Environmental Impact of Electric Vehicles: A Comprehensive Analysis
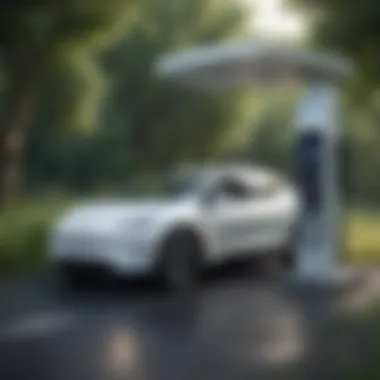
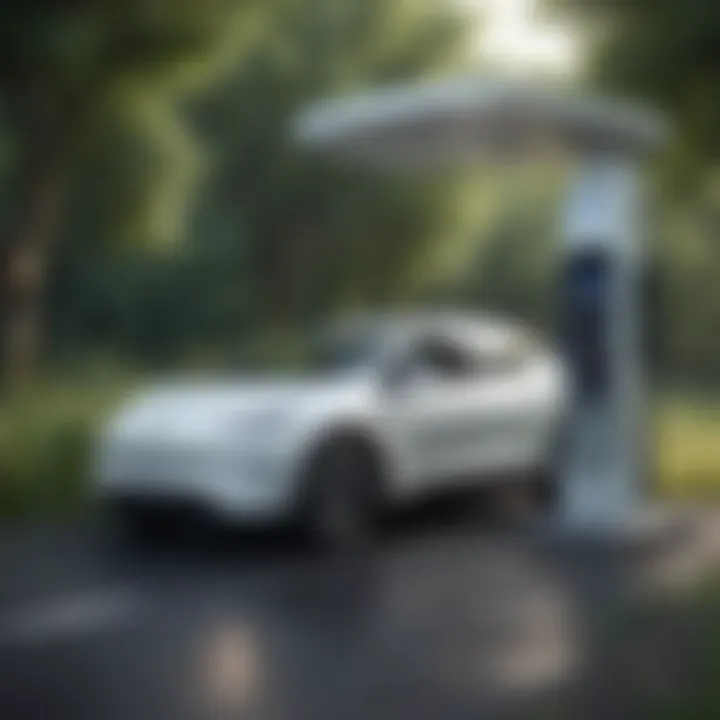
Research Overview
EVs have emerged as a pivotal element in global efforts to reduce reliance on fossil fuels and combat climate change. As nations seek to implement stricter emissions regulations, the uptake of electric vehicles is rapidly accelerating. Understanding their environmental implications is vital for policymakers, industries, and consumers alike. The findings of this research can inform strategies aimed at maximizing the ecological benefits of EVs while addressing their negative impacts.
Importance of the Research in its Respective Field
This study is important in the context of the broader discussion on sustainable transportation. It provides a nuanced view of how electric vehicles can be a part of the solution to mitigating climate change. By examining both the life cycle of EVs and their energy sources, we can identify pathways to improve EV sustainability. The implications of this research extend beyond academia, influencing legislative development and consumer choices in a rapidly evolving automotive landscape.
Methodology
Data Collection Techniques
- Literature Review: Extensive analysis of peer-reviewed articles, reports, and case studies provided a foundational understanding of the topic.
- Comparative Analysis: Evaluating various studies allowed for a broader perspective on how different factors affect the environmental footprint of EVs.
Key Parameters Examined
- Electricity Source: Examining the carbon intensity of the grid used to charge electric vehicles.
- Battery Life Cycle: Investigating the production, usage, and eventual recycling of EV batteries.
- Vehicle Disposal: Understanding the end-of-life management of electric vehicles and associated environmental implications.
Prelims to Electric Vehicles
The significance of electric vehicles (EVs) extends beyond mere innovation. They represent a shift towards sustainable transportation, responding to urgent environmental concerns. As global attention turns to climate change, the transition from fossil fuel dependency becomes critical. EVs are often lauded for their potential to reduce greenhouse gas emissions and promote cleaner air. However, understanding their complete impact requires scrutiny beyond benefits.
Electric vehicles are not only a solution but a multifaceted subject involving their production, daily operation, and end-of-life processing. Each phase presents both ecological advantages and challenges. Evaluating these dimensions is essential for policymakers, manufacturers, and consumers. The risks associated with battery production, resource extraction, and waste management are significant. Therefore, discussions surrounding EVs must encapsulate all aspects of their lifecycle.
As we embark on this exploration, the various types and market dynamics of EVs come into play. It shapes their future growth and environmental implications. Understanding how they are categorized and their current adoption rates aids in grasping their environmental significance in our evolving landscape.
In essence, this section lays the groundwork for a comprehensive evaluation of electric vehicles, highlighting the importance of informed discussions related to their ecological impact, both positive and negative.
Lifecycle Analysis of Electric Vehicles
Lifecycle analysis of electric vehicles (EVs) is crucial for understanding their overall environmental impact. This approach evaluates the environmental effects of EVs from their raw material acquisition to their end-of-life disposal. The specific elements considered in this analysis include resource use, emissions, energy consumption, and waste management. Each phase in EV lifecycle has its own unique impacts and benefits, making it necessary to scrutinize them individually.
Key benefit of lifecycle analysis is its ability to provide a comprehensive view of the EV's impact on the environment. It is not just about emissions during operation, but also encompasses critical aspects like manufacturing and raw material sourcing. This wide lens helps policymakers and stakeholders make informed decisions.
Important considerations in lifecycle analysis involve assessing trade-offs. For instance, while EVs significantly reduce tailpipe emissions, the sourcing of materials needed for batteries such as lithium and cobalt raise ecological concerns. Thus, a thorough lifecycle analysis can lead toward sustainable practices that mitigate adverse impacts while amplifying the benefits of switching to electric mobility.
Raw Material Acquisition
Lithium Mining Impacts
Lithium mining is a critical element in the production of EV batteries. The extraction process often leads to significant land degradation and water depletion. Key characteristic of lithium mining is its resource-intensive nature. It is a popular choice analyzed in this article because lithium-ion batteries are the backbone of most electric vehicles today.
A unique feature of lithium mining is its geographical concentration. Most lithium resources are located in specific regions such as the Lithium Triangle in South America. This concentration poses both advantages and disadvantages. On one hand, it allows for focused extraction efforts but on the other hand, it limits diversity in sourcing and raises geopolitical risks.
Cobalt Sourcing Issues
Cobalt presents unique challenges within the battery supply chain. Most cobalt is sourced from the Democratic Republic of the Congo, where mining operations often face ethical and environmental scrutiny. Cobalt mining has a key characteristic of being associated with serious human rights violations, making it a crucial point of discussion within this article.
Unique feature of cobalt sourcing issues includes the variability in mining practices. Some operations adhere to high environmental standards while others severely damage ecosystems. Such inconsistency complicates efforts to evaluate the environmental impact of cobalt on EV lifecycle.
Manufacturing Processes
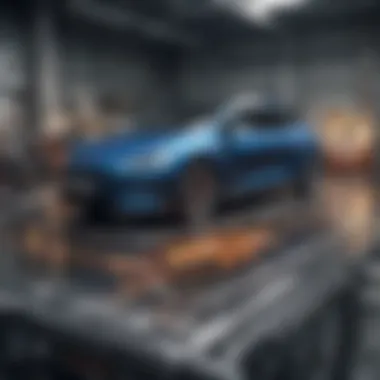
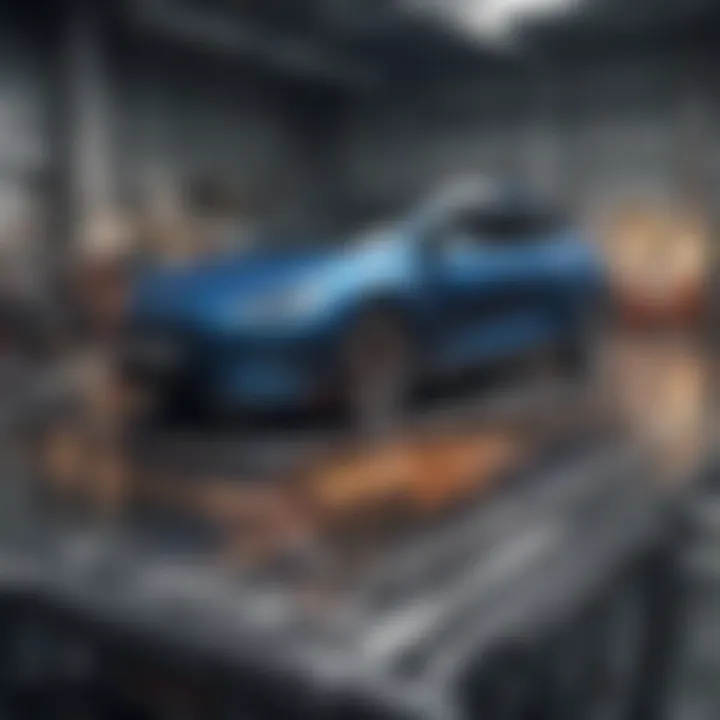
Carbon Footprint of Production
The carbon footprint involved in the production of electric vehicles is substantial. Manufacturing processes for EVs can emit high levels of greenhouse gases, particularly during the battery production phase. A key characteristic of this aspect is the energy intensity required for battery manufacturing.
This is a beneficial topic for consideration in this article because understanding the carbon footprint allows for targeted improvements. Manufacturing efficiency and alternative energy sources can reduce emissions significantly. By measuring these impacts, manufacturers can adopt more sustainable practices.
Energy Consumption During Manufacturing
Energy consumption during the manufacturing phase of electric vehicles is another crucial component. The intensity of energy required can have significant implications on the environmental footprint. A key characteristic of this topic is the reliance on non-renewable energy sources.
By evaluating this section, a clearer understanding emerges of how energy sources contribute to the overall lifecycle emissions of EVs. The use of cleaner energy in manufacturing could offer substantial long-term gains. However, a transition is often complex and requires strategic planning.
Product Use Phase
Emissions During Operation
Electric vehicles are often marketed with the promise of low operational emissions. However, they are not entirely emission-free. During their use phase, emissions vary depending on the electricity source powering the vehicle. A key characteristic of emissions during operation is their reliance on regional energy mixes.
The benefit of analyzing operational emissions is that it highlights the importance of clean energy infrastructure. Areas powered predominantly by fossil fuels may significantly diminish the environmental advantages of EVs. Therefore, building a cleaner energy grid is equally essential as promoting electric vehicle adoption.
Energy Source Variability
Energy source variability greatly impacts the environmental implications of electric vehicle operation. This variability refers to the mix of renewable and non-renewable sources used to charge EVs. A key characteristic of this issue is the difference in greenhouse gas emissions tied to different energy sources.
Highlighting energy source variability is beneficial for understanding the broader context of electric vehicles. It challenges the notion that EVs are always greener and stresses the need for a holistic approach to transportation electrification. Addressing the variances can result in more informed consumer choices and effective policy initiatives.
End-of-Life Disposal
Challenges in Battery Recycling
One significant aspect of the end-of-life analysis of electric vehicles is the recycling of batteries. Rechargeable batteries pose complex recycling challenges due to their composition. A key characteristic of these challenges is the technical difficulty of recovering valuable materials like lithium and cobalt.
The discussion on this issue is beneficial because effective recycling methods can significantly reduce environmental hazards. While progress has been made, the recycling rate of lithium-ion batteries remains low, and improving this rate is essential for a sustainable future.
Landfill and Toxicity Concerns
Landfill and toxicity concerns stem from improper disposal of electric vehicle components, particularly batteries. If batteries are not recycled effectively, they pose a serious risk to the environment. A key characteristic of this issue is the potential for toxic substances to leach into the soil and water.
This aspect is important as it underscores the necessity for robust disposal frameworks. Clear regulations and innovative disposal techniques are needed to mitigate risks associated with battery waste. Addressing these concerns paves the way for a more sustainable lifecycle for electric vehicles.
Environmental Benefits of Electric Vehicles
The discussion of electric vehicles (EVs) invariably leads to a critical examination of their environmental benefits. Understanding these advantages is essential to grasp the role EVs can play in tackling climate change and reducing pollution. This section explores how EVs can significantly impact the environment positively, focusing on three pivotal aspects: greenhouse gas emissions reduction, air quality improvement, and energy efficiency gains.
Reduction in Greenhouse Gas Emissions
One of the prominent benefits of electric vehicles is their potential to reduce greenhouse gas emissions substantially. Unlike traditional internal combustion engines, which emit carbon dioxide and other greenhouse gases during operation, EVs produce zero tailpipe emissions. The key factor influencing overall greenhouse gas reduction is the source of electricity used to charge these vehicles.
If the electricity comes from renewable energy sources, like wind or solar, the overall greenhouse gas emissions associated with operating EVs can be profoundly lower than conventional vehicles. According to studies, replacing gasoline vehicles with EVs powered by renewables could lead to reductions in carbon emissions by up to 90% over the vehicle's lifecycle. This significant decrease could greatly assist in global efforts to mitigate climate change.
"The transition to electric vehicles represents a pivotal strategy in reducing global carbon emissions and combating climate change."
Air Quality Improvement
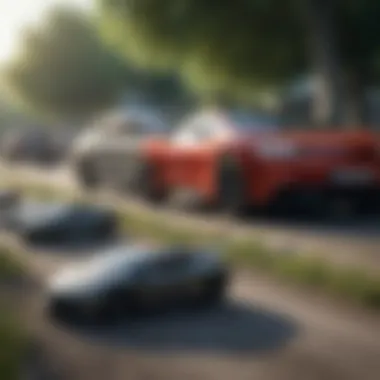
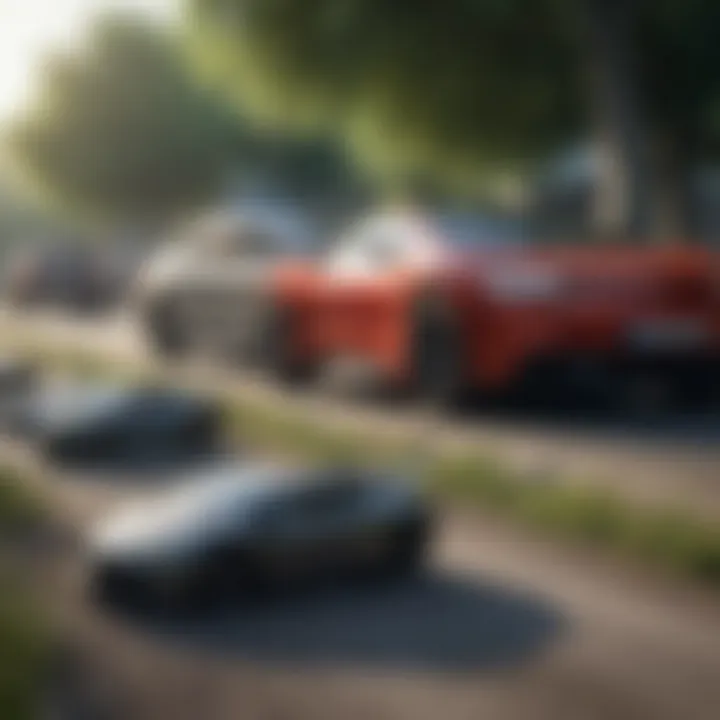
Electric vehicles also contribute to improving urban air quality. In many cities, exhaust from gasoline-powered cars is a primary source of air pollutants, including nitrogen oxides and particulate matter. These pollutants have adverse effects on human health, contributing to respiratory and cardiovascular issues.
As EV adoption increases, especially in urban settings, the reduction of these harmful emissions can lead to cleaner air. This transition not only benefits the general population but also helps protect vulnerable groups such as children and the elderly, who are often more susceptible to air quality-related health issues. Cities that promote electric vehicles can observe tangible improvements in public health and hence reduce healthcare costs over time.
Energy Efficiency Gains
Another significant benefit of electric vehicles lies in their energy efficiency. EVs convert a higher percentage of electrical energy from the grid to power at the wheels compared to conventional vehicles, which lose much of their energy in the form of heat during fuel consumption. Typically, electric vehicles can achieve efficiencies of around 60% or more, while gasoline vehicles might hover around 20% efficiency.
Improving energy efficiency is crucial as it translates into less energy consumption per mile traveled. This reduced demand can lead to a lower overall carbon footprint associated with energy production. Furthermore, the increased efficiency of electric cars means that they can leverage lower-cost energy sources, such as off-peak electricity, making them a more sustainable choice in the long run.
Adverse Environmental Impacts
Understanding the adverse environmental impacts of electric vehicles is crucial for a balanced discussion about their overall sustainability. While electric vehicles (EVs) bring certain environmental advantages, they also have significant drawbacks that merit close examination. These issues present both challenges in the current landscape and considerations for future improvements.
Resource Depletion and Ecological Damage
One of the most pressing concerns associated with electric vehicles is the depletion of resources required for their production. The mining of lithium, cobalt, and nickel not only raises ethical concerns due to labor practices but also poses risks to local ecosystems. For instance, lithium extraction in places like the Salars of South America can lead to water shortages and disruptions in biodiversity. The process often leaves large areas of land degraded, and its water use can negatively affect agriculture and living conditions for nearby populations.
Moreover, the production of batteries, which are essential for EVs, relies heavily on these minerals. As demand increases, so does the intensity of resource extraction, putting even more pressure on the environment. This leads to a feedback loop where the rise in electric vehicle popularity intensifies the very ecological damage that sustainability efforts aim to mitigate.
Battery Production and Recycling Risks
Battery production is not only resource-intensive but also includes numerous environmental risks. The chemical processes involved in manufacturing batteries generate hazardous waste that must be managed properly to avoid soil and water contamination. Furthermore, as the electric vehicle market grows, the demand for reliable recycling methods becomes more critical. Presently, there are significant challenges in battery recycling, which includes the inefficient recovery of valuable materials such as lithium and cobalt.
This inefficiency can lead to increased waste, with used batteries ending up in landfills, where they may leak toxic substances into the environment. As a result, this situation creates a paradox. The technology designed to reduce emissions and reliance on fossil fuels can contribute to pollution if end-of-life management is not addressed adequately.
"We must tackle the paradox of sustainable technology potentially becoming the source of new environmental issues."
Transportation Infrastructure Strain
The increased adoption of electric vehicles also puts strain on existing transportation infrastructures. Charging stations are not as ubiquitous as gasoline stations, leading to gaps in availability, particularly in rural areas. The imbalance can cause grid overload in urban regions where EV uptake is high. Consequently, this may result in a negative impact on grid stability and reliability, ultimately leading to higher emissions if fossil fuels are needed to compensate for the increased demand.
Additionally, as cities adapt to the shift towards electric vehicles, they must consider the implications for urban planning. The urgency of installing new charging infrastructure may push projects that do not prioritize sustainability or environmental conservation, thereby exacerbating land use conflicts. Investments need to balance technological progress with ecological stewardship to avoid repeating mistakes of the past.
Comparative Analysis with Internal Combustion Vehicles
The comparison between electric vehicles (EVs) and internal combustion vehicles (ICVs) is essential for understanding their impact on the environment. This analysis sheds light on how each type of vehicle contributes to emissions, resource usage, and overall sustainability. As the automotive industry shifts toward electrification, this comparative study helps in assessing the true ecological footprint of EVs versus traditional vehicles.
Lifecycle Emission Comparisons
Lifecycle emission comparisons provide a comprehensive view of the carbon footprint associated with both EVs and ICVs. From raw material extraction through to manufacturing, usage, and disposal, both types of vehicles have distinct paths, resulting in differing emissions profiles.
- Raw Material Extraction: The initial phase for ICVs is the extraction and processing of petroleum. This process directly affects greenhouse gas emissions and can cause ecological harm. In contrast, EVs rely on materials like lithium and cobalt, which also present challenges. However, the emissions linked to generating electricity for EVs can be significantly lower if renewable energy sources are utilized.
- Manufacturing Process: The production of vehicles involves energy that also contributes to emissions. Studies indicate that producing an EV can emit more CO2 than an ICV due to battery manufacturing. Yet, this gap can be offset over the vehicle's lifetime through lower operational emissions.
- Operational Emissions: Once on the road, the operational emissions are crucial. ICVs typically burn fossil fuels, releasing significant amounts of CO2 and other pollutants into the atmosphere. For EVs, emissions largely depend on the energy mix used for charging. In regions with cleaner grids, EVs can operate with much lower emissions.
- End-of-Life Emissions: Disposal of both types of vehicles presents further challenges. ICVs result in waste that can be hazardous, while the recycling of EV batteries is developing yet under scrutiny for efficiency and environmental impact.
The above factors indicate that while EVs may initially appear to have a higher footprint, they dramatically reduce emissions during their operational phase, especially when charged from renewable resources.
Resource Usage and Sustainability
Resource usage and sustainability represent vital elements in evaluating the environmental impact of both vehicle types. The sustainability of raw materials must be addressed thoroughly.
- Resource Consumption: ICVs predominantly rely on finite fossil fuels, which are unsustainable in the long term. Electric vehicles, on the other hand, rely on metals that are recyclable, yet the extraction of these metals can also deplete resources and damage ecosystems.
- Economic sustainability: Transitioning to EVs represents a shift towards a more sustainable economic model. The increased demand for electric vehicles prompts innovations in battery technology, recycling methods, and energy solutions, potentially leading to reduced costs and impacts over time.
- Renewable Energy Potential: Integrating renewable energy with electric vehicle operations enhances sustainability. As the world moves towards greener energy sources, the advantages of EVs expand. They can play a crucial role in energy storage and grid stability, especially when charged during peak renewable production hours.
With careful planning and innovative technology, the shift towards electric vehicles can indeed foster a more sustainable future in transportation.
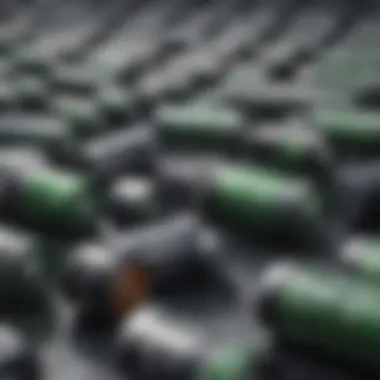
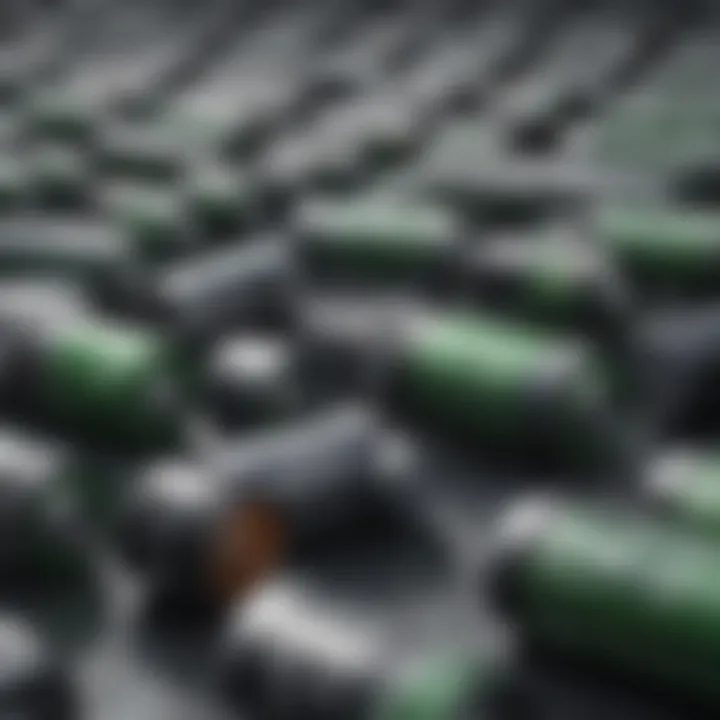
Policy Implications and Regulatory Frameworks
Government Incentives and Subsidies
Government incentives and subsidies have become pivotal in accelerating the electrification of transportation. These financial mechanisms can significantly lower the purchase price of electric vehicles, making them more appealing to consumers. For instance, the Federal Tax Credit in the United States provides a substantial reduction in taxes for individuals who purchase certain electric vehicles. Additionally, many state governments offer incentives in the form of rebates, reduced registration fees, or tax exemptions.
The effectiveness of these incentives is increasingly documented. They not only spur initial adoption but also foster a market that encourages competition among manufacturers. By stimulating demand, these incentives drive innovations in battery technology and manufacturing processes, enhancing energy efficiency and reducing lifecycle emissions.
However, it is essential to consider the long-term impacts of these subsidies. Continuous reliance on government aid can raise questions about the sustainability of the EV market if these incentives diminish or are removed. Therefore, it is necessary for policymakers to assess the balance between financial support and the eventual goal of an economically independent EV industry.
Environmental Regulations and Standards
Environmental regulations and standards serve as a backbone for ensuring that electric vehicles contribute positively to the environment. These regulations are designed to limit emissions generated during the manufacturing process and to mandate certain performance standards for vehicle operations. For example, the EPA (Environmental Protection Agency) in the United States enforces standards that dictate the emissions levels producers must meet, which ultimately influences manufacturing practices.
Moreover, these regulations extend beyond just tailpipe emissions. They also encompass battery production and disposal processes. Standards dictate how producers manage hazardous materials used in batteries, thereby alleviating potential ecological harms from improper disposal methods. This regulatory framework encourages manufacturers to invest in recycling technologies and more sustainable materials, thereby targeting challenges related to battery life cycle management.
The integration of environmental considerations into policy ensures that the shift towards electric mobility does not come at the cost of other critical ecological factors. Policymaking in this realm must remain dynamic and adaptable, considering new scientific findings and technological advances. As regulations evolve, they can help drive the shift towards cleaner energy, ultimately guiding the industry toward a more sustainable future.
Future Directions and Technological Innovations
The future of electric vehicles (EVs) lies heavily influenced by advancements in technology and integration with sustainable energy sources. This section will explore how emerging technologies may reshape the landscape of EVs and their environmental impact. As demands increase, understanding these innovations becomes crucial for mitigating adverse effects while maximizing benefits. Consideration of battery enhancements and renewable energy utilization plays a significant role in shaping effective environmental policies surrounding EV usage.
Advancements in Battery Technology
Battery technology is at the heart of electric vehicle performance and sustainability. Improvements in battery capacity, efficiency, and lifespan can significantly mitigate the environmental concerns associated with EVs. Current lithium-ion batteries, commonly used in electric vehicles, have limitations, including resource-intensive production and potential for toxic waste at the end of their life cycle.
Key advancements include:
- Solid-state batteries: These offer higher energy density and reduced risk of fire compared to traditional batteries. Solid-state technology could lead to longer ranges and faster charging times, making electric vehicles more appealing to consumers.
- Recycling improvements: Enhanced recycling technologies can reclaim valuable materials like lithium and cobalt. This reduces dependence on mining and lowers ecological damage, making the entire lifecycle of the battery more sustainable. Many companies are investing in pilot projects to improve recovery rates.
- Battery management systems: These systems optimize charging and discharging cycles, extending the life of batteries and enhancing overall performance. Data from these systems can inform users on efficient usage, further reducing environmental footprints.
By focusing on advancements in battery technology, electric vehicles could become a more sustainable option in the transportation industry, alleviating some environmental concerns currently attributed to their lifecycle.
Potential of Renewable Energy Integration
Integration of renewable energy sources directly into electric vehicle infrastructure can yield significant environmental benefits. When EVs are powered by clean energy, their overall carbon footprint diminishes markedly. This shift strives towards a truly sustainable transportation system. The effective implementation of renewable energy hinges on various aspects:
- Solar charging stations: Utilizing solar panels to charge electric vehicles can reduce reliance on fossil fuels. This model promotes the use of sustainable energy, minimizing emissions associated with the electrical grid.
- Smart grids: These improve energy management and enhance the ability to harness diverse energy sources including wind and solar. Smart grids can balance loads more efficiently and facilitate the integration of Electric Vehicle Supply Equipment (EVSE) that utilizes renewable energy.
- Vehicle-to-grid solutions: This innovative approach allows electric vehicles to supply energy back to the grid. It not only supports energy storage but also incentivizes EV owners to participate in peak demand management, encouraging responsible energy usage.
Incorporating renewable energy into the electric vehicle ecosystem is vital for maximizing sustainability. These integrations can transform how electric vehicles influence energy consumption, driving a progressive shift toward greener transportation practices.
"The transition to electric vehicles represents not just a change in technology, but a profound shift in how we conceive energy use, infrastructure, and environmental sustainability."
While the path forward is filled with challenges, the ongoing innovations in battery technology and renewable energy integration provide hope for a greener future. Continued research and investments in these areas will be critical for the holistic environmental progress of electric vehicles.
Finale and Takeaways
The examination of electric vehicles’ environmental impact reveals critical insights into both their potential and limitations. This conclusion synthesizes the findings presented throughout the article, emphasizing how electric vehicles are positioned at a complex intersection of technological advancement and environmental sustainability.
Summary of Key Findings
Electric vehicles have showcased substantial benefits, particularly in reducing greenhouse gas emissions when compared to traditional internal combustion vehicles. The life cycle analysis indicates that emissions during the use phase are significantly lower, primarily due to the absence of tailpipe emissions. However, it is essential to acknowledge that the environmental benefits can vary dramatically based on the energy sources utilized for electricity generation. For instance, regions that depend heavily on fossil fuels for electricity may see diminished advantages in emission reductions.
Moreover, the resource extraction for batteries, like lithium and cobalt, poses serious environmental and ethical challenges. The ecosystem degradation from mining activities and the social implications of sourcing these materials raise questions about the overall sustainability of electric vehicles. Additionally, battery disposal and recycling processes are not yet optimized, leading to potential hazards if not managed properly.
Implications for Future Research and Policy
Looking forward, further investigation into enhancing the sustainability of electric vehicles is crucial. Improving battery technology to increase lifespan and recyclability forms a key area of focus. Investments in renewable energy sources for electricity generation can also help improve the net environmental benefit of electric vehicles.
Policy implications are multifaceted. Governments should consider implementing stricter regulations on battery production processes, promoting responsible sourcing of raw materials. Furthermore, supporting innovations in recycling technologies could mitigate some of the adverse impacts associated with the disposal of electric vehicle batteries. As the market evolves, ongoing research will be necessary to address these emerging challenges and to guide the development of sustainable transportation solutions.