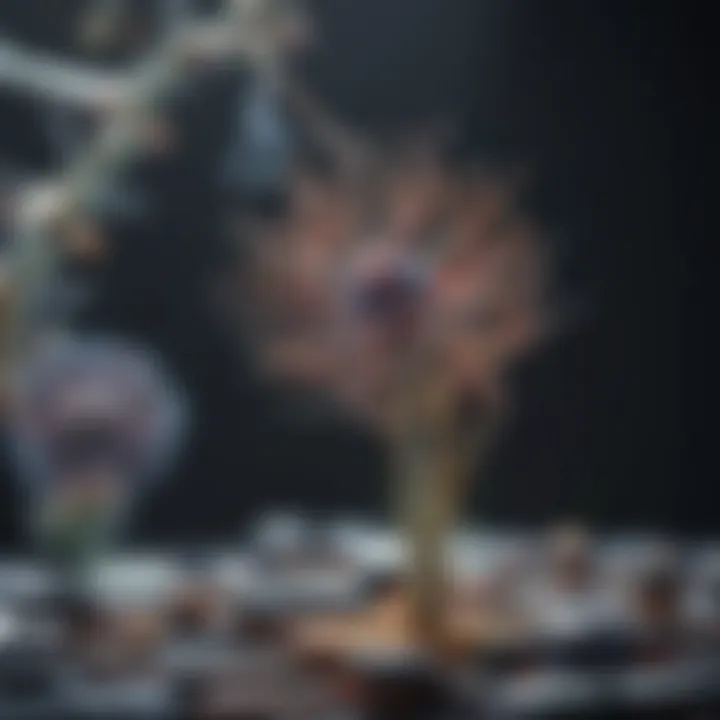
Intro
CRISPR technology has taken the scientific world by storm, reshaping our understanding of gene editing and its possibilities. At the heart of this ground-breaking technology lies CRISPR RNA, often abbreviated as crRNA. This small yet significant molecule plays a pivotal role in the CRISPR system's operation, guiding molecular machinery to target specific DNA sequences with remarkable precision. As scientists strive to harness this powerful tool for various applications, from agriculture to medicine, the discussions around crRNA's functionalities become even more essential.
In this exploration, we'll dive into the details surrounding CRISPR and crRNA, unpacking their mechanisms, the research landscape, and the ethical considerations that accompany their use. The aim is to provide a clearer understanding of these components and their broader implications in genetics and biotechnology.
Research Overview
Summary of key findings
The research surrounding CRISPR technology has yielded fascinating insights. Primarily, studies show that crRNA is integral in guiding the Cas9 enzyme to specific locations on the genome, effectively facilitating targeted DNA modifications. Key findings include:
- crRNA's role as a molecular GPS, ensuring precise editing at the desired loci.
- The stability and efficacy of crRNA have been enhanced through various engineering approaches.
- Advances in delivery mechanisms for crRNA are making it more accessible for therapeutic applications.
Importance of the research in its respective field
The significance of understanding crRNA and its dynamics cannot be overstated. As researchers continuously pioneer new methodologies and applications, crRNA is emerging not just as a tool for gene editing, but also as a strategic part of synthetic biology, therapeutic development, and biotechnology. This technology has the potential to revolutionize fields like gene therapy, agriculture, and even combating infectious diseases. For instance, crRNA's applications in creating genetically modified organisms (GMOs) have sparked significant debates among stakeholders regarding their implications on food security and biodiversity.
"CRISPR and crRNA are not just terms in the lexicon of molecular biology; they represent a paradigm shift in how we approach genetic engineering."
Methodology
Description of the experimental or analytical methods used
The methods employed in the study of CRISPR and crRNA are as diverse as their applications. Many experiments are centered around:
- In vitro assays to test the efficiency of crRNA in guiding Cas9 for targeted edits.
- Transgenic models which help in studying the effects of CRISPR-induced changes in live organisms.
- Bioinformatics tools to analyze the sequences and predict possible outcomes of edits.
Sampling criteria and data collection techniques
To ensure reliable results, the research employs rigorous sampling criteria. For instance, selecting target genes is based on their relevance to specific diseases or traits. Data collection often involves high-throughput sequencing and quantitative PCR methods to measure the efficiency of gene targeting and subsequent phenotypic outcomes.
By laying down these foundational aspects of CRISPR and crRNA, the exploration reveals not just the mechanisms behind the technology but also its expansive potential towards shaping the future of genetic engineering.
Prolusion to CRISPR
The development of CRISPR technology marks a watershed moment in genetic engineering, providing researchers with unprecedented tools to modify DNA with precision. Understanding CRISPR is not just an academic exercise; its implications reverberate through medicine, agriculture, and biotechnology, significantly reshaping how we approach genetic manipulation and treatment of genetic disorders.
CRISPR, which stands for Clustered Regularly Interspaced Short Palindromic Repeats, works by utilizing a unique mechanism derived from bacterial immunity. This mechanism allows for the targeting and modification of genes, offering a highly efficient means of gene editing that is easier and less error-prone compared to past methodologies. Consequently, researchers and practitioners see CRISPR as a critical breakthrough for gene therapy and genetic research.
Moreover, as this technology continues to evolve, it presents not only scientific possibilities but also ethical dilemmas that necessitate careful consideration. Its capacity to alter fundamental genetic codes brings forth discussions on regulation, potential misuse, and the moral ramifications of altering life forms. Thus, an introduction to CRISPR lays the groundwork for ongoing dialogues about its capabilities, challenges, and societal impacts.
Historical Context
The journey of CRISPR began long before it became the darling of genetic engineering. Initially identified in the late 1980s in E. coli, the relevance of these sequences was not immediately recognized. It was only in the early 2000s that scientists started piecing together how these segments functioned as part of the bacterial immune system. By storing snippets of viral DNA, bacteria could recognize and fend off future attacks from similar viruses, a biological function that was pivotal in adapting intensity against external invaders.
Key milestones include:
- 2005: The discovery that CRISPR sequences work in conjunction with Cas proteins, forming a sophisticated defense mechanism.
- 2012: Jennifer Doudna and Emmanuelle Charpentier, through their groundbreaking work, demonstrated how CRISPR-Cas9 could be programmed to target specific stretches of genetic code. This breakthrough opened the door to a whole new realm of possibilities in genetic editing.
- 2013: The first successful demonstration of CRISPR in mammalian cells not only validated its effective application but also sparked an explosion of research activities across the globe.
This historical backdrop is essential for understanding how CRISPR evolved from a bacterial defense mechanism to the forefront of modern gene editing.
Basic Structure of CRISPR Systems
For a clear comprehension of CRISPR technology, one must delve into its fundamental structure. CRISPR systems are composed primarily of three critical components: crRNA, tracrRNA, and Cas proteins. Each element fulfills a distinct role in the gene editing process.
- crRNA (CRISPR RNA): This RNA is a short guide that directs the Cas proteins to specific sites within the target DNA. It is crucial for the recognition of the sequence to be edited.
- tracrRNA (trans-activating CRISPR RNA): Functioning alongside crRNA, tracrRNA aids in stabilizing the interaction between crRNA and the Cas protein, ensuring precise targeting during the editing process.
- Cas Proteins: These are the molecular scissors that execute the cuts in DNA strands. Different Cas proteins, like Cas9 and Cas12, vary in such features as targeting efficiency and specificity.
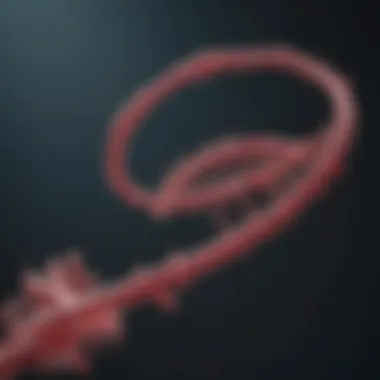
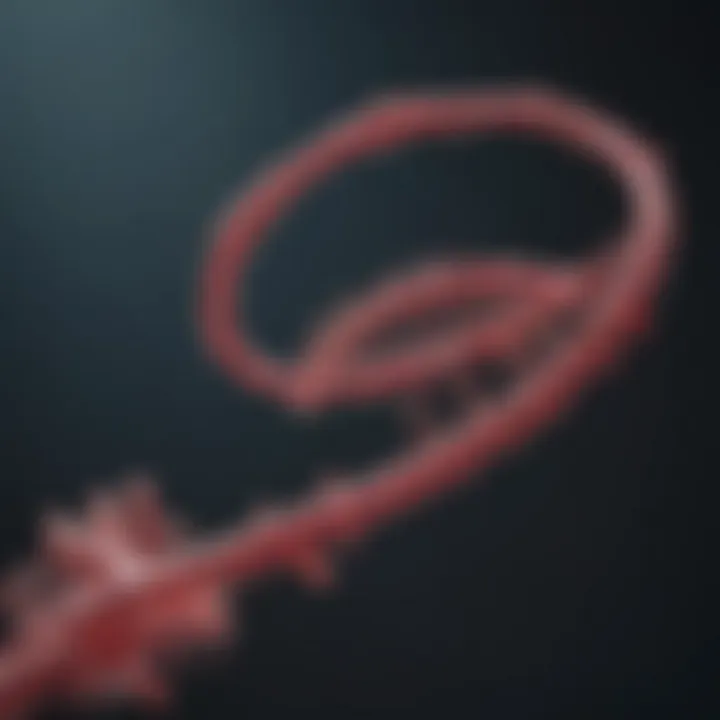
Understanding the basic structure and function of these components lays the foundation for deeper insights into the mechanisms at play during CRISPR's action. With this knowledge, we are better equipped to explore the subsequent complexities and applications presented by CRISPR technology.
Components of CRISPR
The intricate architecture of CRISPR technology is largely underpinned by three fundamental components: CRISPR RNA (crRNA), trans-activating CRISPR RNA (tracrRNA), and Cas proteins. Each of these elements serves a distinct purpose, resembling parts of a machine where every cog is essential to its operation. Understanding these components is crucial, as it paves the way for grasping how CRISPR functions within the gene-editing sphere.
CRISPR RNA (crRNA)
Role in Gene Targeting
The crRNA is the key player in the recognition and targeting of specific DNA sequences. Acting as a guide, crRNA perfectly matches the sequence of the target DNA, a sort of homing beacon directing the Cas proteins where to cut. This targeting ability not only allows precise editing but also minimizes off-target effects, which can lead to unintended mutations. The precise matching capability of crRNA is a prized characteristic, making it a popular choice among genetic researchers. Its unique feature lies in its ability to be designed to match virtually any sequence, offering flexibility in interests ranging from agriculture to medicine. However, developing a crRNA for an entirely new target sequence may require rigorous trials and optimization, which could be a time-consuming endeavor.
Structure and Functionality
Structurally, crRNA has a simple yet elegant design. It is composed of a sequence that corresponds to the target DNA and an essential scaffold that facilitates interaction with Cas proteins. This bifunctional aspect makes crRNA incredibly effective in gene editing. The dual nature of crRNA simplifies the entire mechanism, ensuring that once it's bound to its target, the Cas proteins can do their job effectively. The advantage here is that researchers are able to target multiple genes simultaneously by designing several crRNAs within one experiment. The trade-off, however, is that a misdesign in the crRNA can lead to inefficient editing or off-target mutations, which necessitates careful design and validation.
Trans-activating CRISPR RNA (tracrRNA)
Interaction with crRNA
TracrRNA complements crRNA by stabilizing its structure and enhancing the specificity of target recognition. When crRNA and tracrRNA bind together, they form a complex that is crucial for recruiting Cas proteins to the right location on the DNA strand. This interaction is vital; without the tracrRNA, crRNA would lose its structural integrity and functionality as a guide. Its role is highlighted by the charm of synergy, transforming two separate components into a highly functional unit. The downside is that working with tracrRNA adds another layer of complexity to CRISPR applications; researchers must fine-tune this interaction to achieve the desired outcomes.
Function in the CRISPR Mechanism
In terms of functionality, tracrRNA not only stabilizes crRNA but also aids in recruiting Cas proteins, enhancing the overall efficiency of the CRISPR mechanism. The ability of tracrRNA to interact seamlessly with crRNA and Cas proteins solidifies its status as an indispensable element of the CRISPR system. While this collaboration has myriad benefits, including greater editing accuracy, there is also the caution of potential complications that arise from the additional layer of RNA, which can introduce variability in the gene-editing process.
Cas Proteins
Cas9 and Its Variants
Cas9 is often singled out in discussions about CRISPR because of its prominent role as the cutting enzyme that performs the actual DNA cleavage. This protein aligns with the targeting information provided by the crRNA-tracrRNA complex and executes double-strand breaks on the DNA. Variants of Cas9 have emerged that either enhance specificity or reduce potential off-target effects. This versatility is a key benefit, giving researchers the option to optimize their gene-editing endeavors for various applications. However, each variant comes with its own specifications, and the choice of Cas9 variant can greatly influence editing outcomes.
Mechanisms of DNA Cutting
The mechanism through which Cas proteins cut DNA is straightforward yet robust. Once the targeting sequence is confirmed through the crRNA, Cas9 proceeds to induce a double-strand break in the DNA, a critical step that paves the way for repair. At this point, cellular repair mechanisms kick in, and the science behind gene editing truly unfolds. While effective, these cutting mechanisms invite risks; if not well-managed, they can lead to insertion or deletion errors during the repair process. Understanding the nuances of these mechanisms is essential for anyone considering practical applications of CRISPR technology.
Mechanisms of CRISPR Action
Understanding the mechanisms behind CRISPR technology is critical to fully grasp how it revolutionizes gene editing. Delving into these mechanisms unveils the intricate interplay between the system's components. It underscores the potential of targeted modifications in genetic material, leading to significant advancements across various fields like medicine, agriculture, and beyond. A well-rounded comprehension of these actions is crucial as it helps us appreciate both the power and the limitations of CRISPR technology.
The Role of crRNA in Target Recognition
CRISPR RNA, or crRNA, plays a pivotal role in the recognition of target DNA sequences in this sophisticated system. Each crRNA sequence is derived from the viral DNA that an organism has previously encountered, acting like a genetic memory. This arm of the CRISPR system enables precise targeting of foreign genetic material, facilitating an immune response against invaders.
The crRNA guides the Cas proteins to their target, ensuring that modifications happen at the intended site. The specificity afforded by crRNA is one of the reasons CRISPR is considered a game-changer. This level of accuracy has transformed the landscape of genetic engineering, allowing scientists to manipulate genetic sequences with unprecedented precision. By understanding how crRNA operates within the CRISPR framework, researchers can also explore new ways to enhance its targeting capabilities.
The Process of Gene Editing
Target DNA Binding
Target DNA binding is a fundamental aspect of the gene-editing process via CRISPR. In straightforward terms, this phase is about how the CRISPR machinery identifies the DNA sequence that needs editing. The guiding crRNA binds to its complementary DNA strand, forming a complex known as the RNA-DNA heteroduplex.
One notable feature of this step is that it ensures high specificity. The targeted matching sequence dictates the accuracy of the entire editing process, minimizing unintended changes elsewhere in the genome. This precision is what makes CRISPR appealing for therapeutic interventions where every base pair counts.
However, it’s not devoid of challenges. The efficiency of target binding can vary depending on the DNA sequence's context and the chromatin structure. This variability means that while target DNA binding is essential, there can be limitations in how reliably this process works in every scenario.
DNA Cleavage and Repair Mechanisms
Once the target DNA is identified and bound, the next step in the gene editing process is cleavage. Cas proteins, particularly Cas9, make precise cuts in the DNA backbone at predetermined sites. This act of 'molecular scissors' is fundamental to achieving the desired genetic modification.

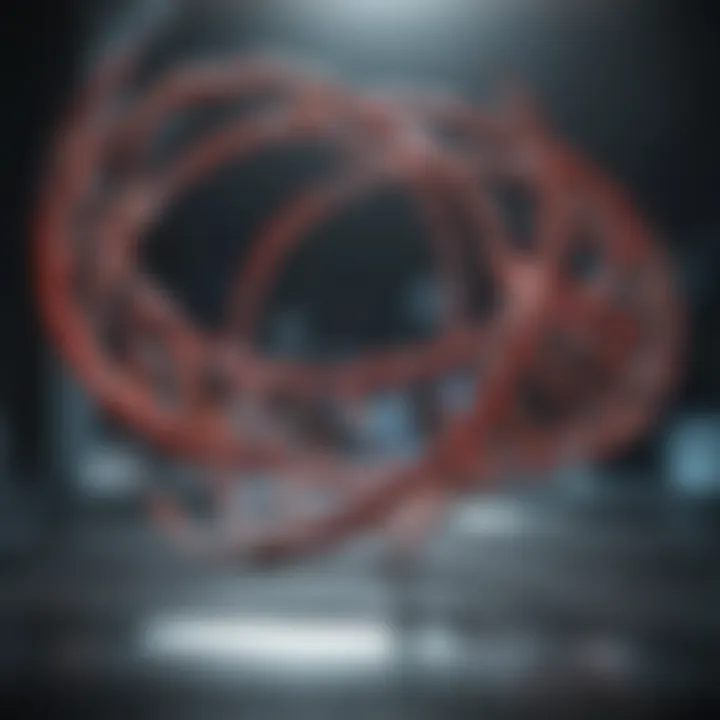
One of the key characteristics of DNA cleavage is its ability to induce double-strand breaks. These breaks are crucial because they trigger the cell's natural repair mechanisms. Two primary repair pathways can fix these breaks: non-homologous end joining (NHEJ) and homology-directed repair (HDR).
- Non-homologous End Joining (NHEJ): This is a quicker, albeit error-prone pathway. It can lead to insertions or deletions, which may alter gene function but can also be utilized effectively for gene disruption.
- Homology-Directed Repair (HDR): This repair path is more precise and can be harnessed for accurately inserting new genetic information, provided the correct donor DNA template is present.
The unique feature of these mechanisms lies in their flexibility. Depending on how researchers choose to approach gene editing, they can either leverage the error-prone nature of NHEJ for knockout strategies or aim for HDR when precise insertions are desired.
Delivery Methods for CRISPR Components
With the fundamentals of CRISPR mechanics laid out, the next significant aspect to examine is how these components are delivered into cells effectively. As innovative as CRISPR technology is, it is only as good as its methods of delivery. Various techniques, such as viral vectors, liposomes, or even electroporation, are employed to transport the CRISPR system into target cells. Each method has its pros and cons, influencing the efficacy and safety of the editing process. Understanding these methods is vital for optimizing CRISPR applications in research or clinical settings.
Applications of CRISPR Technology
The significance of CRISPR technology in contemporary biosciences cannot be overstated. It operates at the forefront of genetic engineering, enabling precise alterations to an organism's DNA. The applications span across various fields, including agriculture, medicine, and industrial biotechnology. Each field leverages CRISPR's capabilities to tackle unique challenges, thereby demonstrating its multifaceted utility.
Agricultural Advances
In agriculture, CRISPR has emerged as a game-changer. Farmers face pressing issues like climate change and food security—challenges that demand innovative solutions. With CRISPR, scientists can develop crops that not only yield more but are also resistant to pests and diseases. This means less reliance on pesticides, touching on both economic and environmental benefits. For instance, CRISPR-modified rice varieties are being created to tolerate flooding, which is a real boon for farmers in areas prone to seasonal inundation.
Additionally, using CRISPR technology could speed up the traditionally slow process of crop breeding. Genetic modifications can occur in much less time, allowing for quicker responses to market demands. However, there are concerns regarding the long-term effects on biodiversity and ecosystem balance due to the promotion of monocultures. Thus, while the innovation is appealing, it raises valid questions about sustainability.
Medical Therapeutics
The realm of medical therapeutics holds perhaps the most profound potential for CRISPR's application, particularly in the fields of cancer treatment and genetic disorders.
Cancer Treatment
Cancer treatment using CRISPR is an exciting and ever-evolving area. Researchers are exploring how to leverage this technology to customize therapies based on an individual's genetic makeup. One significant aspect is the ability of CRISPR to precisely target and edit mutations that lead to cancer. For instance, when scientists use CRISPR to remove specific oncogenes—genes that can transform a normal cell into a cancerous one—they target the heart of the disease at its molecular level.
The key characteristic of this approach is its precision. Unlike traditional treatments, which often come with broad side effects due to their indiscriminate nature, CRISPR can be fine-tuned to affect only the cells exhibiting cancerous traits. This refined targeting not only heightens efficiency but also reduces collateral damage—an attractive feature in oncology.
However, the technology isn't without its challenges. Ethical considerations in human trials are paramount, and potential off-target effects pose significant risks that need thorough examination. Despite these concerns, the prospect of personalized treatment through CRISPR presents a revolutionary shift in addressing cancer, important enough to warrant ongoing research and investment.
Genetic Disorders
The utilization of CRISPR in addressing genetic disorders is another critical aspect. Genetic disorders arise from mutations in genes, which can lead to various health issues. CRISPR provides a method to rectify these mutations. For example, diseases like cystic fibrosis or sickle cell anemia could potentially see groundbreaking therapies derived from CRISPR research.
The standout feature here is the potential for not just treating symptoms but actually curing conditions at their roots. This proactive approach to health is a significant advancement from conventional therapies that primarily manage symptoms without addressing underlying causes.
Nonetheless, the ethical implications are significant. Genetic editing raises questions about the future of human genetics and the concept of 'designer babies.' As scientists navigate these ethical waters, balancing innovation with responsibility is a notable hurdle.
Industrial Biotechnology
In industrial biotechnology, CRISPR plays a transformative role as a tool for engineering microbial strains. This engineering allows for the production of biofuels, biodegradable plastics, and even pharmaceuticals more efficiently. By fine-tuning metabolic pathways in microorganisms, companies can enhance yields and reduce costs.
Moreover, CRISPR's ability to edit genomes rapidly means that innovation cycles get shorter. Industries can adapt to changing market demands with improved agility. However, the long-term consequences of these genetically modified organisms in industrial applications remain to be seen. The intersection with natural ecosystems and other industries presents layers of complexity that require careful consideration.
In summary, the applications of CRISPR technology span a broad spectrum with implications that extend to society, economy, and ethics. The advantages it offers are substantial, yet they compel a conversation about the responsible use of such powerful technology.
Ethical Considerations in CRISPR Research
The exploration of CRISPR technology brings with it a treasure trove of possibilities but also a thicket of ethical considerations that cannot be ignored. The necessity for an ethical framework in CRISPR research is paramount, especially given its potential to drastically alter living organisms, including humans. Ethical considerations shape public perception, influence regulatory policies, and often dictate the pace of technological advancement. Understanding the intricate interplay of potential risks and benefits aids researchers, educators, and students in navigating these uncharted waters effectively.
Potential Risks and Benefits
CRISPR's prowess in gene-editing unveils a spectrum of potential risks and benefits that merit careful evaluation.
- Potential Benefits:
- Potential Risks:
- Medical Advances: CRISPR provides the ability to target genetic disorders at their root, enabling therapies that could eliminate diseases like Sickle Cell Anemia or Cystic Fibrosis.
- Agricultural Enhancement: CRISPR can create crops that are more resistant to pests or diseases, which could significantly increase food security amidst climate change challenges.
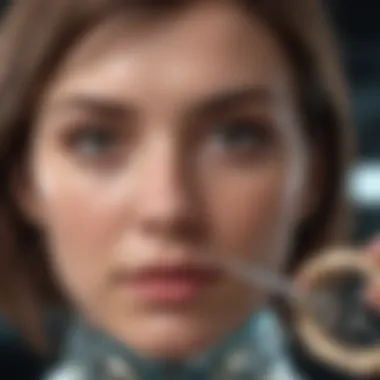
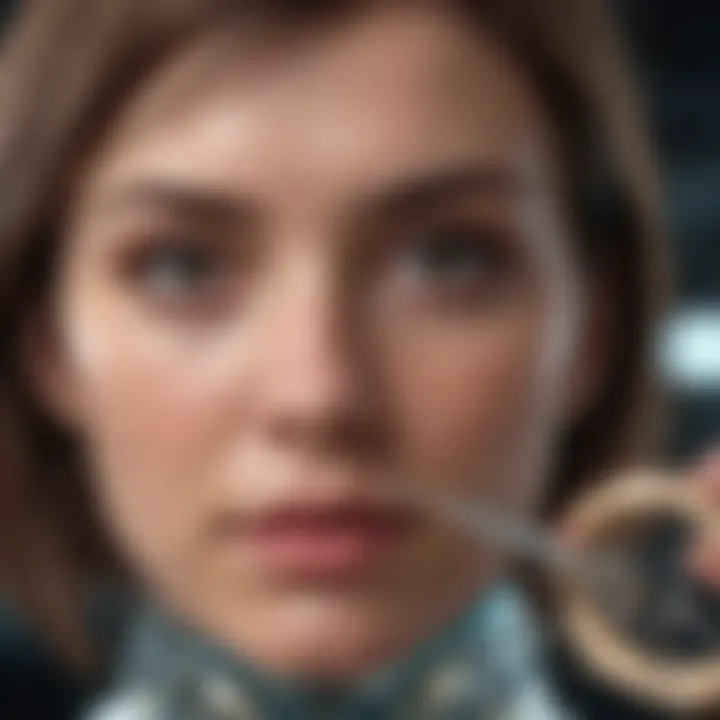
- Off-Target Effects: One pressing concern is the risk of unintended gene alterations. These unplanned changes may lead to harmful genetic consequences, disrupting entire biological systems.
- Ethical Dilemmas in Human Editing: Editing human embryos or germline cells opens a Pandora's box. Concerns arise about 'designer babies', leading to social implications that could escalate inequalities.
"With great power, comes great responsibility"—a phrase that resonates deeply in the context of CRISPR technology.
Regulatory Frameworks
The trajectory of CRISPR research is influenced heavily by regulatory frameworks which are still evolving.
- Current Regulatory Landscape: Institutions like the U.S. FDA and the European Medicines Agency oversee clinical trials. These bodies provide guidance to ensure that gene-editing technologies are used safely and ethically.
- Adapting Regulations: As scientific understanding grows, regulations must adapt. New policies will need to evolve to address the nuances of CRISPR technology, especially concerning human genetic editing and synthetic biology.
- International Agreements: Collaborations and agreements across nations must be enforced to create a cohesive ethical stance on CRISPR usage globally. The complexity of genetic technologies necessitates a unified approach to prevent misuse.
Public Perception and Acceptance
How the general public views CRISPR technology is integral to its acceptance and future development.
- Educational Outreach: Increasing awareness and understanding about CRISPR can demystify the technology. Educational campaigns and transparent communication about its risks and benefits can foster informed public opinion.
- Fear of the Unknown: Misinformation can stir fear. It's crucial for scientific communities and stakeholders to engage in dialogue and address concerns realistically. Examples include the alarmist narratives surrounding cloning and other biotechnologies that previously met with public backlash.
- Engaging Stakeholders: The role of community feedback must not be underestimated. Engaging various stakeholders—from ethicists to patients alike—will ensure that a diverse set of perspectives inform and guide decision-making in CRISPR research.
In summation, the ethical implications of CRISPR technology are vast and intertwined with its development. As scientists push boundaries, the dialogue surrounding ethics must keep pace. Addressing potentially harmful consequences while amplifying benefits will sculpt the future of CRISPR, laying the groundwork not only for scientific advancement but for societal acceptance.
Future Perspectives on CRISPR and crRNA
The trajectory of CRISPR and its components, particularly CRISPR RNA (crRNA), holds promise for transforming diverse fields ranging from medicine to environmental science. This future shines brightly with the evolution of gene editing technologies, presenting an exciting amalgamation of possibilities and challenges. As scientific communities push the envelope, understanding how these advanced tools integrate with societal norms and ethical standards becomes imperative.
Emerging Technologies
Next-Generation Genome Editing Tools
Emerging genome editing tools are stepping up the game in this space. Technologies like prime editing and base editing are redefining precision in targeting genetic sequences. These next-gen tools allow scientists to make targeted changes with heightened accuracy, limiting off-target effects that often haunted earlier CRISPR methodologies. What sets these tools apart is their ability to modify DNA without necessitating double-strand breaks, a common characteristic of traditional CRISPR approaches.
This precision is particularly beneficial when addressing complex diseases where mutations might be scattered across the genome. Moreover, their tug at favor comes from requiring less regulatory oversight compared to conventional CRISPR systems, thus streamlining their incorporation into research settings.
Integration with Other Technologies
The fusion of CRISPR with other technologies indicates an exciting frontier. For instance, the combination of CRISPR with machine learning is opening avenues for predictive analytics in genetic modification. This integration relies on algorithms to analyze vast datasets, predicting the outcomes of specific edits before making them.
What distinguishes this integration is not just enhanced prediction, but also accelerated pace in research and development of therapies. However, this does not come without its own set of challenges; merging technologies can create a labyrinth of ethical considerations particularly surrounding data privacy and manipulation.
Ongoing Research Directions
Very much on the scientific radar is ongoing research that probes not only into enhancing CRISPR functionality but also into understanding the biological implications of such interventions. Fields like synthetic biology are leveraging CRISPR's newfound capabilities to create custom organisms designed for specific tasks, like biodegradation of waste. Recent explorations delve into epigenome editing, a frontier that may hold keys to treating previously incurable genetic disorders without altering the underlying DNA. Insights from these paths can significantly shape therapeutic strategies and environmental technologies.
The Role of Public Engagement in Future Developments
As CRISPR technology continues to evolve, the public must be included in discourse surrounding its applications. The science community should actively work towards demystifying the technology for laypeople, ensuring that public sentiment aligns with scientific progress. Public engagement can encompass community workshops, accessible educational materials, or interactive forums where concerns and questions about genetic modifications can be discussed openly. This participation not only fosters trust but also helps in establishing a balanced perspective amidst innovations.
Ultimately, the future of CRISPR and crRNA is not solely in the hands of researchers. It requires a collective effort involving ethical pillars, societal understanding, and scientific advancement working in concert.
Epilogue
In the realm of genetic engineering, understanding CRISPR and crRNA transcends mere academic curiosity; it forms the backbone of numerous innovative advancements in science and technology. This article encapsulates the pivotal role of these components, shedding light on their remarkable capabilities in gene editing and the transformative consequences they entail across various sectors. As we wade through the complexities of CRISPR technology, it becomes increasingly clear that it offers a robust toolkit for addressing some of humanity's pressing challenges, from eradicating genetic disorders to enhancing agricultural resilience.
Summary of Key Insights
The journey into the nuances of CRISPR has unveiled crucial insights:
- CRISPR systems, particularly crRNA, are integral for precise gene targeting, highlighting a sophistication in biological design.
- The mechanism of action, involving binding and cleavage, illustrates a dynamic interplay between components that facilitates effective genome editing.
- The ethical dimensions surrounding CRISPR use are multifaceted, mandating a dialogue that consider potential risks alongside the undeniable benefits.
Each of these points not only highlights the advancements we’ve made but also underscores the vast potential yet to be realized.
Call to Action for Further Research
As the field of genetic editing continues to advance, further research is not just encouraged, it is essential. There remain many uncharted territories, particularly in adapting CRISPR-based technologies for therapeutic use in humans. Here are several avenues for future investigation that merit attention:
- Exploring off-target effects remains critical. Understanding these implications could enhance the safety and efficacy of CRISPR applications.
- Developing refined delivery methods for CRISPR components will increase accessibility for various applications, influencing fields from healthcare to industrial biotech.
- Engaging with the public about gene editing's ethical considerations will foster a well-rounded dialogue, ensuring that advancements align with societal values.
By committing to these research efforts, we can ensure that the evolution of CRISPR technology aligns harmoniously with human benefit, striking a balance between innovation and ethical stewardship.