Culturing Mesenchymal Stem Cells: Techniques and Applications
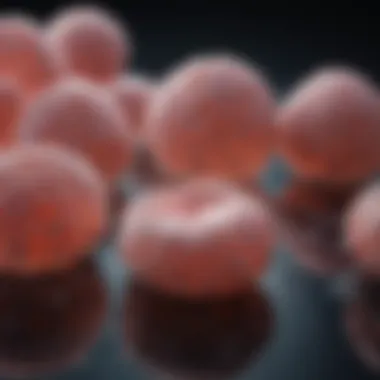
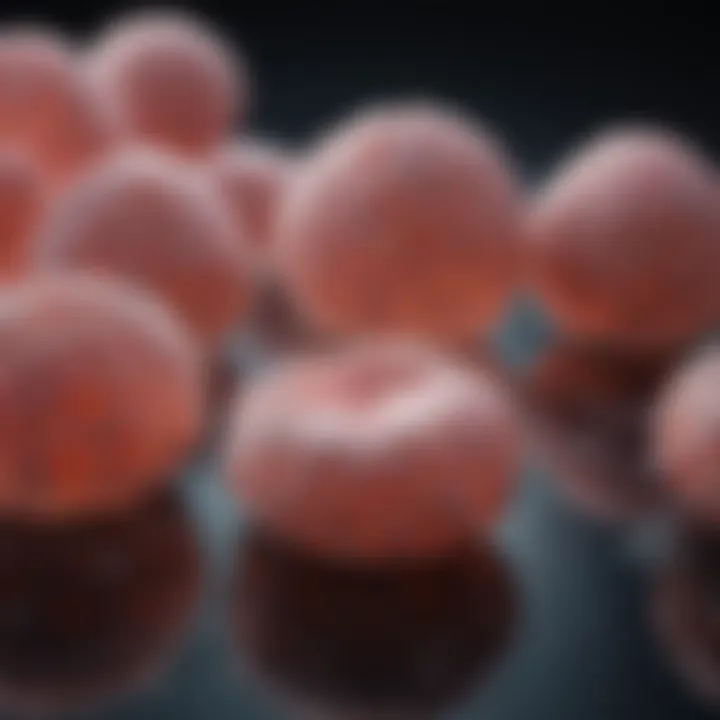
Intro
Mesenchymal stem cells (MSCs) have grabbed the attention of biomedical researchers and practitioners in recent years. They are celebrated for their unique ability to differentiate into various cell types, making them key players in regenerative medicine and tissue engineering. As we delve into the nuances of culturing these cells, it's crucial to understand the techniques, challenges, and applications that define this burgeoning field.
By studying sources such as adipose tissue and bone marrow, scientists unlock the potential of MSCs for healing and restoration. Their ability to proliferate under specific conditions opens up a world of possibilities, from treating degenerative diseases to facilitating complex tissue repair. This article serves as a guide, highlighting the methods employed in culturing MSCs, the vital factors influencing their growth, and the exciting future that lies in their clinical applications.
Research Overview
Summary of Key Findings
The exploration into MSC culturing unveils several key findings. It is evident that the source of the stem cells plays a significant role in their properties and behavior. Adipose-derived stem cells (ADSCs) typically demonstrate higher proliferation rates compared to those derived from bone marrow, yet the latter may offer greater longevity in culture. Furthermore, environmental conditions, including temperature, oxygen levels, and growth factors, prove pivotal in guiding cell maturation and differentiation.
Importance of the Research in Its Respective Field
The research on MSCs cultivations, as this article elucidates, is crucial for several reasons. Firstly, understanding the intricacies of MSC culture enhances our capabilities in regenerative medicine, paving the way for innovative therapies. Secondly, this body of work aids in bridging gaps in knowledge that limit the effectiveness of existing clinical applications. Ultimately, these insights contribute to improved patient outcomes, fulfilling the promises of modern medicine.
Methodology
Description of the Experimental or Analytical Methods Used
In culturing MSCs, techniques vary greatly, yet the overarching methods incorporate standard protocols involving cell isolation, expansion, and differentiation. Typically, isolation involves:
- Adipose Tissue Procurement: For ADSCs, liposuction techniques are employed.
- Bone Marrow Aspiration: This involves extracting marrow from the iliac crest or other sites.
- Cell Separation: Following isolation, centrifugation is often used to separate the desired cell type from unwanted material.
Once isolated, cells undergo a series of steps to promote optimal growth. For instance, stem cells are routinely grown in specialized media enriched with growth factors such as fibroblast growth factor (FGF) or platelet-derived growth factor (PDGF).
Sampling Criteria and Data Collection Techniques
Sampling plays a vital role in ensuring the viability and applicability of the cultured MSCs. Researchers typically define criteria to ensure high-quality samples, which often involve the age or health status of the donor as well as controlled environments during cell collection. Data collection methods, too, have evolved with technology. Transcriptomic and proteomic analyses facilitate better understanding of cell behavior, offering insights into how MSCs respond to varying culture conditions.
"Knowledge of the culturing process not only improves experimental outcomes but also lays the groundwork for future innovations in therapies using MSCs."
In essence, as this narrative unfolds, the sophisticated dance of culturing mesenchymal stem cells becomes increasingly clear. It’s a blend of art and science, requiring attention to detail, ingenuity, and a forward-thinking approach to harness the promise of these remarkable cells.
Preface to Mesenchymal Stem Cells
Understanding mesenchymal stem cells (MSCs) is like piecing together a jigsaw puzzle of regenerative medicine. These cells hold immense potential in the realm of tissue engineering and therapy. They can differentiate into various cell types, making them valuable for researching treatments for numerous conditions, such as ischemic diseases, spinal cord injuries, and degenerative diseases. This section explores the significance of MSCs, covering their definition, key characteristics, and the historical backdrop that has shaped our current comprehension of these fascinating entities.
Definition and Characteristics of MSCs
Mesenchymal stem cells are multipotent stem cells, typically derived from various tissues, including bone marrow, adipose tissue, and umbilical cord. These cells are known for their self-renewing ability, which allows them to produce identical copies over time. Additionally, MSCs are marked by their capacity to differentiate into specialized cell types, such as adipocytes, osteoblasts, and chondrocytes.
A hallmark of MSCs is their plasticity, or the ability to adapt and change according to their environment. They can respond to various signaling molecules, which influences their fate in the body. In the petri dish, it is crucial to provide the right conditions for these cells to thrive and fulfill their potential. This is why a detailed understanding of the conditions necessary for their growth is invaluable for researchers and practitioners.
Historical Context of MSC Research
The journey of MSC research commenced in the late 20th century, though the first mentions of multipotent stem cells date back even earlier. In 1970, researchers discovered a rare population of stem cells within the bone marrow that could turn into different cell types. This flared interest, which has only gained momentum over the decades, leading to a flurry of studies and applications.
In the early 2000s, MSCs were increasingly recognized for their immunomodulatory properties, which opened doors for therapeutic avenues in autoimmune diseases and organ transplantation. As the field evolved, so did the techniques for isolating and culturing these cells,making it easier for scientists to work with MSCs and understand their mechanisms.
Recent discoveries have not only validated the promise these cells hold but have also unveiled the complexities that come with their use, including challenges in cell therapy applications and nuances in their behavior under various culture conditions. The convergence of biology, engineering, and clinical science around MSCs has led to an intensified quest for innovative approaches in regenerative medicine.
Understanding the intricacies of mesenchymal stem cells shapes future strategies in regenerative therapies, helping us navigate the maze of cellular behavior within the human body.
In summary, this section lays the groundwork for a deeper exploration into how we can leverage MSCs for medical advances. Understanding their definition, growth conditions, and historical significance sets the stage for unraveling the complexities of culturing these cells, which will be discussed in the following sections.
Sources of Mesenchymal Stem Cells
Understanding the sources of mesenchymal stem cells (MSCs) is vital in the realm of regenerative medicine and cell therapy. The choice of source influences not only the quality and quantity of the MSCs harvested but also their differentiation potential, immunogenicity, and therapeutic efficacy. Each MSC source comes with its own set of advantages and challenges, dictating the direction of their applications in clinical settings. Grasping the nuances of these sources can pave the way for improved outcomes in tissue engineering and regenerative practices.
Classification of MSC Sources
Mesenchymal stem cells can be derived from various tissues in the human body, each presenting unique characteristics and applications:
- Adult MSCs: Found in postnatal tissues such as bone marrow, adipose tissue, and dental pulp.
- Embryonic MSCs: Sourced from the inner cell mass of a blastocyst, presenting high pluripotent capabilities but also facing ethical considerations.
- Perinatal MSCs: Located in umbilical cord tissue, amniotic fluid, and placental tissue, these cells exhibit regenerative properties and lower ethical concerns.
Each type of MSC source can fulfill specific roles in research and therapeutic applications, making this classification foundational in understanding their potential impact.
Adipose-Derived MSCs: Advantages and Limitations
Adipose-derived stem cells (ADSCs) are gaining traction due to the ease of access and relative abundance found in adipose tissue. Their extraction through liposuction is minimally invasive, making them appealing for both patients and practitioners.
Advantages:
- Abundance: High yield of stem cells can be obtained in a single procedure.
- Rapid Expansion: ADSCs have a quicker proliferation rate compared to bone marrow-derived MSCs.
- Multilineage Differentiation Potential: Capable of differentiating into a variety of cell types, such as adipocytes, chondrocytes, and even osteocytes.
Limitations:
- Variability: Their properties can vary according to the individual’s metabolic state and age.
- Less Characterized: Compared to bone marrow MSCs, less is known about their long-term behavior and safety in clinical applications.
Bone Marrow-Derived MSCs: A Comparative Analysis
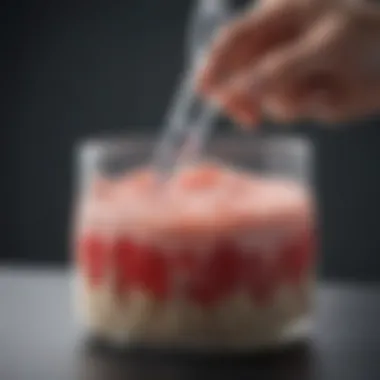
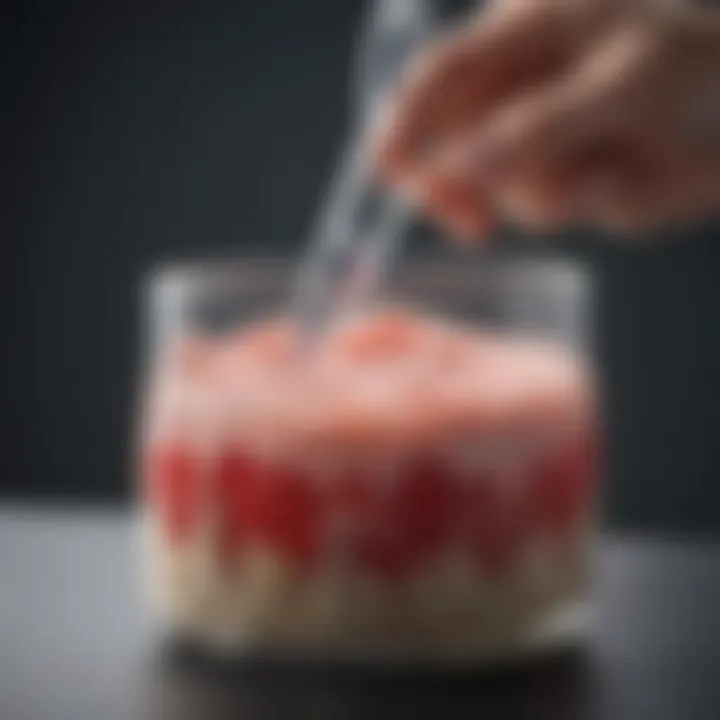
Bone marrow-derived MSCs (BM-MSCs) have long been the gold standard in cell therapy due to their extensive research backing and application history. Historically, they have been widely utilized in conditions like hematological malignancies and bone regeneration.
Pros of BM-MSCs:
- Well-Characterized: A large body of literature provides insights into their biology and applications.
- Strong Differentiation Capacity: They possess robust potential to differentiate into various cell types, particularly osteogenic lineages.
Cons of BM-MSCs:
- Invasive Harvesting: The procedure to extract cells involves bone puncture, which may raise discomfort and risks for the patient.
- Age-Related Decline: Stem cell viability and regenerative capacity decrease with age, impacting efficacy.
When comparing ADSCs to BM-MSCs, the debate often hinges on ease of access versus the quantity and quality of outcomes.
Other Sources: Dental Tissue and Umbilical Cord
Emerging sources of MSCs such as dental tissue and umbilical cord have started to draw attention due to their regenerative potential and lower ethical hurdles.
Dental Tissue-Derived MSCs:
- Highly multipotent, with the ability to differentiate into neurogenic and odontogenic lineages.
- Harvested from teeth that are naturally lost or extracted, presenting a sustainable option.
Umbilical Cord-Derived MSCs:
- Non-invasive to both mother and child, making them ethically appealing.
- Exhibits a high proliferation rate and exhibits immunomodulatory properties, which could be beneficial in treating autoimmune disorders.
Both dental and umbilical cord sources allow for innovative research avenues, potentially shaping the landscape of regenerative therapies.
"The journey of MSC research is thrilling, revealing new frontiers beyond what was once thought possible for regenerative therapies."
Understanding these diverse sources equips researchers and clinicians with the insights needed to make informed choices in cell-based therapies, ultimately enhancing the journey towards effective treatments.
Isolation Techniques for MSCs
The process of isolating mesenchymal stem cells (MSCs) is a crucial step in ensuring the success of downstream applications, especially in research and regenerative medicine. The techniques used can significantly affect the quality, yield, and functionality of the isolated cells. A deep understanding of isolation techniques not only facilitates efficient MSC acquisition but also addresses challenges in contamination, viability, and cell characteristics. This section explores various methods used to isolate MSCs, highlighting their importance, advantages, and considerations.
Enzymatic Digestion Methods
Enzymatic digestion is one of the most common methods used to isolate MSCs from tissues such as bone marrow or adipose tissue. This technique typically employs enzymes like collagenase, trypsin, and hyaluronidase to break down the extracellular matrix, releasing the stem cells into suspension.
Key benefits of enzymatic digestion include:
- High Efficiency: This method can extract a large quantity of MSCs, particularly from dense tissues, with relative ease.
- Controlled Environment: With careful optimization, it is possible to minimize cellular damage, maintaining the viability of cells.
- Versatile: Various types of tissues can be processed using different enzyme combinations, making it adaptable to research needs.
However, there are several considerations:
- Enzyme Concentration: Overexposure to enzymes can lead to the degradation of cell surface markers and loss of stemness, which is vital for subsequent applications.
- Standardization: Variability in enzyme sources and preparations may yield inconsistent results.
In high-stakes applications where precision is king, these factors necessitate meticulous protocol development and rigorous validation.
Adherence-Based Methods
Another common approach for isolating MSCs is via adherence-based techniques. This method leverages the unique property of MSCs to adhere preferentially to culture dishes. Initially, a mixed cell population is seeded onto a cell culture dish, often coated with fibronectin or collagen to enhance cell attachment.
Some advantages here include:
- Simplicity: The technique is straightforward and does not require advanced skills or specialized equipment.
- Purity: Non-adherent cells can be removed through washing, leading to a highly enriched MSC population.
- Low Cost: It is usually more economical compared to enzymatic alternatives, particularly in resource-restricted settings.
Nevertheless, adherent isolation has its challenges:
- Time-Consuming: The selection process can take several days, delaying further experimentation.
- Cell Stress: Cells may undergo stress during the initial attachment period, which can affect their survival and functionality.
This method is particularly effective in settings where other cell types are present, but diligence is needed to maintain cell health during culture.
Fluorescence-Activated Cell Sorting (FACS)
Fluorescence-activated cell sorting, often known as FACS, represents a more advanced isolation technique that harnesses the power of flow cytometry to separate MSCs based on their surface markers. Cells are tagged with fluorescent antibodies corresponding to specific MSC surface antigens, allowing for precise identification and sorting.
Highlights of using FACS include:
- Accuracy: This method allows for the isolation of highly pure MSC populations, as cells can be sorted based on the expression of multiple surface markers.
- Real-Time Analysis: FACS provides immediate feedback about the cell populations during isolation, allowing for on-the-fly adjustments.
- Flexibility: Researchers can customize sorting parameters according to specific project needs, targeting a broad array of surface markers.
The drawbacks of FACS, however, should be noted:
- Equipment Needs: FACS requires expensive flow cytometers and proper training to operate effectively.
- Cell Viability: The sorting process can induce stress and may affect the viability of sensitive cell types if not carefully managed.
In summary, the choice of isolation technique can influence the subsequent applications of MSCs. Whether to opt for the efficiency of enzymatic digestion, the simplicity of adherence-based methods, or the precision offered by FACS ultimately rests on the specific research goals, available resources, and the desired characteristics of the isolated cell populations.
By understanding these different techniques, researchers can optimize MSC isolation protocols to suit their individual needs, enhancing the quality and applicability of their work.
For further insights on isolation techniques and MSC biology, check out these resources: Wikipedia on Stem Cells, Britannica Overview.
Culturing Techniques for MSCs
Culturing techniques for mesenchymal stem cells (MSCs) play a pivotal role in their utility across various fields, particularly in regenerative medicine and tissue engineering. The crux of successful MSC application lies in how well these cells are cultivated, as the conditions of their culture significantly influence their behavior, proliferation, and differentiation. Establishing optimal culturing techniques is not merely a laboratory preference; it’s an essential step that dictates the outcomes for subsequent applications in clinical settings. In essence, good culture practices not only enhance the yield of MSCs but also preserve their fundamental characteristics that make them priceless in therapeutic situations.
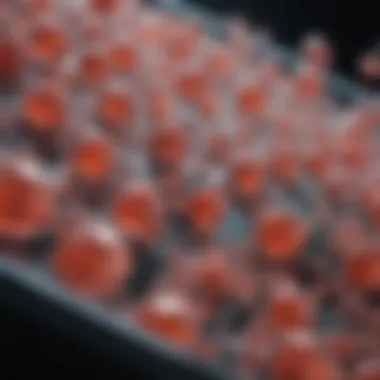
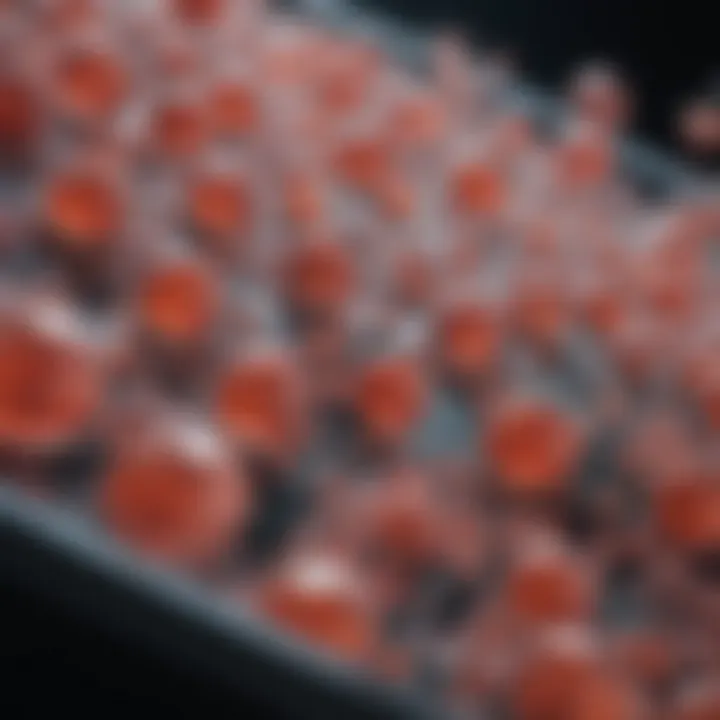
Basic Culture Conditions
Basic culture conditions serve as the foundation upon which MSCs thrive. Typically, these conditions involve the choice of a suitable growth medium, with Dulbecco's Modified Eagle Medium (DMEM) being commonly favored. The medium needs to be supplemented with fetal bovine serum (FBS) to provide essential nutrients and hormones that encourage cell growth. Temperature control is another essential detail; incubating MSCs at 37 degrees Celsius mimics the human body's environment, ensuring that the cells remain viable and functional.
Moreover, maintaining a humid atmosphere with 5-10% CO2 helps to stabilize pH levels and further supports the growing cells. It's also crucial to monitor the density of the cells: overcrowding can lead to cellular stress and unexpected differentiation. In the end, a delicate balance exists between nurturing the cells and allowing them space to proliferate.
Use of Growth Factors and Cytokines
In the pursuit of enhancing MSC cultures, the inclusion of specific growth factors and cytokines cannot be overlooked. For instance, basic fibroblast growth factor (bFGF) and epidermal growth factor (EGF) come to mind, both of which promote proliferation and maintain the stem-like properties of MSCs. These bioactive molecules are not merely additives but act as essential players in regulating cellular responses.
Furthermore, coupling these factors with appropriate concentrations can lead to improved outcomes. The intricate dance of signaling pathways activated by these factors can either hinder or stimulate the cells’ differentiation pathways. It's a nuanced approach that, when executed correctly, can fine-tune the MSCs' future capabilities.
- Benefits of using growth factors:
- Enhanced proliferation
- Protection of stemness traits
- Guiding differentiation towards desired lineages
Influence of Extracellular Matrix Components
The extracellular matrix (ECM) components represent the native environment which plays a crucial role in guiding MSC behavior. Various ECM proteins, such as collagen and fibronectin, have a substantial influence on how MSCs adhere, migrate, and differentiate. The question then, is not merely what you culture your MSCs in, but how you modify that ‘home’ to reflect the body's natural tissue architectures.
The presence of various ECM elements can also modulate the effects of growth factors, creating a synergistic relationship that enhances outcomes. For example, integrating hydrogels infused with ECM proteins can provide a spatial structure that promotes better cell attachment and function. As a result, researchers and practitioners must consider the ECM as an active participant in the culture environment.
"The extracellular matrix is not just a structural component but a vital player in MSC biology."
Advanced Culture Techniques
Advanced culture techniques for mesenchymal stem cells (MSCs) are pivotal in elevating our understanding and application of these cells in various fields, particularly regenerative medicine. These methodologies are designed to mimic the natural environment of the cells more closely, fostering improved growth, functionality, and differentiation potential. By leveraging sophisticated systems like 3D cultures, bioreactors, and co-culture systems, researchers can create conditions that not only enhance cell yields but also preserve the intrinsic characteristics of MSCs.
3D Culture Systems
Three-dimensional culture systems are increasingly heralded for their ability to provide a more physiologically relevant environment for MSCs as compared to traditional two-dimensional techniques. In a natural setting, cells exist in a multi-layered organization within tissues, where they interact not only with neighboring cells but also with extracellular matrix (ECM) components. 3D cultures utilize scaffolds made from various biomaterials that support cell attachment and proliferation, offering several benefits:
- Enhanced Cell-Cell Interactions: This model allows MSCs to communicate more effectively, potentially leading to improved functionality.
- Improved Differentiation: MSCs in 3D environments often display enhanced differentiation capabilities into desired lineages, such as osteoblasts or chondrocytes.
- Mimicking Physiological Responses: The conditions replicate those found in vivo, allowing researchers to observe more accurate responses to stimuli or drugs.
While creating these systems requires a deeper know-how regarding the choice of materials and design, the resultant products can be indispensable in developing advanced therapeutic applications.
Bioreactor Applications for MSC Expansion
Bioreactors offer a controlled environment that can significantly optimize the expansion of MSCs. These devices are engineered to support cell growth and can be tailored to suit specific experimental needs. The benefits of bioreactor systems in MSC culturing include:
- Scalability: Bioreactors are capable of producing large quantities of MSCs, which is essential for clinical applications.
- Environment Control: Parameters such as pH, temperature, and nutrient levels can be finely adjusted, ensuring optimal growth conditions.
- Dynamic Culture Conditions: Some bioreactors enable the simulation of shear stress or perfusion, closely mimicking the physiological situations that cells would typically encounter in the body.
These advantages illustrate why bioreactors are becoming fundamental in cell cultivation protocols aimed at achieving better yields and quality of MSCs for therapeutic purposes.
Co-Culture Systems with Other Cell Types
Co-culture systems involve the simultaneous growth of MSCs alongside other cell types. This technique can shed light on cell behavior in a more realistic microenvironment. The dynamics of these interactions can have profound implications for their application in therapy. Key considerations in co-culture systems include:
- Cellular Communication: MSCs can influence surrounding cells through secreted factors, promoting effects like enhanced tissue regeneration or immunomodulation.
- Enhanced Functional Properties: Co-culturing MSCs with other cell types often results in improved viability and functionality of both cell types. For instance, pairing MSCs with endothelial cells can enhance vascularization of engineered tissues.
- Understanding Niche Interactions: These systems also provide valuable insights into the niche of MSCs, helping to unravel how environmental signals modulate stem cell behavior.
Implementing co-culture strategies could pave the way for breakthroughs in understanding complex cellular interactions that drive regenerative processes.
"Advanced culture techniques not only improve cell yields but also better simulate the physiological conditions under which MSCs thrive."
Challenges in MSC Cultivation
Cultivating mesenchymal stem cells (MSCs) is not all sunshine and rainbows. Though the potential applications of MSCs in areas like regenerative medicine and tissue engineering dazzle researchers, the road to successful cultivation is fraught with obstacles. Addressing the challenges that arise during the culturing process is paramount for optimizing the application of these cells in clinical settings.
Three critical elements emerge when considering the challenges faced in MSC cultivation: contamination, limited proliferation, and cell lifecycle issues. These hurdles require careful strategies to ensure the viability and efficacy of MSCs in therapeutic situations. Understanding these challenges can illuminate the path toward more effective MSC applications.
Contamination and Sterility Issues
One of the foremost concerns when culturing MSCs is maintaining sterility. Contaminants can come in various forms, including bacterial, fungal, or viral agents, each posing a significant threat to cell cultures. Sources of contamination often include the environment or improperly sterilized equipment. As contamination can compromise research results or therapeutic outcomes, strict adherence to aseptic techniques is vital.
- Strategies to Mitigate Contamination:
- Utilize biosafety cabinets during cell manipulation.
- Regularly monitor cultures for signs of contamination.
- Implement routine sterilization protocols for all culture materials.
Even with sterile techniques, contamination still remains a possibility. Implementing good laboratory practices reduces the risk but does not eliminate it completely, making awareness and vigilance critical components of successful MSC cultivation.
Limited Proliferation and Differentiation Potential
MSCs face a unique limitation concerning their growth and differentiation capabilities. As these cells age during culture, they can experience a decline in proliferative and differentiation potential. Factors such as their source, initial passage number, and microenvironment can substantially influence these characteristics. The limited ability to differentiate into specialized cells may thwart the potential therapeutic applications of MSCs.
To address these issues, researchers are often left scrambling for solutions:
- Adjusting culture conditions, such as cell density and media composition.
- Exploring the role of specific growth factors to enhance proliferation.
- Investigating alternative culture systems that support better growth and differentiation.
Each of these approaches brings its own nuances and potential pitfalls, necessitating a tailored strategy for each specific MSC source and application context.
Cell Senescence and Apoptosis
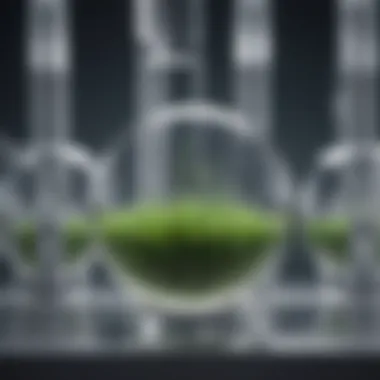
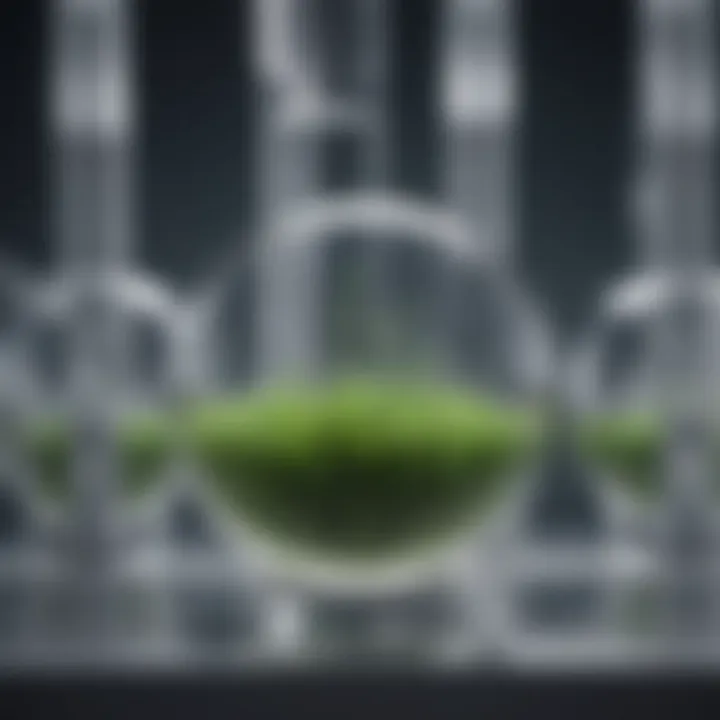
As cells get older, they tend to undergo senescence—a point in their lifecycle where they cease to divide but do not die. This state can significantly affect MSC functionality, limiting their use in regenerative therapies. Apoptosis, or programmed cell death, is another concern; it often happens in response to stressors in the environment or unfavorable culture conditions.
- Key Factors Leading to Senescence and Apoptosis:
- Long-term culture conditions without adequate nutrient sources can trigger stress responses, leading to cell death.
- The accumulation of reactive oxygen species (ROS) due to metabolic activity can accelerate cellular aging.
Both senescence and apoptosis not only limit the available pool of healthy MSCs but also can alter the properties of surviving cells, further complicating their therapeutic effectiveness.
"Keeping a step ahead of these challenges often determines the efficacy of MSC technology in clinical applications. Understanding the biology behind these cells is just as crucial as refining the methods used to cultivate them."
As researchers navigate these challenges, crafting innovative solutions must become the focal point of future MSC studies, ensuring that these remarkable cells can meet the promise of regenerative medicine.
Applications of Cultured MSCs
Cultured mesenchymal stem cells (MSCs) provide a wide range of applications that are significant in modern biomedical research and clinical therapy. Their versatility in differentiation and ability to modulate immune responses have brought MSCs to the forefront of regenerative medicine. The exploitation of MSCs for various therapeutic strategies not only highlights their importance but also suggests avenues for overcoming existing medical challenges.
Regenerative Medicine and Tissue Repair
Regenerative medicine has gained substantial traction thanks to the unique properties of MSCs. These cells can differentiate into multiple cell types, such as osteoblasts, chondrocytes, and adipocytes, which is vital for tissue repair and regeneration. With them being sourced from both adult and fetal tissues, the accessibility offers an avenue for developing treatments that could aid in healing damaged tissues.
The regenerative potential of MSCs is particularly evident in conditions such as:
- Bone fractures or non-unions where MSCs can assist in forming new bone.
- Cartilage injuries, where their chondrogenic differentiation helps regenerate cartilage tissue.
- Cardiac repair, following myocardial infarction, MSCs may contribute to heart tissue regeneration.
MSC therapy in these contexts is not simplistic. It comes with nuances regarding their administration, which often necessitates preconditioning or the addition of factors that could enhance their therapeutic efficacy. As an example, adding specific growth factors can boost their survivability post-implantation.
Role in Immunomodulation
One of the more remarkable attributes of MSCs is their ability to influence the immune system. They have immunosuppressive capabilities, making them valuable in treating autoimmune diseases and inflammatory conditions. This quality stems from their ability to interact with various immune cells, including T cells, B cells, and dendritic cells, often asserting a negative regulatory effect.
In practical applications, MSCs can
- Reduce inflammation in diseases like rheumatoid arthritis.
- Adjust immune responses in organ transplantation, potentially lowering rejection rates.
This inherent property suggests that MSCs could serve more than just a regenerative role; they could be strategic players in balancing immune homeostasis, presenting a dual role in tissue repair and immune modulation.
"The dual role of MSCs in regeneration and immunoregulation positions them as key players in the future of therapeutic strategies."
Preclinical and Clinical Trials Involving MSCs
The growing interest in MSC applications has sparked numerous studies and clinical trials aimed at validating their efficacy. These trials might involve MSCs harvested from various sources, such as bone marrow, adipose tissue, or even umbilical cord blood, exploring different treatment protocols for several diseases.
In preclinical settings, these cells have demonstrated promise in:
- Treating spinal cord injuries where applications of MSCs have led to moderate recovery in animal models.
- Improving skin healing through enhanced angiogenesis and reduced fibrosis.
As for clinical trials, findings have been varied, depending on factors such as the source of MSCs, the methods of administration, and the conditions being treated. For example, early-phase trials for MSCs in treating osteoarthritis have yielded encouraging results, often describing enhanced patient outcomes and improved function.
Nevertheless, the road to widespread acceptance of MSC therapies is riddled with challenges, including ethical considerations and the standardization of cultivation procedures. Authors in recent reviews emphasize the need for rigorous protocols in the reporting of trial results to ensure reliability.
The future of MSC applications appears promising, with ongoing trials paving the way for broader clinical implementation, which could redefine approaches to treating complex medical conditions.
Future Directions in MSC Research
The future of mesenchymal stem cell (MSC) research holds a myriad of promising paths that may not only refine current practices but also open doors to revolutionary applications in regenerative medicine, tissue engineering, and beyond. Understanding these directions is crucial for researchers and practitioners alike, as they provide insight into what methodologies could define the next wave of innovations in the field. The focus on these elements can enhance both the efficacy of MSC usage and the safety of treatments derived from these versatile cells.
Innovations in Cell Culture Technology
Current innovations in cell culture technology are reshaping how MSCs are cultivated and expanded. Traditional techniques often struggle with scalability and maintaining cell potency, which is where new technologies come into play. Examples include:
- Microfluidic Devices: These tools enable precise control of the cell environment, promoting optimal growth conditions. Their capability to manipulate small volumes of fluids allows researchers to carry out experiments with a high level of accuracy.
- Automated Cell Culture Systems: Reducing human error, these systems can improve reproducibility in MSC cultures. Automation enhances capacity and efficiency, allowing larger quantities of cells to be handled consistently.
- Bioprinting: An emerging technique that facilitates the generation of tissue-like structures using MSCs. This can be a game-changer for creating complex tissue models and for potential use in transplantation.
These advancements highlight the importance of integrating technology in cell culture, paving the way for more efficient production and application of MSCs in clinical settings.
"The harmonization of innovative technologies with traditional culturing methods is setting the stage for unprecedented breakthroughs in cell therapy."
Potential for Genetically Modified MSCs
Genetically modified MSCs present an exciting frontier in stem cell research, providing potential avenues for treatment modalities that integrate gene therapy with cellular therapy. The modification can enhance the inherent properties of MSCs, positioning them as powerful agents in diverse therapeutic contexts. Key aspects include:
- Enhanced Therapeutic Efficacy: By introducing specific genes that promote survival, migration, or differentiation, MSCs can be tailored to better address various pathological conditions.
- Targeted Delivery Systems: Genetic modifications can endow MSCs with the ability to home in on particular tissues or tumors, improving the precision of therapeutic interventions.
- Long-Term Expression of Therapeutic Agents: Genetic engineering can allow MSCs to produce therapeutic molecules in a sustained manner, potentially reducing the need for repeated treatments and enhancing patient outcomes.
As researchers continue to explore the potential of genetically modified MSCs, ethical considerations and safety protocols will be paramount. This carries the need for rigorous preclinical testing to evaluate the risks, ensuring that advances in this area are pursued responsibly and effectively.
Culmination
The concluding segment of this article on culturing mesenchymal stem cells (MSCs) holds significant value as it not only encapsulates the discussions but also points towards future trajectories in this field. Summarizing key procedures and insights on MSC culture techniques, the culmination emphasizes how critical these methodologies are to the broader landscape of regenerative medicine and tissue engineering. The importance of optimizing culture conditions, ensuring cell viability, and understanding the potential of MSCs in various applications cannot be overstated.
Summation of Key Points and Future Perspectives
As we peer into the future of MSC research, several key points stand out:
- Versatility of MSCs: The remarkable ability of MSCs to differentiate into multiple cell types positions them as pivotal players in regenerative therapy.
- Emerging Techniques: Advanced methods like 3D culture and bioreactor systems pave the way to enhanced MSC proliferation while maintaining their functional integrity.
- Integration into Clinical Practices: Continuous research presented in clinical trials is indicative of a shift towards practical applications of MSCs.
- Barriers to Overcome: Challenges such as cell senescence and growth limitations remain. Addressing these issues through innovative research methodologies is essential for the advancement of therapeutic uses.
Looking ahead, the future may well lie in developing genetically modified MSCs that can offer tailored solutions to diverse medical conditions. As researchers dive deeper into harnessing the potential of these cells, the understanding of their biology will likely incite new applications, further bridging the gap between laboratory research and clinical practice.
By focusing on innovative technological applications and overcoming existing hurdles, the realm of MSC research could fundamentally reshape approaches to healing and regeneration.
The importance of this conclusion is profound. It serves not only to synthesize the main findings but also encourages ongoing inquiry and application of the principles discussed throughout the article. The journey of culturing MSCs is far from over. Rather, it's just the beginning of a fertile landscape ripe with possibilities.