DNA Gel Electrophoresis: A Comprehensive Step-by-Step Guide
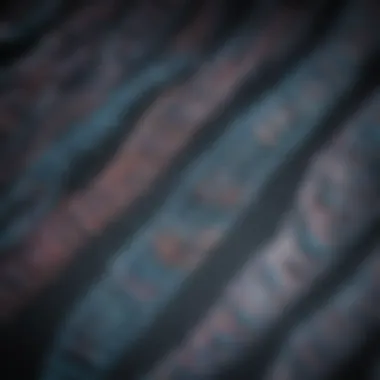
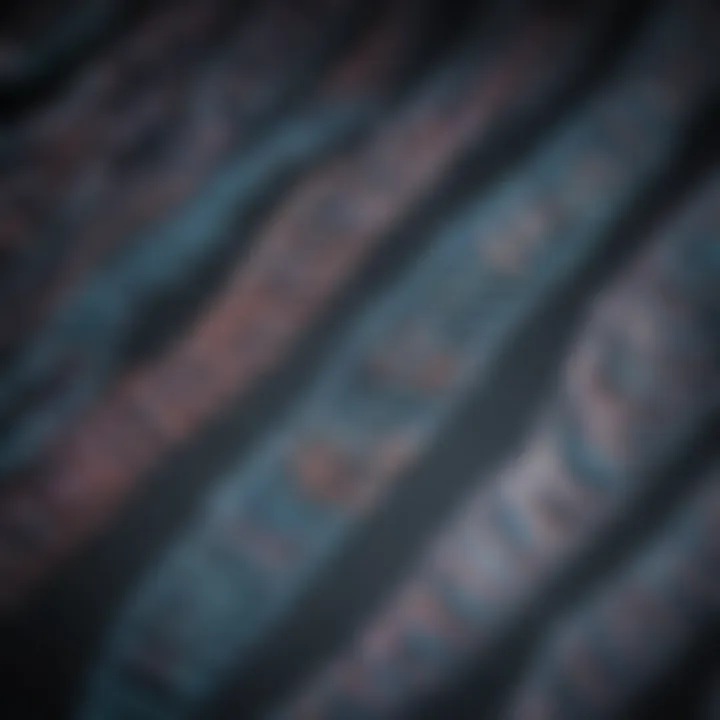
Research Overview
DNA gel electrophoresis is a cornerstone method in molecular biology, allowing researchers to explore the genetic code and uncover essential insights in various studies. The ability to separate and analyze DNA fragments based on size underpins many applications in genetics, forensics, and biotechnology. This technique serves not just as a means of analysis, but also as an invaluable tool in the advancement of modern scientific knowledge.
Summary of Key Findings
Recent studies consistently highlight the effectiveness of DNA gel electrophoresis in determining the integrity of DNA samples, especially those extracted from challenging sources such as environmental samples or ancient specimens. The method has proven indispensable in identifying genetic markers and validating PCR products, making it a go-to approach for many labs worldwide.
Importance of the Research in Its Respective Field
The significance of DNA gel electrophoresis in scientific research is difficult to overstate. Forensic scientists rely on this technique to analyze crime scene evidence, while agricultural scientists utilize it to identify genetically modified organisms. Furthermore, in clinical settings, it assists in diagnosing genetic disorders by allowing for the visualization of gene mutations. Each application underscores the inner workings of life at a molecular level, fostering advancements in health, safety, and our understanding of biological processes.
Methodology
Description of the Experimental or Analytical Methods Used
The methodology surrounding DNA gel electrophoresis is as critical as its applications. This process begins with the preparation of an agarose gel, through which samples will migrate. The gel acts as a molecular sieve, separating DNA fragments based on their size due to the application of an electric field. An array of factors influences the outcome, including gel concentration, the voltage applied, and buffer solutions used. A common practice is using ethidium bromide or other DNA stains to visualize the separated bands when viewed under UV light.
The steps typically involved are:
- Preparation of agarose gel: Different concentrations yield different resolutions, so a careful choice is needed.
- Loading samples: DNA samples mixed with loading dye are placed into the wells.
- Running the gel: The electric current facilitates the movement of DNA toward the positive electrode.
- Visualization: After the gel has run for a specified time, it is stained for observation.
Sampling Criteria and Data Collection Techniques
Sampling in DNA gel electrophoresis varies depending on the aim of the study. Researchers select samples based on specific criteria that align with their objectives, be they clinical samples, cultured cell lines, or environmental extracts. Data is often collected through controlled experiments that ensure reproducibility and allow for comparability across various studies.
"Each sample carries a story encoded in its DNA – unraveling that story requires both skill and insight."
Furthermore, documentation of results through imaging software allows for precise quantification and comparison of DNA band sizes, supporting a deeper analysis of genetic information. This meticulous attention to detail ensures that findings can be verified and built upon in future research.
The intricacies of DNA gel electrophoresis represent a fascinating intersection of techniques and technology, laying bare the fabric of life itself.
Prelude to DNA Gel Electrophoresis
DNA gel electrophoresis stands as a cornerstone technique in molecular biology, enabling the analysis and separation of nucleic acids. Understanding the mechanism of this process not only enriches one’s knowledge of genetic analysis but also highlights its applications in various fields like forensic science, genetic research, and biotechnology. The ability to visualize and interpret DNA fragments is vital for researchers, educators, and even students embarking on their scientific journey.
Through gel electrophoresis, one can achieve precise results that are essential for a range of applications from gene cloning to ancestry testing. The ease of which gel electrophoresis can be integrated into experiments strengthens its relevance in both educational and professional laboratories. Accordingly, mastering this technique can transform a novice into a proficient researcher.
Overview of Electrophoresis
Electrophoresis is a basic yet profound process that utilizes an electric field to move charged particles through a medium. In the context of DNA, the negatively charged phosphate backbone repels towards the positive electrode when subjected to an electric current. This migration is influenced significantly by the size and charge of the DNA fragments being analyzed. Smaller fragments navigate the gel matrix with greater ease than larger ones, resulting in clear separation based on size.
The gel itself, often made from agarose or polyacrylamide, serves as a sieve. The choice between these gels hinges on the desired resolution and fragment size; agarose is generally favored for larger fragments, while polyacrylamide is preferred for smaller ones. Additionally, the pH and ionic concentration of the buffer solutions used during electrophoresis also play pivotal roles in defining the efficiency of DNA migration. An understanding of these elements assists researchers in optimizing their experiments.
Historical Context
The roots of electrophoresis trace back to the early twentieth century, with the first documented instances appearing in the 1930s. Initial studies concentrated on protein analysis, laying the groundwork for methods still in use today. Notably, the advent of DNA electrophoresis in the 1970s coincided with the discovery of the double helix structure by Watson and Crick. This period marked a significant shift in molecular biology, as scientists sought techniques to manipulate and study DNA at a granular level.
As technology advanced, so did the methods used in electrophoresis. The introduction of agarose gel electrophoresis opened doors for more comprehensive DNA studies, facilitating discoveries in genetics and heredity. Over the decades, continual enhancements in equipment and methodologies have empowered scientists to refine their techniques, resulting in more precise and efficient analyses.
It is noteworthy that the evolution of DNA gel electrophoresis reflects broader trends in scientific inquiry – as research methodologies evolve, so too does our understanding of the intricate behaviors of biological molecules. Those studying electrophoresis today inherit a rich history that underscores the value of patience and innovation in scientific progress.
Principles of Gel Electrophoresis
Understanding the principles of gel electrophoresis is critical as it lays the groundwork for performing and interpreting experiments in molecular biology. This technique separates nucleic acids based on size and charge, facilitating a range of applications from DNA analysis to forensic investigations. By grasping these principles, researchers can optimize conditions to enhance clarity and efficiency in results.
Basic Mechanism
The basic mechanism of gel electrophoresis involves an electrical field applied across a gel matrix, typically composed of agarose or polyacrylamide. When an electrical current is applied, negatively charged DNA fragments migrate towards the positively charged electrode. The gel acts like a sieve, allowing smaller DNA fragments to move faster than larger ones. This differential migration creates distinct bands within the gel. Essentially, it is the interplay of charge and size that governs the movement of these fragments through the gel.
With the right conditions, the process can yield high-resolution separation of DNA, allowing for effective analyses such as fragment size estimation or purity assessments. The application of voltage and gel composition are key factors that influence this mechanism.
Factors Affecting Migration
Several factors affect the migration of DNA fragments during electrophoresis. Understanding each factor helps refine the experimental conditions for desired outcomes.
Voltage
Voltage plays a significant role in dictating the speed at which DNA travels through the gel. A higher voltage increases the electric field strength, leading to faster migration of the fragments. However, this must be managed carefully. If the voltage is too high, it may result in overheating of the gel, which can distort the bands, thereby compromising the results. Conversely, low voltage can lead to longer run times that may allow diffusion, resulting in band smearing. Therefore, selecting an appropriate voltage is crucial for maintaining the integrity of the bands.
Gel Composition
The gel composition is another critical factor that influences the behavior of DNA during electrophoresis. Different types of gels, such as agarose and polyacrylamide, offer varied pore sizes, which can affect how DNA fragments migrate. Agarose gels are generally used for larger DNA fragments, as they provide larger pores, while polyacrylamide gels are suitable for smaller fragments due to their finer resolution. This selection impacts separation efficiency directly, hence understanding the specific needs of the fragments being analyzed will guide appropriate gel choices.
Concentration of DNA
The concentration of DNA in samples also bears significant weight on migration. Higher concentrations may lead to overcrowding in the wells, exacerbating the risk of band smearing and signal interference. Conversely, very low concentrations can yield faint bands that may be challenging to visualize. Finding that sweet spot is essential, as it affects not only the clarity of results but also the reliability of repeated analyses.
In summary, the principles governing gel electrophoresis, especially the basic mechanisms and factors affecting migration like voltage, gel composition, and DNA concentration, are fundamental to mastering the technique. By paying close attention to these elements, researchers can ensure more precise and effective separation of nucleic acids.
Materials Required for DNA Gel Electrophoresis
Understanding the materials needed for DNA gel electrophoresis isn’t just a nice-to-have; it’s essential for the successful execution of this technique. The right mix of materials significantly impacts the quality of results, making this section a cornerstone of the entire process. Utilization of appropriate substances can enhance separation and decrease error margins, ultimately benefiting any research endeavor.
Gel Preparation Materials
Agarose or Polyacrylamide
When it comes to choosing a medium to separate DNA fragments, agarose and polyacrylamide often enter the conversation. Agarose is favored for its ability to create gels that are easy to handle and pour. The structure of agarose is more porous, making it suitable for larger DNA fragments, which is a big plus in many molecular biology experiments.
On the other hand, polyacrylamide gels provide higher resolution for smaller DNA fragments. Polyacrylamide’s unique characteristic is that it can offer fine control over pore size, allowing careful tuning to achieve specific separation needs. This feature makes it a common choice in applications such as protein studies where precision is crucial.
But keep in mind, agarose gels are generally simpler to prepare and less hazardous, making them a go-to for straightforward DNA analyses. In contrast, the polyacrylamide preparation requires more expertise due to its toxic nature during polymerization. So, while both options have their merits, the choice ultimately hinges on the specific requirements of the separation task at hand.
Buffers and Reagents
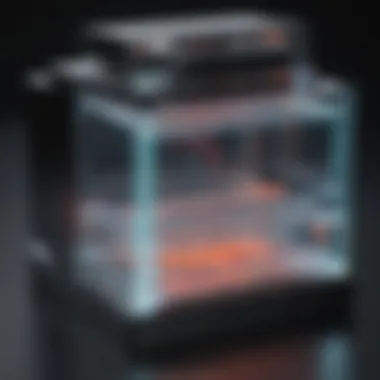
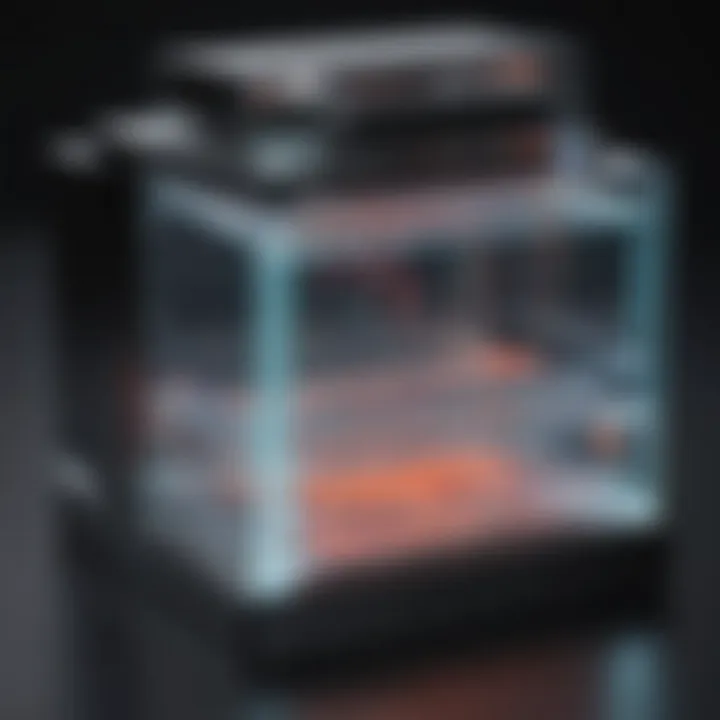
Buffers are the unsung heroes of gel electrophoresis. They maintain the pH throughout the process, ensuring that the DNA molecules don’t undergo unwanted reactions that could affect their integrity or migration behavior. TBE (Tris-Boric Acid-EDTA) and TAE (Tris-Acetate-EDTA) are two popular choices. Each has its own characteristics; TAE is generally better for running gels over extended periods, while TBE is often the preferred choice for shorter runs. This makes it crucial for researchers to know which buffer best suits their experiment.
Reagents like DNA loading dye also play a vital role. They not only help visualize the sample during loading but also allow for monitoring the progress of electrophoresis. Customarily, a loading dye contains a tracking dye that migrates along with the DNA, ensuring that the latter stays on track. This portion of the procedure is often overlooked but is fundamental in preventing mishaps that could lead to erroneous interpretations.
Electrophoresis Equipment
The equipment needed for DNA gel electrophoresis cannot be overstated; they are the backbone of the entire operation. Quality equipment not only improves accuracy but can also save time.
Gel Tank
A gel tank holds the agarose or polyacrylamide gel and facilitates the migration of DNA when electric current is applied. The design of the gel tank is crucial; it should be sturdy, impermeable, and able to accommodate the gel size needs based on the sample types being processed. Some advanced models even come with integrated cooling systems that help maintain a consistent temperature, preventing distortion of the results.
What sets a good gel tank apart is its user-friendliness. A well-designed tank allows for easy gel replacement and offers clear, visible markers for sample loading. If you find yourself wrestling with equipment rather than focusing on results, it's high time for an upgrade.
Power Supply
The power supply unit is another key player, dictating the current that drives the DNA separation process. Regulated power supplies provide the right voltage and amperage, safeguarding against abrupt fluctuations that could ruin an experiment. A constant voltage is essential for consistent results; varying currents can lead to uneven separation.
Moreover, some advanced power supplies come with features designed to optimize runs, like automatic shut-off systems when the gel has completed its run. Investing in quality here can pay off big time in terms of reliability and efficiency, allowing for the flow of results without hitch.
Loading Dye
Finally, the loading dye should not be an afterthought. Although it may seem minor, loading dye is important for both sample visualization and control. It typically contains dyes like bromophenol blue and xylene cyanol, which travel at predictable rates in the gel. This means they serve as benchmarks to observe the progress of electrophoresis.
Loading dyes come in various formulations; some are even optimized for specific applications. The presence of glycerol in certain loading dyes also helps the sample sink into the wells rather than dispersing. As a detail that often gets overlooked, a good loading dye can be the difference between a failed run and successful band visualization.
In summary, the materials and equipment required lay the groundwork for effective gel electrophoresis. From gel preparation to electrophoresis apparatus, each component plays a pivotal role that must be carefully considered to achieve reliable and interpretable results.
Step-by-Step Procedures in Gel Electrophoresis
In the intricate world of molecular biology, understanding DNA gel electrophoresis is essential. This section lays out the procedural steps, which are crucial to achieving accurate and reliable results. By following meticulous instructions in each phase, one can ensure not only the integrity of the DNA samples but also the effectiveness of the analysis that follows. Each step contributes to a well-structured process that yields informative data about the genetic material of the organisms being studied.
Preparation of the Gel
Determining Gel Concentration
When it comes to determining gel concentration, the percentage of agarose in the gel is a decisive factor impacting resolution and separation of DNA fragments. Generally, a low concentration, around 0.5% to 1%, is ideal for larger DNA fragments greater than 500 base pairs. Conversely, higher concentrations (up to 3% or more) improve resolution for smaller fragments. This adaptability is one of the key characteristics that makes adjusting gel concentration a popular choice in laboratories.
Furthermore, it’s important to note that while high concentrations provide better separation of smaller fragments, they can also lead to poor mobility of larger fragments, making it a double-edged sword in practice. Thus, meticulous planning regarding concentration based on the expected sizes of fragments can avoid common pitfalls in the electrophoresis journey.
Mixing and Melting the Agarose
Mixing and melting the agarose is another vital step, ensuring the gel solidifies uniformly. The agarose powder must be dissolved in an appropriate buffer solution, usually TAE or TBE, using heat. This process not only ensures homogeneity but also helps in achieving a clear gel without clumps or inconsistencies.
Care must be taken, as overheating the agarose can lead to the breakdown of the gel matrix, compromising its structural integrity. Thus, watching the melting process closely is pivotal. This phase is seen as beneficial because uniform gels provide reproducible results when running samples, aiding in consistent data collection and comparison across experiments.
Casting the Gel
Creating a Gel Tray
The next step, creating a gel tray, might seem trivial, but it's an integral part of the electrophoresis setup. A gel tray serves as a mold for the agarose and affects how the gel sets, since a well-constructed tray keeps the gel evenly distributed, facilitating optimal dye migration during the run.
Using a tray with proper dimensions aligned with the gel block size can ensure an even gel thickness, impacting the uniformity of DNA banding patterns. A poorly made tray can lead to uneven gel dimensions, resulting in distorted results. Hence, the precision in creating a gel tray cannot be overstated.
Removing Air Bubbles
Another common challenge in the process is removing air bubbles from the gel after casting. Air bubbles can obstruct DNA migration and create artifacts that confuse interpretation of results.
One effective way to eliminate bubbles is to gently tap the tray after pouring the gel, which usually helps displace air trapped in the gel mixture. Despite seeming like a mundane task, the impact of clean flow in the gel on electrophoresis results is critical since even the smallest bubble can alter the behavior of an entire gel lane significantly. This task is simple yet essential, highlighting the importance of detail-oriented techniques in successful DNA analysis.
Sample Preparation
DNA Fragment Isolation
Moving on to DNA fragment isolation, it's evident that this phase plays a fundamental role. By isolating specific fragments for the experiment, we ensure that the gel analysis reflects only the desired sections of DNA.
Several methods like PCR amplification or gel extraction techniques can be utilized for isolation. Each method comes with its unique advantages and can enhance the quality of the sample. The idea is straightforward; when you analyze only relevant fragments, you eliminate the noise from unrelated DNA infraction. Thus, isolating fragments not only improves clarity but also strengthens the accuracy of the gel electrophoresis findings.
Mixing with Loading Dye
Once the DNA fragments are isolated, the next step is mixing with loading dye. This preparatory step not only helps in visualizing the sample during loading but also aids in tracking DNA migration during the run.
Loading dye contains components that will weigh down the DNA, allowing it to sink into the wells and enabling easier handling. It is a key characteristic of this step, making it indispensable for efficient sample loading, as without it, samples might diffuse out too quickly or float away. This addition of dye equips researchers with the ability to monitor progress effectively, ensuring a seamless electrophoresis experience.
Loading the Gel
Using Pipettes
During the loading of the gel, using pipettes accurately is paramount. Precision in loading samples into the wells can greatly affect the clarity of the results.
A well-calibrated pipette ensures that identical volumes of sample are loaded, preventing issues like uneven banding or mixing between lanes. Improper technique in this phase can lead to contamination, leading to unreliable results. Hence, skillful pipetting becomes a hallmark of quality in DNA electrophoresis, underscoring the importance of mastering this technical skill for reproducible outcomes.
Ensuring Proper Sample Loading
Alongside pipetting, ensuring proper sample loading is crucial. This means carefully placing samples without overfilling the wells or causing them to run together. Ensuring the samples are settled right at the bottom is vital. It’s not just about physics here but ensuring a well-structured lane in the context of the gel itself.
Overloading can cause bands to smudge or overlap in a way that complicates data interpretation. Thus, precision in loading must not be dismissed, as it affects overall experiment outcomes dramatically.
Running the Gel
Setting Voltage and Time
Finally, after loading, one reaches the stage of setting voltage and time. Voltage settings directly influence the speed at which the DNA moves through the gel. High voltage can lead to increased migration speeds, but it can also introduce heating that distorts results.
It's thus crucial to find a balance between speed and sample integrity. Setting the right parameters ensures that the gel run is not only timely but also that the DNA maintains its integrity during migration. A well-run gel reflects thoughtful choices made in this phase, showcasing the thoughtfulness required throughout the entire procedure.
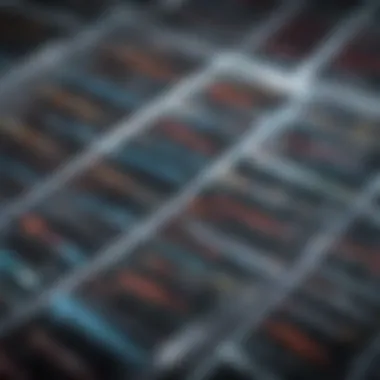
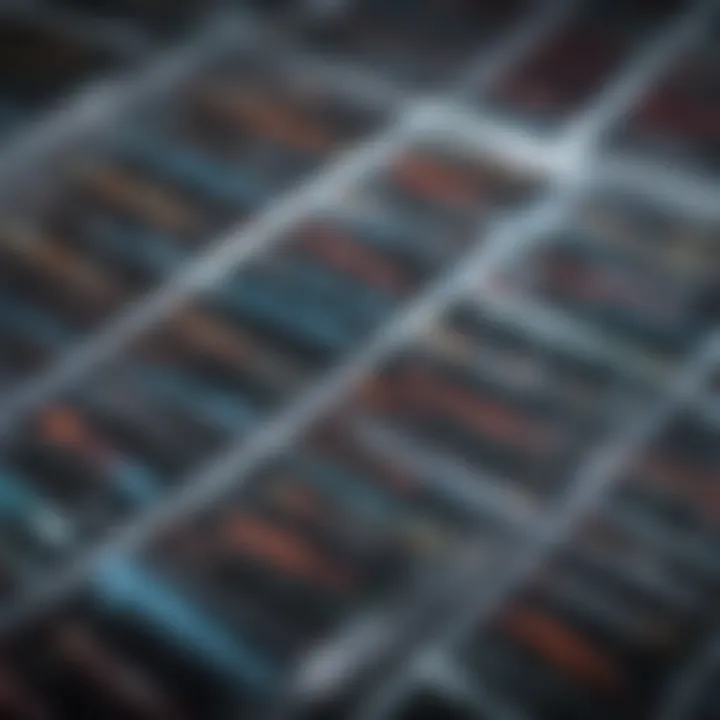
Monitoring Progress
During the run, monitoring progress is essential. It involves checking regularly that the DNA bands are moving as expected, without showing signs of disproportionate diffusion or distortion.
Utilizing a UV transilluminator at intervals can provide real-time feedback on the migration patterns. Any deviations can be addressed immediately, saving valuable time and effort before reaching final results. This stage shows that even minor observations can lead to substantial impacts on data quality.
In summary, each procedural step is interconnected and crucial. From preparing the gel to correctly loading and monitoring it, every action contributes to the precision and reliability of DNA gel electrophoresis.
Careful consideration and attention to detail at each step not only enhances the process but leads to meaningful findings in the realm of molecular biology.
Post-Electrophoresis Analysis
Post-electrophoresis analysis is a critical phase in the application of DNA gel electrophoresis. It serves as the bridge between simply running an experiment and interpreting its outcomes effectively. After the electric current has moved the DNA fragments through the gel and they have been separated based on size, the next steps determine the clarity of results and their potential implications in further research or diagnostics.
Staining the Gel
The staining phase is where the invisible becomes visible. Without proper staining, you are left looking at an empty gel, which adds to the frustration of an already meticulous process.
Ethidium Bromide
Ethidium bromide is perhaps the most commonly used stain in DNA gel electrophoresis. This compound intercalates between the base pairs of DNA, allowing fragments to emit fluorescence when exposed under UV light. One significant characteristic of ethidium bromide is its effectiveness in detecting even minute quantities of DNA. This aspect makes it a preferred choice for many laboratories focused on molecular biology.
However, ethidium bromide carries its risks. It's a potent mutagen and can pose health hazards if not handled with care. Researchers often use personal protective equipment and keen awareness of disposal methods when working with this stain. Another limitation is the potential for photobleaching; high-intensity light can diminish fluorescence, leading to less effective results over time.
Alternative Stains
As scientific inquiry evolves, so do the alternatives to ethidium bromide. Some researchers favor dyes like SYBR Safe or GelGreen, which provide safer handling with similar fluorescence properties. These stains offer advantages such as reduced toxicity, making laboratory conditions safer for users.
These alternatives, however, may differ in sensitivity and detection limit compared to ethidium bromide, requiring some adjustments depending on the specific experimental needs. Importantly, these options continue to gain traction due to their non-mutagenic nature, appealing to labs aiming to promote safer practices.
Visualizing the Results
Once the gel is stained, visualizing results becomes imperative to understanding what’s actually going on in your samples.
UV Light Exposure
UV light exposure is the next step in making the DNA bands visible. When using ethidium bromide, a UV transilluminator is essential to illuminate the fluorescence emitted by the stained DNA fragments. A distinct but crucial aspect of this process is ensuring that the wavelength used matches the emission spectrum of the stain. This method is widely favored because it allows for the rapid visualization of bands without extensive downstream processing.
Nonetheless, UV light has its drawbacks. Prolonged exposure can damage the DNA, affecting downstream applications like cloning or sequencing. Careful planning around the timing and duration of exposure can mitigate these risks, ensuring the integrity of samples while still capturing the essential data.
Image Capture Techniques
Once bands are illuminated, the next step is capturing the results for analysis. Image capture techniques can vary widely, ranging from simple camera setups to advanced gel documentation systems. A key feature of these systems is their ability to enhance and analyze specific bands, providing quantitative data, which can be vital for gene expression studies or other genomic investigations.
Many systems today also integrate analysis software that can quantify band intensity or even size, which allows for more refined results interpretation. However, some capture methods may introduce artifacts or shadows, compromising data accuracy. Choosing the right technique and equipment for your specific need is key to achieving reliable and accurate visual representation of your results.
"Staining and visualizing results is where the magic happens; it's the moment of truth in gel electrophoresis".
In summary, post-electrophoresis analysis encapsulates the steps that follow the execution of gel electrophoresis, offering a detailed evaluation of findings. By delving into both the staining and visualization phases, we can grasp the nuances that transform raw data into meaningful insights.
Interpreting Gel Electrophoresis Results
Understanding how to interpret the results of gel electrophoresis is not just an extension of this technique; it's a crucial part of the scientific process that opens the door to various applications in research and diagnostics. Being able to accurately analyze and interpret the bands seen on the gel can help in determining DNA sizes, evaluating the quality of your samples, and identifying potential issues that could arise during the experimental process.
Analysis of Bands
Estimating DNA Size
Estimating the size of DNA fragments is fundamental in any experiment involving gel electrophoresis. This estimation allows researchers to compare their samples against a standard ladder or marker that specifies known sizes. The size determines how effectively a particular fragment can be amplified or sequenced in downstream applications. This characteristic is not just important; it is vital for quantifying biological information accurately.
The most common method for estimating DNA size involves measuring the distance migrated by the DNA fragments relative to the marker. It provides a quick reference to assess whether the expected fragment size matches what has been obtained. As such, this method is often favored for its straightforward approach and efficiency in outcome evaluation. However, misestimating sizes is a common pitfall, particularly in low-resolution gels, leading to erroneous conclusions.
- Key characteristics: Quick to assess.
- Benefits: Allows simple comparisons with known standards.
- Disadvantage: Risk of inaccuracy if gel concentration affects migration.
Comparative Analysis
Comparative analysis takes the results a step further by comparing samples to one another. This approach is particularly useful when evaluating multiple samples to assess genetic similarities or differences. It's about gaining insight into the landscape of genetic makeup, which can have wider implications in research endeavors.
Comparative analysis can highlight how closely related different samples are, based on band patterns. It becomes important in applications such as genotyping and DNA fingerprinting. Its unique feature lies in its ability to derive more detailed conclusions by visualizing multiple samples side by side. A notable advantage is that this method can reveal subtle variations in band patterns that might indicate genetic mutations or polymorphisms; however, it does demand careful control of experimental conditions to minimize variability.
- Key characteristics: Insight into genetic relationships.
- Benefits: Can indicate potential genetic mutations or variances.
- Disadvantage: Complexity increases with sample number.
Common Issues and Troubleshooting
Smearing
Smearing is one of the most common issues faced during gel electrophoresis. When separation fails, researchers may notice that their DNA appears more like a smudge or streak rather than distinct bands. This occurs when too much DNA is loaded into the well or when the gel concentration isn't appropriate for the size of the DNA fragments being analyzed. Smearing can severely impact the interpretation of results; instead of clear bands, you might end up with a blurry mess.
- Key characteristics: Blurry or indistinct bands.
- Benefits: Alerts researchers to potential issues in sample integrity.
- Disadvantage: Makes accurate analysis next to impossible.
Low Quality Bands
Low-quality bands refer to those that appear faint or poorly defined. They can occur due to insufficient DNA concentration, improper loading, or degradation of the DNA sample prior to running the gel. This can lead to challenges in interpretation as it becomes difficult to gauge the actual presence and size of DNA. In applications where quantification matters, such as in PCR products, low-quality bands can throw off the entire downstream process.
- Key characteristics: Faint appearance on the gel.
- Benefits: Can indicate the need for optimization of the gel conditions.
- Disadvantage: Complicates the assessment of results and can skew data.
Therefore, interpreting the results requires careful attention to detail and a good understanding of both the techniques and the biology behind the samples being studied. It’s an essential skill for any scientist working with molecular biology, genetics, or forensic science.
Applications of DNA Gel Electrophoresis
DNA gel electrophoresis serves as a cornerstone in molecular biology, providing a method through which researchers can visualize, analyze, and manipulate DNA fragments with precision. This technique holds immense significance across various disciplines, typifying its application in both basic research and practical settings. Understanding its applications can enlighten practitioners on how this tool not only facilitates experiments but also contributes to advancements in science and technology.
Molecular Biology Research
Gene Cloning
Gene cloning is a fundamental process in molecular biology where scientists replicate a specific gene of interest. DNA gel electrophoresis plays a pivotal role in this context by allowing researchers to separate the DNA fragments resulting from the restriction enzyme digestion. A crucial aspect here is the ability to estimate the size of DNA fragments, ensuring that the desired gene is accurately isolated.
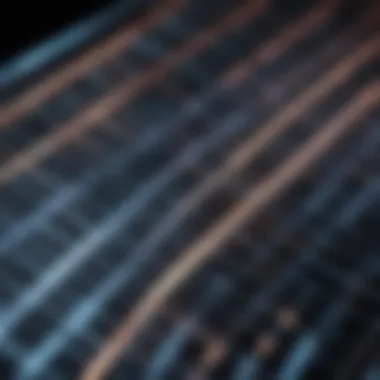
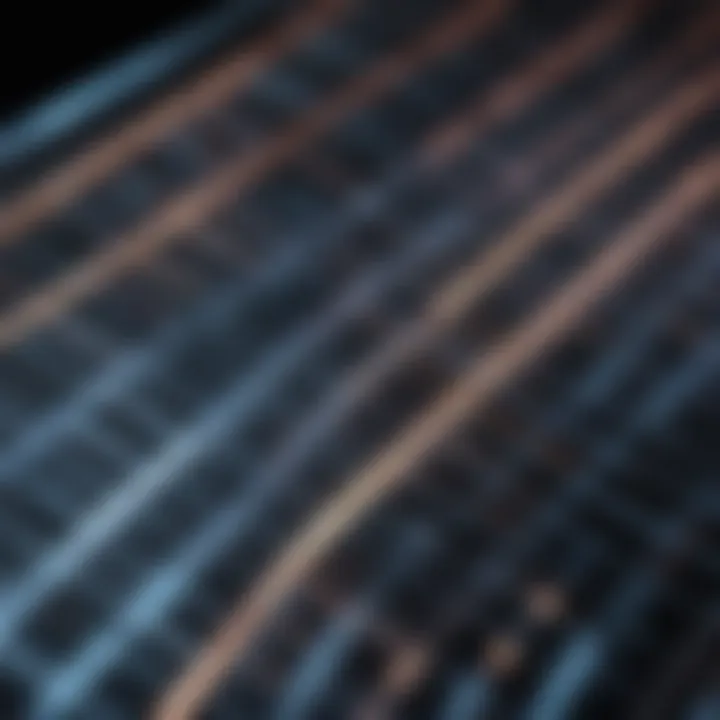
The key characteristic that makes gene cloning stand out is its versatility. This method can be adapted to clone genes from various organisms, making it a popular choice among molecular biologists. Noteworthy is how DNA gel electrophoresis aids in verifying successful cloning. It’s not just about cutting the DNA; it’s about confirming the inclusion of the gene in a vector, which is facilitated by observing clear bands on the gel.
Advantages of Gene Cloning:
- High specificity in target gene isolation.
- Facilitates functional studies and protein expression.
- Supports variety of applications like producing recombinant proteins or studying gene function.
Disadvantages:
- Can be resource-intensive, requiring careful planning and reagents.
- Potential for issues like mutations during amplification.
Genotyping
Genotyping is another significant application of gel electrophoresis, often used to identify variations in DNA sequences. By comparing the banding patterns obtained from different samples, researchers can infer genetic differences, making this an invaluable method for genetic population studies.
The core of genotyping lies in its reliability and accuracy. With the help of gel electrophoresis, scientists can visualize alleles and assess genetic diversity within populations. The technique uses size-based separation, helping to elucidate whether individuals carry certain alleles associated with specific traits.
Advantages of Genotyping:
- Enables identification of genetic variations with high precision.
- Supports utilization in various fields including agriculture and medicine.
Disadvantages:
- Requires meticulous sample preparation to avoid errors.
- Limited to identifying known variations; novel mutations might be overlooked.
Forensic Science
Forensic applications of DNA gel electrophoresis illustrate its relevance beyond research settings. In criminal investigations, it has become a tool of choice, particularly for DNA profiling, which provides scientific backing in legal cases.
DNA Fingerprinting
DNA fingerprinting is a powerful technique allowing forensic scientists to identify individuals based on their unique DNA profiles. During investigations, this method can differentiate between individuals with high accuracy, making it invaluable in criminal justice.
A major characteristic of DNA fingerprinting is its high discriminative power. Quick and precise analysis of small DNA samples can often yield critical evidence. What makes it particularly remarkable is its non-invasive nature, as DNA can be extracted from a variety of sources, from blood to hair.
Advantages of DNA Fingerprinting:
- Can analyze minute samples, providing flexibility in forensic investigations.
- High accuracy in individual identification supports legal processes.
Disadvantages:
- Ethical concerns surrounding privacy and consent.
- Potential for contamination or sample degradation.
Ancestry Testing
Ancestry testing has gained popularity, enabling individuals to discover their genetic heritage. The role of DNA gel electrophoresis in this application is significant, as it helps verify relationships and trace lineage. By analyzing markers associated with specific populations, companies can provide insight into an individual's ancestral roots.
The uniqueness of ancestry testing lies in its personal relevance. Many individuals seek such tests to connect with their identity, making it a beneficial choice for consumers intrigued by family history studies. The comprehensive analysis that comes with electrophoresis offers clarity in lineage mapping.
Advantages of Ancestry Testing:
- Provides meaningful connections to heritage and identity.
- Engages a broad audience, from casual users to serious genealogists.
Disadvantages:
- Results can be misinterpreted or sensationalized.
- Privacy concerns regarding genetic information.
Understanding these applications not only showcases the versatility of DNA gel electrophoresis but also emphasizes its impact on both scientific research and societal interests.
Future Trends in Gel Electrophoresis Technologies
The field of gel electrophoresis isn't static; it's constantly evolving. New technologies are emerging to make this essential technique faster, more efficient, and accessible. Understanding these trends is crucial for anyone involved in molecular biology and related fields. With advancements in equipment and integration with other technological innovations, the future of gel electrophoresis looks promising. By exploring these shifts, we can gain insights into how they might enhance research capabilities and data analysis.
Advancements in Electrophoresis Equipment
Recent developments in electrophoresis equipment are paving the way for more precise and quicker analyses. For instance, some newer gel electrophoresis systems come equipped with improved power supplies that allow for variable voltage settings, providing greater control over the migration speed of DNA fragments. This leads to better resolution and clearer bands, essential for accurate interpretations of results.
Another advancement involves miniaturization. Pocket-sized electrophoresis units are becoming available, making it possible to run gels in even the most modest lab setups. These advancements are significant, especially in teaching labs, where space is often at a premium. The compact systems make it easier for students to grasp essential techniques without needing large, bulky apparatus.
Integration with New Technologies
Automated Systems
The integration of automated systems into gel electrophoresis marks a significant leap forward. These systems are designed to streamline the process, minimizing human error. By automating sample loading and gel running, researchers can focus on interpreting results rather than spending time on manual tasks. The key characteristic of automated systems is their reproducibility; they consistently produce the same quality of results across different runs.
Moreover, many automated systems come with built-in imaging capabilities as well. This feature allows for the instant capture of results, enabling real-time analysis. One standout advantage is their ability to handle multiple samples simultaneously, which is a significant boon for projects requiring high sample throughput. However, a potential downside might be the initial investment cost, which could be a barrier for smaller labs.
High-Throughput Screening
High-throughput screening systems are taking gel electrophoresis to new heights. This approach allows researchers to analyze large numbers of samples quickly. The unique aspect of high-throughput screening is its scalability; researchers can run multiple gels side-by-side, drastically reducing the time needed for data collection.
This method is especially beneficial in genomics and drug discovery, where speed is essential. However, while the primary advantage is efficiency, the technique may present challenges in data management. Handling and interpreting large datasets require significant computational resources and expertise.
In summary, the integration of advanced equipment and modern technologies is not just enhancing gel electrophoresis; it's revolutionizing it. As we look to the future, the potential for increased accuracy, efficiency, and accessibility bodes well for the ongoing advancement of molecular biology research.
Closure
In wrapping up this comprehensive guide to DNA gel electrophoresis, it becomes clear that understanding this intricate technique is more than gaining mere knowledge; it’s about recognizing its immense value in scientific research and various applications. The conclusion serves not only as a summary but as a vital bridge towards appreciating the impact that this method has had across multiple disciplines, from molecular biology to forensic science.
Summary of Key Points
- Fundamental Technique: DNA gel electrophoresis plays a crucial role in separating DNA fragments based on size, making it essential for various biological analyses.
- Step-by-Step Approaches: The process includes multiple stages—gel preparation, sample loading, running the gel, and result analysis. Each step is integral for achieving reliable outcomes.
- Application in Research: This method is pivotal for gene cloning, genotyping, DNA fingerprinting, and even ancestry testing. By supplying clear visual data, researchers can effectively interpret genetic information.
- Future Perspectives: As equipment and technologies evolve, DNA gel electrophoresis is set to benefit from more automated systems and higher throughput screening, promising enhancements in efficiency and accuracy.
Reflections on Research Impact
The impact of DNA gel electrophoresis on research cannot be understated. It has facilitated breakthroughs in genetic research, opened avenues in forensic analysis, and contributed to our understanding of hereditary diseases. The ability to visualize DNA has democratized scientific inquiry, allowing students and researchers alike to explore complex genetic relationships with relative ease.
In these modern times, as we face enormous challenges related to health and genetics, refining the techniques and expanding the application of gel electrophoresis may yield even greater insights in the years to come. Thus, reflecting on the advancements in this area encourages a sense of responsibility for upcoming generations of scientists—fostering innovation while grounding in classic methods.
"As we enhance the technology of today, let’s not forget the foundational techniques that paved our path towards scientific discoveries."
In essence, the knowledge gleaned from comprehending DNA gel electrophoresis is not merely academic; it shapes our outlook on genetics and empowers future exploration in this fascinating field.