Dynamic Light Scattering Techniques for Nanoparticles
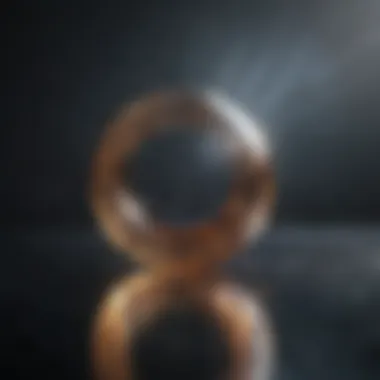
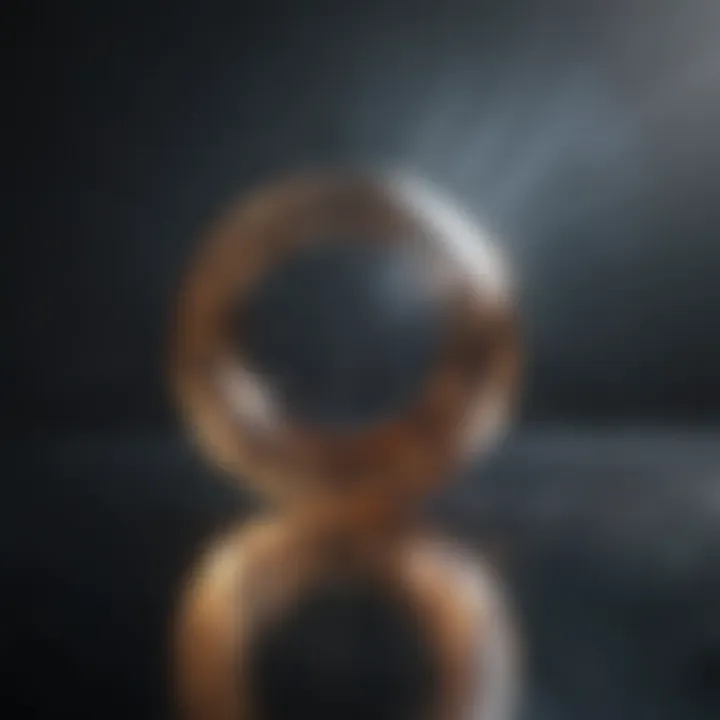
Intro
Dynamic Light Scattering, often abbreviated as DLS, has carved a niche for itself in the analytical landscape, particularly when it involves the study of nanoparticles. This technique, which leverages the scattering of light by small particles in suspension, opens the floodgates to a realm of insights regarding particle size distribution and stability. For students, researchers, and professionals alike, it provides a window into the nuances of nanoparticle behavior in various environments.
DLS is not just a toolbox for scientists; it is a fundamental method that facilitates the understanding of how nanoparticles interact with each other and their surroundings. This understanding is crucial, especially in fields like materials science, drug delivery, and nanotechnology, where precise characterization is paramount.
Research Overview
In light of the growing interest in nanoparticles, the research surrounding DLS has undergone significant expansion. Key findings indicate that DLS not only reveals size distribution but also helps in assessing the stability of colloidal systems. In practical applications, insights from DLS can influence the formulation of drugs and the development of new materials.
The importance of this research extends far beyond academic boundaries; it has implications in industrial applications and healthcare innovations. With the increasing use of nanoparticles in diagnostics and therapeutics, understanding their properties at the nanoscale has never been more vital.
Significance of DLS in Nanoparticle Research
- Size Distribution Insights: DLS provides a method for measuring the hydrodynamic size of nanoparticles, an essential parameter for their performance in various applications.
- Stability Evaluations: By tracking how particles behave in suspension over time, researchers can infer the stability of formulations.
- Fundamental Understanding of Interactions: DLS sheds light on how nanoparticles aggregate or disperse in different media, offering insights crucial for material design.
Understanding these facets not only enhances fundamental research but also informs practical applications, positioning DLS as a cornerstone technique in nanoparticle analysis.
Methodology
Approaching the technique of DLS requires meticulous methodology, ensuring that results are reliable and relevant. The process typically encompasses several steps, notably in the realms of experimental design, data gathering, and analysis.
Experimental Techniques
Essential elements of DLS methodology include:
- Sample Preparation: The suspension must be adequately prepared to ensure a homogenous distribution of nanoparticles, as any aggregation could yield misleading results.
- Data Acquisition: Using a laser, the scattered light from the nanoparticles is collected. The fluctuations in intensity over time are analyzed to extract size information.
Sampling Criteria and Data Collection
The criteria for selecting samples are critically important.
- Typical Sampling Considerations:
- Particle concentration must be appropriate; too high can lead to multiple scattering, skewing results.
- Type of medium can affect light scattering and must align with the intended application.
In terms of data collection, DLS instruments typically provide real-time analysis, allowing for immediate interpretation of particle behavior under various conditions.
The elegance of DLS lies not only in its ability to provide immediate insights but also in the depth of information it offers about particle interactions and stability. The journey of understanding nanoparticles is intricate, and DLS stands out as a key contributor to the narrative.
Prologue to Dynamic Light Scattering
Dynamic Light Scattering (DLS) is an essential tool for analyzing nanoparticles, shedding light on their size distribution and behavior in various environments. This technique has gained significant attention in the realm of nanotechnology and material science due to its ability to provide real-time insights into the dynamic characteristics of nanoparticles. In a world where the manipulation of materials at the nanoscale opens new avenues for research and innovation, understanding DLS becomes paramount.
DLS offers several benefits that make it an attractive option for researchers. Firstly, the technique is non-invasive. It allows for the observation of particles in solution without altering their natural state. This is particularly important when studying delicate nanoparticles that might undergo changes with other methods. Secondly, DLS can yield rapid results, which is crucial when time is of the essence in experimental setups. Additionally, the technique can analyze a wide range of particle sizes, from a few nanometers to several micrometers, thereby catering to diverse research needs.
However, utilizing DLS comes with its own set of considerations. For instance, certain sample preparations must be meticulously executed to avoid artifacts that can skew results. Moreover, the properties of the dispersive medium significantly impact the scattering data, necessitating a thorough understanding of these factors.
As we delve deeper into the complexities of DLS, it is crucial to grasp not only how the technique operates but also its historical development and foundational principles. A comprehensive understanding of these aspects will serve as the bedrock upon which we explore its myriad applications in nanoparticle research.
"Understanding the nuance of DLS does not just empower researchers; it paves the way for novel discoveries in nanoscience, bridging the gap between theory and practical application."
In this article, we will traverse through the historical background of DLS, examining its evolution and significance. We will also unpack the fundamental principles that govern its operation, laying the groundwork for appreciating its pivotal role in characterizing nanoparticles.
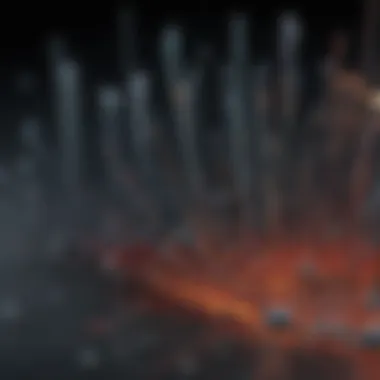
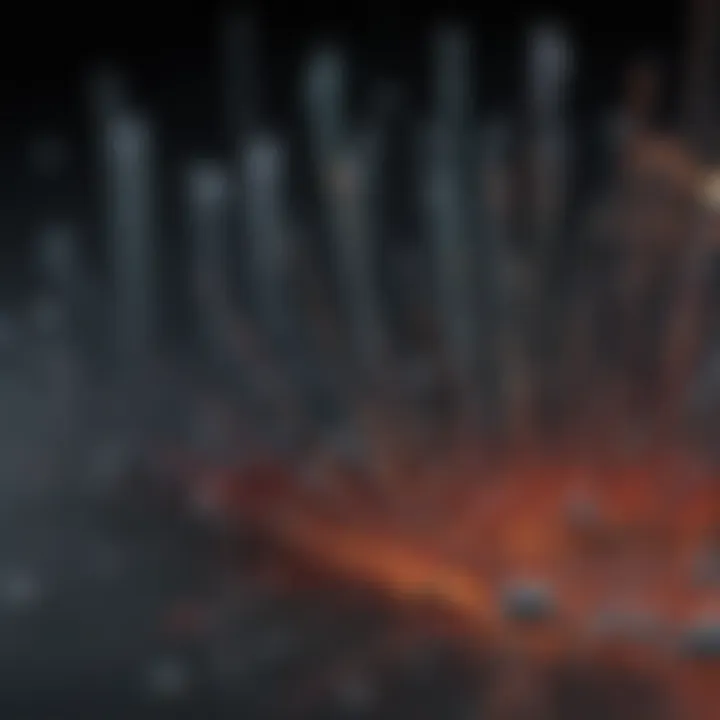
Understanding Nanoparticles
Nanoparticles have become a focal point in various areas of research and industry, and understanding them is crucial for utilizing their unique attributes effectively. From biomedicine to environmental applications, the role of nanoparticles is growing immensely, bridging gaps that were previously insurmountable with larger materials. Their minute size, often ranging from 1 to 100 nanometers, allows these particles to exhibit properties that differ significantly from their bulk material counterparts. This distinctive behavior is what makes grasping the concept of nanoparticles essential.
Definition and Classification
At its core, a nanoparticle can be defined as a microscopic particle whose size falls within the nanoscale range. They can be classified into various categories based on their composition, shape, and application. Some common classifications include:
- Metallic nanoparticles: Often formed from gold, silver, or copper, these are used in applications ranging from drug delivery to sensors.
- Polymeric nanoparticles: Created from synthetic or natural polymers, these are significant in drug formulation and tissue engineering.
- Ceramic nanoparticles: These include metal oxides such as titanium dioxide and silica, commonly utilized in electronics and environmental remediation.
Defining nanoparticles is only part of the picture; classification not only aids in understanding their diverse applications but also assists researchers in selecting suitable types for specific tasks. Moreover, knowing the types helps in addressing questions regarding biocompatibility, stability, and interaction with surrounding media.
Unique Properties of Nanoparticles
What specifically sets nanoparticles apart are their unique properties resulting from their incredibly small size and high surface-to-volume ratio. These properties include:
- Increased reactivity: Due to a larger surface area available for interactions, nanoparticles tend to be more reactive compared to bulk forms.
- Quantum effects: At nanoscale dimensions, particles can experience unique quantum mechanical effects, like changes in optical and electrical properties not seen in larger materials.
- Enhanced solubility: Many nanoparticles exhibit improved solubility in liquids, which is vital for applications in drug delivery systems.
Furthermore, nanoparticles can display behaviors not observed in larger particles due to their size, like superparamagnetism in iron oxide nanoparticles, which allows them to be manipulated with magnetic fields.
Understanding the classification and unique properties of nanoparticles provides a fundamental basis for leveraging their potential across a variety of fields. Such knowledge helps scientists and engineers navigate the complexities of their applications, leading to innovations that can positively impact numerous aspects of science and technology.
"The properties of nanoparticles can open brand new doors in technology, which were previously just dreams for researchers."
As the interest in nanoparticles grows, the importance of understanding them will only intensify, driving advancements in characterization techniques like dynamic light scattering. This understanding lays the groundwork for comprehending how techniques, including DLS, can be effectively utilized in nanoparticle research.
Significance of DLS in Nanoparticle Research
Dynamic light scattering (DLS) has become essential in the realm of nanoparticle research, primarily due to its capability to provide rapid, accurate, and reproducible measurements of particle size and distribution. Understanding the significance of DLS in this field goes beyond mere technicalities; it opens doors for innovations across various applications, ranging from biomedical to environmental science.
One of the primary benefits of utilizing DLS is its non-invasive nature. This attribute allows researchers to probe nanoparticle samples without altering their intrinsic characteristics. Consequently, the integrity of the samples is preserved, and real-time analysis becomes feasible. Whether in a lab or an industrial setting, DLS offers an efficient means to ensure the reliability of data obtained in nanoparticle studies.
The importance of accurate characterization cannot be overstated. Nanoparticles exhibit unique behaviors that differ significantly from their bulk counterparts due to their small size and high surface area. Misleading size distributions or erroneous stability assessments can lead researchers astray, possibly compromising the success of applications they aim to develop or enhance. Here’s where DLS shines, delivering precise measurements that inform vital decisions in drug formulation, material synthesis, and environmental assessments.
Moreover, the stability of nanoparticles in suspension is a focal point in many research scenarios, influencing their usability and efficacy in practical applications. This brings us to the two crucial aspects of DLS's role in nanoparticle research: characterization of nanoparticles and stability assessment.
Characterization of Nanoparticles
Characterizing nanoparticles is akin to finding the right key for a intricate lock—only with precise details can one unlock the potential they hold. DLS provides insights into the size distribution of nanoparticles, distinguishing aggregates from individual particles with remarkable efficacy. This capacity to determine hydrodynamic radius forms the foundation of further analyses and can be pivotal in applications such as drug delivery systems, where particle size can dictate biological interactions.
DLS measurements yield size distribution profiles that can be analyzed statistically. For instance, by employing the second cumulant analysis method, researchers can derive additional parameters, such as polydispersity index (PDI), which provides a numerical representation of the width of the size distribution. A low PDI indicates a uniform sample, essential in many formulations, especially for biopharmaceutical applications.
Furthermore, it aids in understanding how particles behave under various conditions, such as different solvent viscosities or ionic strengths. Recognizing these behaviors contributes substantially to tailoring nanoparticles for their intended applications. Knowledge gained through DLS is gaining ground as a standard aspect of comprehensive nanoparticle characterization, enhancing accuracy in multiple fields.
Stability Assessment
Stability is a game-changer in the practical application of nanoparticles. In assessments of nanoparticle stability, DLS offers insights into how particles react under varying environmental conditions. For instance, the presence of certain ionic strengths or pH levels may alter particle interactions, leading to agglomeration or precipitation. DLS can detect these changes, providing critical data that inform formulation adjustments in real time.
"In the world of nanoparticles, a stable formulation is often the difference between success and failure."
Assessing the stability of nanoparticles is often linked to their intended use. For drug delivery, metering the stability means ensuring that the therapeutic agents remain effective throughout their journey in the body. In environmental science, stability assessment is crucial in understanding pollutant behavior and lifecycle in ecosystems when nanoparticles interact with various environmental matrices.
There is no one-size-fits-all when it comes to nanoparticle applications; hence, the insights rendered by DLS technology are indispensable to anticipate and address challenges associated with nanoparticle formulation and application.
Technical Aspects of DLS
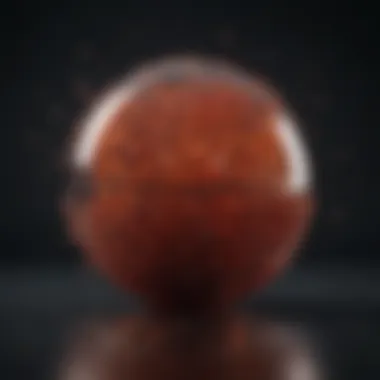
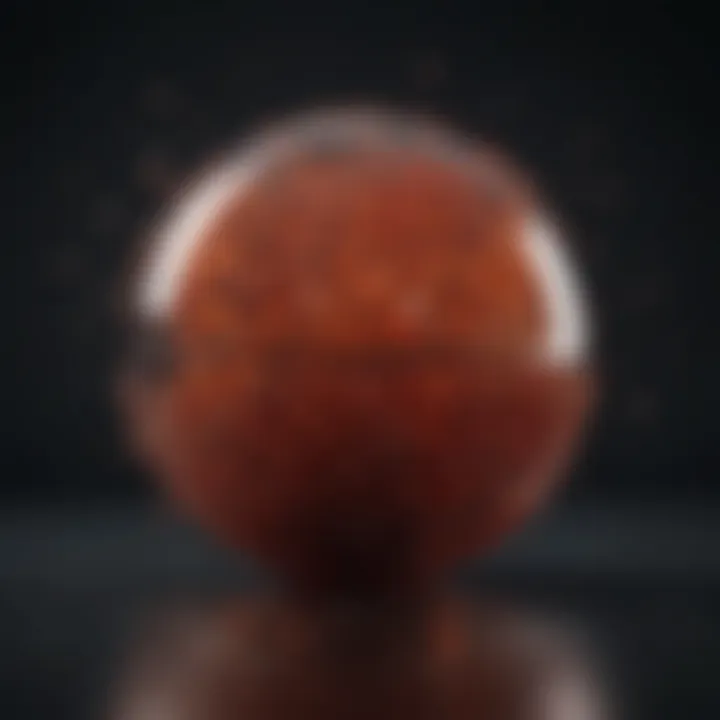
Understanding the technical aspects of Dynamic Light Scattering (DLS) is essential for employing this technique effectively in nanoparticle research. The intricacies of instrumentation, measurement techniques, and data analysis all combine to give researchers the ability to characterize nanoparticles in various states. This section will dive into each of these facets, highlighting their significance and the considerations that must be taken into account.
Instrumentation and Setup
The heart of any DLS analysis lies in its instrumentation. At the core, the DLS apparatus typically consists of a laser source, a sample chamber, a detector, and a computer system for data analysis. The laser provides the coherent light necessary for scattering measurements. This light interacts with nanoparticles suspended in liquid, leading to scattering phenomena that can be studied.
Correct setup is vital. Positioning the sample correctly in regards to the laser beam ensures optimal scattering light collection. For instance, a common configuration involves a 173-degree detection angle, approximating the angle that maximizes the intensity of scattered light. Improper alignment can lead to erroneous measurements, undermining the reliability of the results.
Moreover, temperature control is crucial. Fluctuations in temperature can alter the viscosity of the medium, influencing particle movement and light scattering. Therefore, many DLS systems are equipped with temperature sensors to maintain consistent conditions during measurements.
Measurement Techniques
Once the instrument is set up, the next step is selecting appropriate measurement techniques. DLS can assess particle size, distribution, and even interactions. The most commonly used measurement method in DLS is the autocorrelation function (ACF). This function correlates the intensity fluctuations of the scattered light over time, allowing researchers to determine the diffusion coefficient of the particles, which is then translated into size information through the Stokes-Einstein equation.
It's important to consider the concentrations of the samples being analyzed. Low concentrations are often preferable as they reduce multiple scattering effects, which can complicate the analysis. However, for some studies, higher concentrations may be necessary, in which case methods such as cross-correlation techniques can be employed. Such methods pair multiple detectors to creatively handle the complexities of scattering data.
Data Analysis Methods
Analyzing data from DLS requires both software and statistical scrutiny. Most modern DLS systems come equipped with proprietary software designed to process scattering data, calculating essential parameters like mean size, polydispersity index (PDI), and zeta potential. The PDI is an important measure for nanoparticle uniformity: lower values signify size homogeneity, which is critical in many applications like drug delivery.
A practical understanding of the data analysis methods is equally essential. Different algorithms—such as the CONTIN algorithm—serve to analyze the autocorrelation data. Each algorithm comes with its own set of assumptions and limitations, so knowing which is appropriate for a given study can impact the validity of the results.
"Thorough comprehension of measurement and data analysis techniques allows researchers to enhance the accuracy and reliability of particle characterization."
In summary, the technical aspects of DLS, from instrumentation and measurement techniques to data analysis methods, form a comprehensive framework that can profoundly impact nanoparticle characterization. Awareness of these elements increases not just understanding but also practical application in this rapidly advancing field.
Applications of DLS in Various Fields
The use of dynamic light scattering (DLS) has carved a niche in numerous scientific fields, owing to its pivotal role in the characterization of nanoparticles. Understanding the distribution of particle sizes, gauging their stability, and studying interfacial phenomena are just some of the significant benefits that DLS offers. This section delves into two crucial areas—biomedicine and environmental science—each highlighting how DLS contributes to their respective advancements.
Biomedicine
In biomedicine, the application of dynamic light scattering techniques has unlocked new avenues for drug delivery systems and therapeutic development. The biocompatibility of nanoparticles often hinges on their size, shape, and distribution; thus, DLS serves as a reliable method to monitor these characteristics. Here, the real-time tracking of particles can lead researchers to optimal formulations in pharmaceutical development.
- Drug Formulation: DLS aids in the formulation of nanoparticles by providing information about size distributions, which is paramount to achieving targeted delivery systems.
- Vaccine Development: The technology has become central in assessing vaccine particle sizes, crucial for efficacy and stability against pathogens.
Moreover, DLS contributes to determining the stability of nanoparticles in biological fluids. For instance, nanoparticles designed for drug delivery often encounter complex biological environments. Being able to analyze stability allows researchers to refine design parameters, ultimately enhancing therapeutic effectiveness.
"The understanding of particle behavior in physiological environments is vital for successful biomedical applications. DLS simplifies this by offering swift and reliable measurements of size and stability."
Environmental Science
Dynamic light scattering also holds essential significance in environmental science. The characterization of particulate matter such as aerosols or nanoparticles in suspension can unveil unprecedented insights into pollution and its impact on ecosystems.
- Pollution Monitoring: By analyzing the size distribution of airborne particles, DLS can help in tracking pollution patterns, revealing the dynamics of particulate matter under varying atmospheric conditions.
- Water Quality Assessment: The technique is equally vital in evaluating water samples; understanding the colloidal stability of pollutants and their aggregation behavior can inform remediation strategies.
In essence, the ability of DLS to analyze the size and concentration of nanoparticles in various environments underscores the importance of this technique in addressing contemporary environmental challenges. By integrating DLS with other analytical methods, researchers might forge a path towards more effective environmental monitoring and management strategies.
The integration of DLS in both biomedicine and environmental science underscores its multifaceted applications, hinting at a growing recognition of its importance in the characterization of nanoparticles. As researchers continue to explore the depths of these applications, the future promises an even greater impact on health and the environment.
Challenges and Limitations of DLS
Dynamic Light Scattering (DLS) is a valuable technique in the field of nanoparticle characterization, but like most methodologies, it comes with its set of challenges and limitations. Understanding these obstacles is crucial in ensuring the reliability of the results produced through this method. These issues can affect the accuracy and reproducibility of measurements, which are foundational aspects of any scientific inquiry.
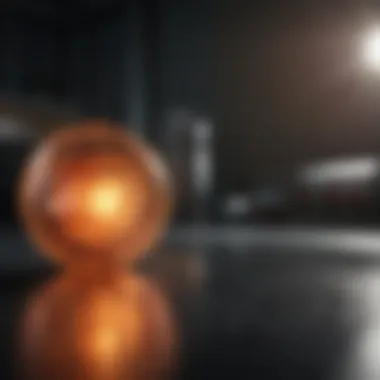
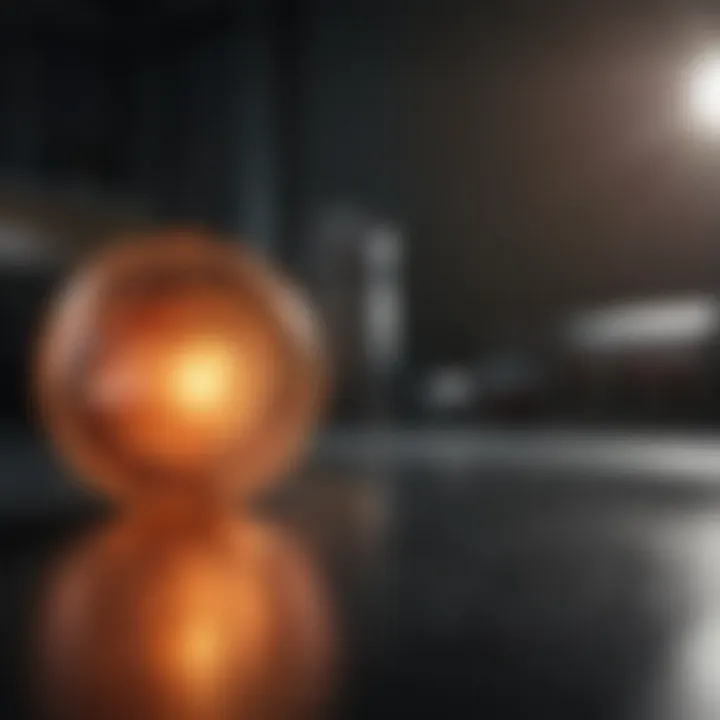
When delving into the DLS setup, researchers need to take a sensible approach and consider how various pitfalls can impact their studies. Here, we will explore some of the primary challenges associated with DLS, focusing on specific elements such as the preparation of samples, and the interference posed by the median properties.
Sample Preparation Issues
One major hurdle in DLS is related to sample preparation. It might seem like a straightforward task, yet the devil is really in the details. The way a sample is prepared can greatly influence the DLS results—everything from concentration to pH levels. It's essential to ensure that nanoparticles are uniformly dispersed, as clumps or aggregates can give skewed results.
- Concentration: A too-high concentration can lead to multiple scattering effects which can muddle the data and hinder the accurate interpretation of particle size distributions.
- Solution Medium: The nature of the solution in which the nanoparticles are dispersed plays a critical role. For instance, different solvents can cause varying degrees of interactions among particles, impacting their dynamics.
Moreover, the characteristics of the solvent such as viscosity and refractive index must align with the parameters of the DLS equipment. Inadequate dispersion, as well as fluctuations in temperature, can create a scenario where the results are unreliable. Getting the prep right is not just a step in the process; it sets the stage for the outcome of the entire experiment.
Interference from Medium Properties
Equally significant is the interference posed by the properties of the dispersing medium. These properties can either enhance or hamper the measurement process, affecting the final results. The refractive index of the medium impacts the scattering of light and, in turn, the accuracy of size measurements. If this is not accurately accounted for, the DLS may produce misleading size distributions.
Additionally, the viscosity of the medium is another important factor. High viscosity can slow down the Brownian motion of the nanoparticles, which can lead to underestimating their size. The density of the medium can also play a role—changes can create variability in the scattered light intensity and consequently affect the calculations based on that intensity.
"A thorough understanding of the medium used is crucial; neglecting these properties can result in flawed interpretations and misguided conclusions."
The impacts of these factors underscore the need for diligence at each phase of the experiment, from preparation to execution. Consequently, researchers must not view DLS as a fit-all solution but rather as a tool that requires careful calibration and adjustment in alignment with the properties of the samples used.
Understanding these challenges enables professionals and researchers to make informed decisions, optimizing their processes and improving the robustness of their findings in nanoparticle research.
Future Directions in DLS Research
As the field of nanoparticle research continues to evolve, so too does the methodology of dynamic light scattering (DLS). Understanding the future directions in DLS research is crucial not just for academic curiosity, but also for practical applications across industries. By keeping abreast of upcoming technological advancements and best practices, researchers can enhance the accuracy and effectiveness of their analyses. This section dives into key emerging trends that promise to refine DLS techniques, ensuring they remain at the forefront of nanoparticle characterization.
Technological Advancements
The realm of DLS is poised for significant transformation due to rapid technological advancements. Novel approaches and enhanced instrumentation are on the horizon that could significantly impact how measurements are made. Typically, traditional DLS setups face challenges like multiple scattering and material interactions that skew results. However, new algorithms and hardware are being integrated to mitigate these issues.
- High-speed cameras are making it possible to capture scattering patterns with much greater temporal resolution. Faster frame rates allow for better identification of particle motion over time, leading to clearer size distribution data.
- Machine learning algorithms, when applied to DLS data, can dramatically improve the interpretation of results. By harnessing computational power, these systems can uncover subtleties in data that might be missed by the human eye or traditional statistical methods.
- Microfluidics is another exciting avenue. This technology allows scientists to conduct DLS measurements on tiny sample volumes with higher precision, minimizing waste and enhancing the reliability of measurements.
- Dual-scattering detection systems can help differentiate between the light scattered by different particles in mixtures, providing a more detailed profile of sample composition.
The integration of these technologies holds the potential to not just advance the precision of DLS, but also the compatibility of this technique with various materials.
Integration with Other Characterization Techniques
In the search for a more holistic understanding of nanoparticles, the integration of DLS with other characterization methods is essential. Each analytical technique brings its own strengths, and combining them can yield richer, more comprehensive insights into particle behavior, interactions, and stability.
- Atomic Force Microscopy (AFM): By pairing DLS with AFM, researchers can obtain real-time size and shape information. While DLS gives average size distributions, AFM can visualize and quantify surface features with nanoscale resolution.
- Transmission Electron Microscopy (TEM): DLS can provide statistical size distributions, whereas TEM offers high-resolution images. Together, they can validate results and ensure that the assumptions made through DLS reflect the reality of the samples.
- Zeta Potential Measurement: Integrating zeta potential analysis with DLS enables a better understanding of the stability and interactions of nanoparticles in suspension. Knowing both size and surface charge informs decisions about formulation in applications, especially in drug delivery.
- Rheology: Merging rheological measurements with DLS could reveal insights into how nanoparticles behave under various flow conditions — vital for industries focused on mixing and processing.
"The future of DLS isn't just about refining existing methods; it's about creating a synergy between various analytical techniques that can provide a multifaceted view of nanoparticles."
The combination of DLS with complementary techniques opens doors to new discoveries and elevates the robustness of findings in nanoparticle research. As these technologies develop, they facilitate deeper investigations and more reliable outcomes, ultimately pushing the boundaries of the field.
Ending
In this article, the exploration of dynamic light scattering (DLS) has illuminated its critical role in nanoparticle characterization. Dynamic light scattering is not simply a technique; it’s a scientific lens through which researchers can examine the minute details of nanomaterials, helping them to unlock valuable insights into particle size, stability, and interactions. Understanding DLS is fundamental for students, researchers, and professionals alike, as it forms the backbone of much contemporary nanoparticle research.
Summary of Key Findings
Throughout the article, we have highlighted several key points that underscore the relevance of dynamic light scattering in the study of nanoparticles:
- Mechanism of DLS: DLS relies on the analysis of scattered light from nanoparticles, revealing information about their size and distribution in solution. The time-dependent fluctuation in intensity of scattered light is a crucial factor in the data obtained; it reflects the unique motion of particles.
- Diverse Applications: This technique isn't just confined to academic research; it has a myriad of applications ranging from biomedicine—where it's employed to characterize drug delivery systems—to environmental science for monitoring pollutants.
- Technical Aspects: The operation of DLS instruments, precise measurement techniques, and sophisticated data analysis methods are outlined, giving readers a holistic understanding of the technical framework supporting this technique.
- Challenges and Limitations: We've also delved into the hindrances faced with DLS, such as sample preparation and interference from the medium, which can skew results. These issues must be considered during practical applications.
- Future Directions: Technological advancements and the integration of DLS with other characterization methods promise to enhance nanoparticle research further, making it ever more relevant in various scientific domains.
Implications for Future Research
The implications of this article extend far beyond the basics of DLS. As research progresses, understanding the nuances of dynamic light scattering and its integration into broader scientific contexts will become vital. Here are several considerations for future research in this domain:
- Emerging Technologies: New instruments and methodologies are constantly evolving. Funders and researchers might explore the incorporation of AI algorithms to improve data analysis accuracy.
- Interdisciplinary Approaches: Combining DLS with techniques like electron microscopy or atomic force microscopy could yield multi-faceted insights, offering further layers of understanding of nanoparticle behavior.
- Impact on Industry: As DLS proves useful in industries such as pharmaceuticals and materials science, further research could focus on protocols for industrial application, improving efficiency and reliability in production processes.
- Environmental Monitoring: The pressing issues surrounding environmental pollutants highlight a need for enhanced DLS applications in ecological assessments and remediation strategies, promoting a cleaner future.
By grasping the complexities surrounding dynamic light scattering, professionals in various fields can seize the opportunity to leverage DLS effectively, paving the way for groundbreaking discoveries and innovations in nanoparticle research.