Epigenetic Technology: The Future of Gene Regulation
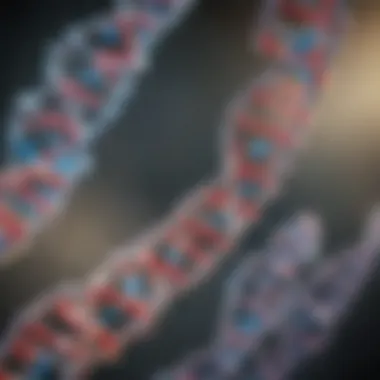
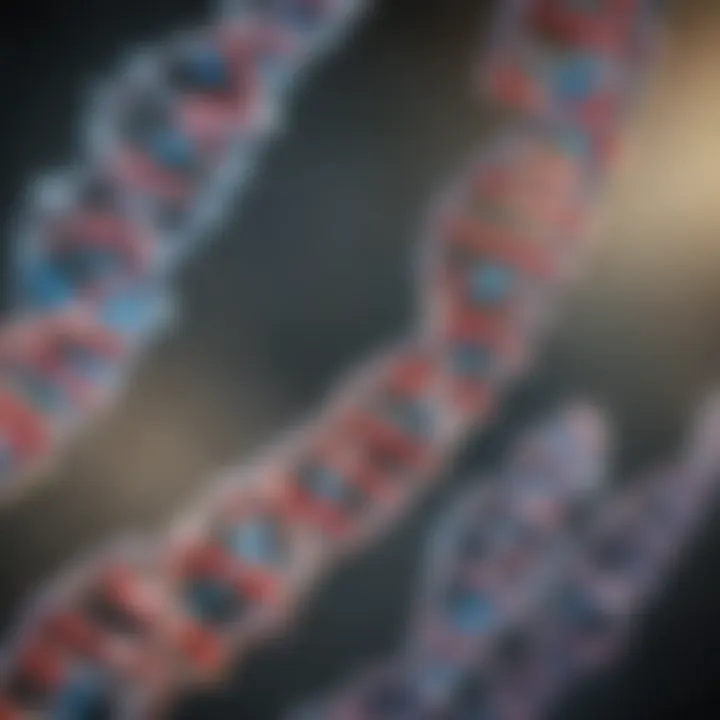
Intro
Epigenetic technology represents a transformative frontier in genetics, emphasizing the intricate mechanisms that regulate gene expression without altering the underlying DNA sequence. This area of research has garnered significant attention due to its profound implications for biotechnology and medicine. By understanding how processes like DNA methylation and histone modification influence cellular function, researchers can develop targeted therapies for various diseases, enhance agricultural practices, and shed light on fundamental biological processes.
In recent years, advancements in gene-editing techniques have offered tools to manipulate the epigenome more precisely. This promises to unlock new pathways for therapeutic intervention, yet it also raises ethical considerations that cannot be ignored. The exploration of epigenetic technology is not merely an academic pursuit; it is a critical inquiry into the future of human health, agriculture, and our understanding of life itself.
As we dive deeper into this field, we will outline key findings on gene regulation through epigenetic mechanisms, discuss the methodologies employed in current research, and highlight potential applications and ethical implications.
Preface to Epigenetics
Epigenetics has emerged as a pivotal field in understanding gene expression and regulation. This subject examines how external and environmental factors can influence gene activity without altering the underlying DNA sequence. The implications of epigenetic modifications are far-reaching, affecting areas such as medicine, agriculture, and biotechnology. In this article, we will investigate the complex mechanisms of epigenetics, highlighting its significance across various domains.
Defining Epigenetics
Epigenetics refers to changes in gene function that do not involve alterations to the DNA sequence itself. These modifications can affect how genes are turned on or off and can result in phenotypic changes. Key mechanisms of epigenetic regulation include DNA methylation, histone modification, and the action of non-coding RNAs. Understanding these processes is essential for researchers and practitioners focused on the intricate details of gene regulation and its applications.
Historical Perspective
The roots of epigenetics can be traced back to early genetic studies, when scientists noticed that identical twins could exhibit different traits despite sharing the same DNA. This observation prompted further investigation into the role of factors beyond genetic sequence. In the 1940s, the concept of epigenetics began to gain traction as researchers explored how environmental influences impact gene expression. By the 21st century, advances in technology allowed for in-depth study of epigenetic modifications, leading to new insights into their role in development, disease, and inheritance. Today, the field continues to expand, informing a diverse range of scientific disciplines and fueling innovation in medical and agricultural practices.
Mechanisms of Epigenetic Regulation
The mechanisms of epigenetic regulation play a crucial role in governing gene expression without altering the underlying DNA sequence. Understanding these mechanisms is essential for advancements in biotechnology and medicine. It opens pathways for innovative treatments and enhances our knowledge of cell function and development. They provide a framework for interpreting complex biological phenomena, allowing scientists to target specific genes in various applications. This article elaborates on the diverse processes involved in epigenetic regulation, focusing on DNA methylation, histone modification, and non-coding RNAs.
DNA Methylation
Role in Gene Silencing
DNA methylation is a well-studied mechanism that involves adding a methyl group to the DNA molecule, primarily at cytosine bases. This process can effectively lead to gene silencing. By inhibiting the binding of transcription factors and attracting repressive proteins, methylation can prevent gene expression. This characteristic makes DNA methylation a popular topic in the understanding of gene regulation.
One unique feature of DNA methylation is its reversible nature. This allows for potential therapeutic interventions where specific genes can be activated or silenced by manipulating methylation patterns. However, there are also disadvantages, such as the difficulty in targeting specific regions of the genome accurately without affecting other areas.
Impact on Development
The impact of DNA methylation on development is profound. It shapes processes such as cell differentiation by regulating the expression of key developmental genes. This characteristic makes the discussion of its role in development especially important for understanding congenital diseases and developmental disorders.
The unique feature of DNA methylation in developmental contexts is its dynamic regulation, which can adapt to environmental stimuli. This flexibility is beneficial for evolutionary adaptations but can also lead to challenges in ensuring proper gene expression throughout development.
Histone Modification
Types of Modifications
Histone modification involves the addition or removal of chemical groups to histone proteins around which DNA winds. This mechanism encompasses various modifications such as acetylation, methylation, and phosphorylation. Each type of modification can either promote or inhibit gene expression. Histone modifications are a beneficial and popular subject because they provide a deeper understanding of chromatin dynamics.
The unique feature of histone modifications is the combinatorial nature of these changes. Different patterns can lead to diverse outcomes in gene regulation, which highlights the complexity of epigenetic control. However, this complexity also poses challenges for researchers aiming to decipher the specific roles of individual modifications.
Effects on Chromatin Structure
Histone modifications significantly affect chromatin structure, influencing its accessibility for transcription. When histones are acetylated, the chromatin becomes more relaxed, facilitating gene expression. This characteristic is key to understanding how transcriptional activation occurs in different cellular contexts.
The unique aspect of these effects is their role in establishing distinct chromatin states in different cell types. This has implications for understanding tissue-specific gene regulation and the maintenance of cell identity. However, the intricacy of how these modifications interact with one another can complicate efforts to predict gene expression outcomes accurately.
Non-coding RNAs
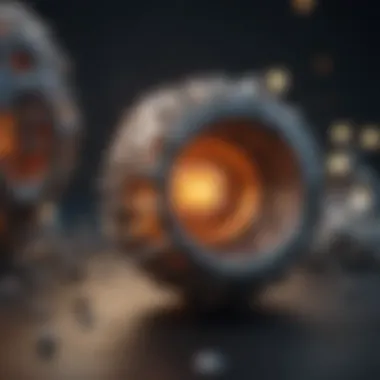
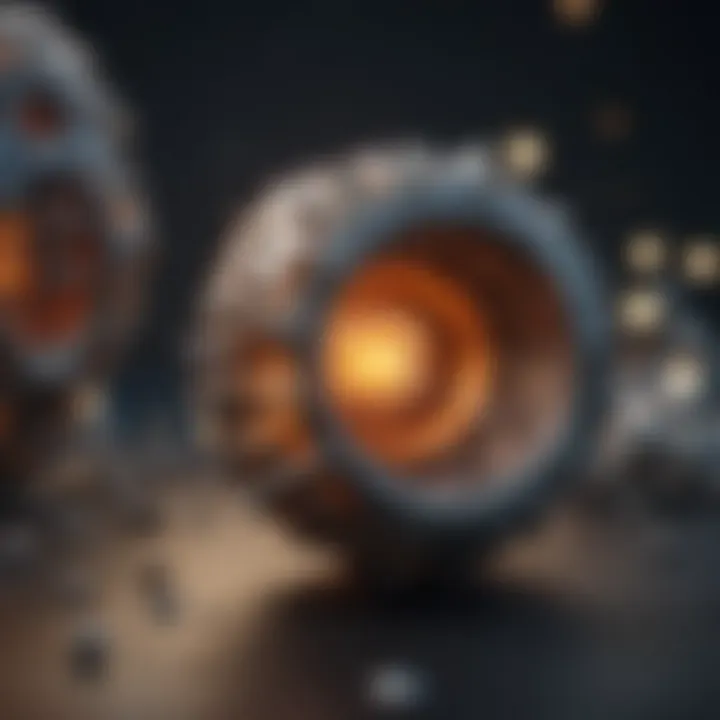
Role in Gene Regulation
Non-coding RNAs, particularly microRNAs and long non-coding RNAs, play crucial roles in regulating gene expression. They can modulate gene activity by binding to messenger RNAs (mRNAs) and suppressing their translation. This characteristic illustrates how non-coding RNAs add another layer to gene regulation, making them essential for understanding cellular processes.
A unique feature of non-coding RNAs is their potential for widespread regulatory networks. Their interactions can influence multiple targets simultaneously, allowing for coordinated responses to internal or environmental signals. However, this broad action can make it difficult to pinpoint specific gene regulatory roles of individual non-coding RNAs.
Interactions with Other Molecules
Interactions between non-coding RNAs and other biomolecules, including proteins and DNA, are critical for understanding epigenetic regulation. These interactions can stimulate or inhibit various biological pathways, adding further complexity to gene expression control.
The key characteristic of these interactions is their ability to create intricate signaling networks. This aspect is beneficial in developing strategies for gene therapy, as targeting specific interactions may yield significant therapeutic outcomes. However, the numerous interactions can also pose a challenge to designing precise interventions.
Innovations in Epigenetic Technology
Innovations in epigenetic technology represent a critical juncture in our understanding of gene regulation. The significance of this area lies in its potential to redefine therapeutic strategies and enhance biotechnological applications. With precise control over gene expression, researchers can manipulate biological pathways, yielding benefits that stretch across various fields including medicine and agriculture. As these technologies evolve, they present both opportunities and challenges that must be navigated with care.
CRISPR-based Epigenome Editing
Mechanisms of Action
CRISPR-based epigenome editing is a groundbreaking method used to modify gene expression without altering the DNA sequence itself. This innovative approach leverages the natural defense mechanisms of bacteria against viral infection, utilizing CRISPR-Cas systems.
One of its key characteristics is the ability to target specific genes with remarkable precision. By employing guide RNAs, researchers can direct epigenetic modifiers, such as DNA methyltransferases or histone deacetylases, to desired genomic locations. The unique feature of this system lies in its flexibility; it can be adapted to achieve either gene activation or repression, depending on the needs of the study. The advantages of CRISPR-based epigenome editing include a high degree of specificity and the potential for multiplexing, whereby multiple genes can be targeted simultaneously. However, careful consideration of off-target effects is essential to ensure the accuracy and safety of this technology.
"The beauty of CRISPR-based epigenome editing is its potential to offer precise control over gene expression, opening doors to innovative therapeutic strategies."
Applications in Research
The applications of CRISPR-based epigenome editing in research are vast and transformative. This technique allows scientists to explore gene function in unprecedented ways, aiding in the understanding of complex diseases such as cancer and neurodegenerative disorders. It has become a popular choice among researchers due to its efficiency and ease of use.
One unique aspect of this method is its capacity to create dynamic models of gene regulation. As a result, researchers can investigate how epigenetic modifications affect cellular behaviors over time. For instance, the reversible aspects of these modifications enable the monitoring of cellular responses to environmental changes or drug treatments. While the benefits are clear, challenges such as potential ethical concerns and the need for extensive validation studies must be addressed as the technology advances.
Next-Generation Sequencing
Techniques Used
Next-generation sequencing (NGS) represents a significant advance in genetic analysis, enabling large-scale sequencing of entire genomes rapidly and cost-effectively. The techniques used in NGS, such as Illumina sequencing and sequencing by synthesis, allow for the analysis of epigenetic modifications at an unprecedented level of detail.
One of the defining characteristics of NGS technologies is their ability to generate vast amounts of data from a single experiment. This feature makes them a favored choice in epigenetic studies, as they facilitate the comprehensive mapping of methylation patterns across the genome. The advantages of NGS include high throughput and accuracy, though the vast data generated presents its own set of challenges, especially in data management and analysis.
Data Interpretation Challenges
While next-generation sequencing offers powerful insights, it also brings significant challenges in data interpretation. With the high volume of data produced, accurately distinguishing relevant biological signals from background noise becomes complex. This issue necessitates sophisticated bioinformatics tools and expertise to ensure meaningful results can be extracted.
The data interpretation challenges serve as a reminder that while technologies advance, the need for rigorous analytical frameworks remains. This aspect is crucial for ensuring the reliability of findings in epigenetic research. As practices evolve, fostering an interdisciplinary approach that combines biology with computational science is essential for overcoming these hurdles and pushing the boundaries of epigenetic research.
Applications of Epigenetic Technology
The applications of epigenetic technology are diverse and impactful, revealing the potential for innovation in various fields. This section discusses its significance in both medical and agricultural sectors, emphasizing how these applications can enhance our understanding and manipulation of biological systems.
Medical Applications
Cancer Treatment
Cancer treatment through epigenetic technology is a crucial area of exploration. Epigenetics plays a role in the regulation of gene expression, which can influence cancer development. One key aspect of cancer treatment is targeting epigenetic modifications. By reversing abnormal DNA methylation patterns, researchers can reactivate tumor suppressor genes. This approach represents a promising strategy in precision medicine, allowing for tailored therapies based on the specific epigenetic profiles of tumors.
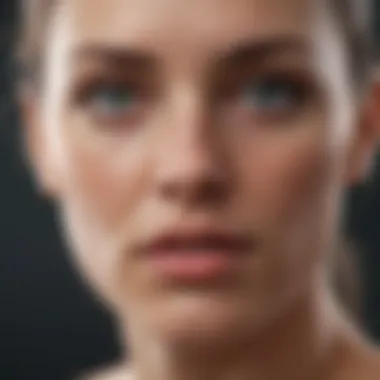
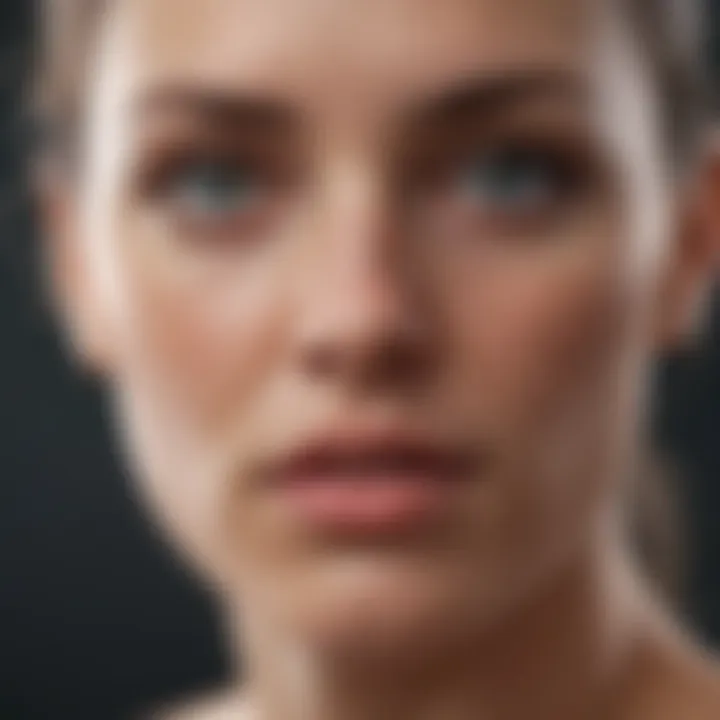
The significant characteristic of epigenetic cancer treatment is its ability to not just attack cancer cells directly but also to modify the underlying cellular environment that allows cancer to thrive. This dual approach makes it a popular choice in modern oncology.
However, the unique feature of this treatment is that it can sometimes lead to unintended consequences. For instance, demethylating agents may inadvertently activate oncogenes, which can complicate treatment outcomes. Thus, while effective, the long-term effects of these treatments require careful study and monitoring.
Gene Therapy
Gene therapy represents another crucial application of epigenetic technology. This approach involves the introduction, removal, or alteration of genetic material within a person’s cells to treat disease. In the context of epigenetics, gene therapy can modify epigenetic marks to silence harmful genes or activate beneficial ones.
The key characteristic of gene therapy is its potential for long-lasting effects. Unlike traditional gene treatments that may provide temporary relief, epigenetic modifications can lead to sustained changes in gene expression. For this reason, it is viewed as a revolutionary strategy with immense therapeutic promise.
A notable feature of gene therapy is its specificity. This technology can be designed to target specific cells or tissues, reducing the risk of side effects compared to conventional therapies. Yet, challenges exist in ensuring accurate delivery and control of epigenetic changes. Off-target effects could pose risks, potentially resulting in unexpected health issues.
Agricultural Biotechnology
Crop Improvement
In agricultural biotechnology, epigenetic technology plays a vital role in crop improvement. By understanding and manipulating epigenetic mechanisms, scientists can enhance desirable traits such as yield, disease resistance, and nutritional content. This specific aspect of crop improvement makes it a strong focus in the quest for sustainable farming practices.
A significant characteristic of this application is its potential to produce crops that are more resilient to pests and environmental stresses. This is especially beneficial in the context of a changing climate and growing global population.
The unique feature of this approach is that it may provide benefits without altering the underlying DNA sequence of crops. This helps improve public acceptance regarding genetically modified organisms (GMOs). However, ecological impacts and regulatory challenges should be considered carefully, as unintended consequences may still occur.
Stress Resilience
Stress resilience in crops is another important application stemming from epigenetic technology. This involves enhancing a plant's ability to endure environmental stresses such as drought, salinity, and temperature extremes.
The key characteristic of this application is that it could help secure food supply chains against climate change. By developing stress-resilient crops, farmers can maintain production levels even under adverse conditions.
A unique feature of promoting stress resilience through epigenetics is its adaptability. It allows for the expression of traits in response to changing environmental conditions. However, the effectiveness of such traits may vary depending on the specific context and environment in which they are applied. Further research is needed to solidify understanding in this area.
Epigenetic technology holds transformative potential, not only in medicine but also in agriculture, by redefining how we approach disease treatment and crop production.
Ethical Considerations
As epigenetic technology progresses, its implications on society and individual rights come to the forefront. Ethical considerations shape how research and applications are conducted. These aspects must be scrutinized to ensure responsible innovation in the field of gene regulation. Understanding these issues is crucial for both scientists and the public, ensuring that advancements benefit society without compromising individual rights or values.
Privacy Issues in Genetic Data
The rise of epigenetic technology has brought significant concerns regarding privacy. Genetic data is sensitive. It can reveal a lot about a person, including predispositions to various health conditions and potential behavioral traits. As such, issues surrounding data protection require rigorous attention.
The importance of informed consent cannot be overstated. Individuals must be aware of how their genetic information will be used, shared, and stored. This also includes clarity on the lifespan of the data storage. Without stringent guidelines, misuse of genetic data can occur, leading to unauthorized disclosures. The implications can affect insurance, employment, and personal relationships.
Data anonymization is one potential safeguard. However, it is not foolproof. Advances in data analysis could lead to re-identification, even with ostensibly anonymized datasets. Researchers and institutions must prioritize transparency in handling genetic data to build trust with participants.
Potential for Misuse
The potential misuse of epigenetic technology presents another significant concern. While the technology can offer remarkable advancements in medicine and agriculture, it also opens a door to unethical practices. Genetic editing, whether intentional or accidental, carries risks that could lead to unanticipated consequences.
For instance, the ability to manipulate gene expression could lead to enhancement technologies that prioritize certain traits over others. This could create ethical dilemmas regarding equality and access. If only certain segments of the population can utilize these technologies, it may widen social gaps and inequalities.
Risks include:
- Discrimination: Individuals might face discrimination based on their genetic makeup.
- Eugenics: Misguided applications could encourage eugenics, undermining societal values of diversity and inclusion.
- Health Risks: There may be unintended health effects from using epigenetic technologies, which are not yet fully understood.
To address these issues, a regulatory framework must be established. This framework should involve diverse stakeholders, including ethicists, scientists, and policymakers. Continuous dialogue and public engagement are essential in monitoring the implications of these technologies on society.
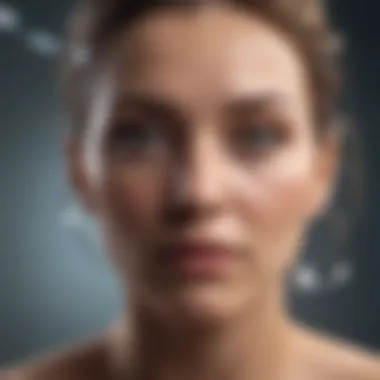
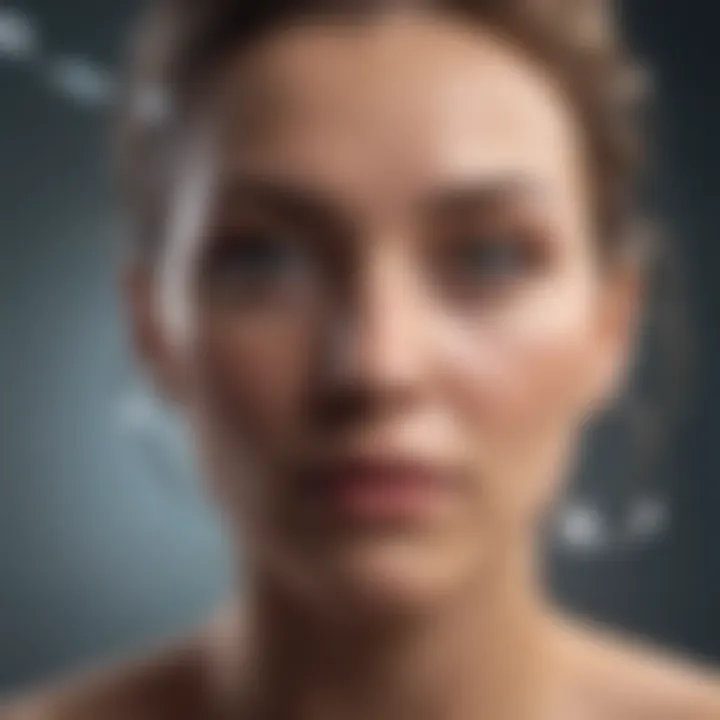
Challenges in Epigenetic Research
The investigation into epigenetic technology presents various complexities that researchers must navigate. Understanding the challenges in epigenetic research is crucial for its advancement and application. Two significant challenges are technical limitations and the standardization of methods. Each of these issues plays a vital role in the progress of epigenetic studies, influencing both the reliability and applicability of findings in varied fields, including medicine and agriculture.
Technical Limitations
Epigenetics is a rapidly evolving field, yet it is not without its technical hurdles. Researchers frequently confront obstacles related to the techniques utilized for studying epigenetic modifications. For example, methods used to analyze DNA methylation and histone modifications can vary significantly in sensitivity and specificity. This inconsistency can lead to challenges in reproducibility, making it difficult for scientists to confirm their results.
Furthermore, the complexity of epigenetic regulation requires advanced technologies that are still in developmental phases. Techniques such as single-cell sequencing and high-throughput screening are promising but may not always accurately reflect the dynamic nature of epigenetic changes within living organisms.
Consequently, these limitations hinder comprehensive understanding and translation of epigenetic research into practical applications like gene therapies or targeted treatments.
Standardization of Methods
The lack of standardized methods across research labs is another significant challenge in epigenetic research. Standardization is essential for ensuring that findings can be compared and verified. Currently, various protocols exist for assessing epigenetic phenomena. This inconsistency can result in significant variations in data interpretation and outcomes.
Establishing unified guidelines would enhance data reliability and promote collaboration across institutions. Efforts are being made within the scientific community to develop such standards. Organizations and researchers advocate for best practices in experimental design, data collection, and analysis.
Clear guidelines for methodology will facilitate collaboration and improve the confidence in epigenetic research outcomes, promoting discoveries that can lead to innovative applications.
By addressing these challenges, the field can progress toward more robust and impactful research. As the technology advances and collaborative standards become more prevalent, the potential for epigenetics to influence various sectors, including healthcare and environmental management, will undoubtedly expand.
Future Directions in Epigenetics
The exploration of future directions in epigenetics is of utmost significance in understanding how gene regulation can evolve with technological advancements. As research progresses, several key areas emerge as focal points for innovation. Future endeavors could potentially reshape the landscape of biotechnology and medicine, addressing both existing challenges and the nascent possibilities in gene regulation.
Interdisciplinary Research Approaches
Interdisciplinary approaches are vital for enhancing our understanding of epigenetics. By integrating insights from fields such as bioinformatics, molecular biology, and systems biology, researchers can develop more comprehensive frameworks to study epigenetic mechanisms. For example, computational models can analyze large datasets from epigenomic studies, unveiling patterns that may not be immediately obvious. Additionally, collaboration between biologists and computer scientists can help in deciphering the complex interactions between epigenetic factors and environmental influences.
Benefits of interdisciplinary research include:
- Holistic Understanding: Combining diverse perspectives fosters a more comprehensive understanding of gene regulation.
- Enhanced Analytical Techniques: Utilizing advanced modeling and analysis techniques from various disciplines can lead to better data interpretation.
- Innovative Solutions: Collaboration often leads to creative solutions to persistent challenges in epigenetics.
Long-term Impact on Human Health
The long-term impact of epigenetic research on human health cannot be overstated. As the field progresses, one crucial area of focus is the role epigenetics plays in chronic diseases such as cancer, diabetes, and neurodegenerative disorders. Incorporating knowledge from epigenetic patterns, researchers aspire to produce targeted preventative strategies and therapies tailored to individual genomic profiles.
Future advancements in epigenetic technology could enable personalized medicine to reach its full potential. This involves aligning treatment modalities not only with genetic variations but also with epigenetic modifications. For instance, identifying specific methylation patterns associated with disease risk might prompt earlier interventions.
"Understanding epigenetic modifications can lead to breakthroughs in prevention and treatment strategies for various diseases."
Moreover, public health initiatives might integrate epigenetic information to better address environmental health issues. Education about epigenetic influences could empower individuals to make informed lifestyle choices that promote healthier gene expression outcomes over time.
In summary, the future landscape of epigenetics rests upon interdisciplinary collaboration and a focus on long-term public health outcomes. By addressing these directions, the potential for advancing our understanding of gene regulation is vast, shaping new frontiers in both science and medicine.
Closure
The conclusion serves as a pivotal element in the discourse on epigenetic technology. It summarizes the critical insights gleaned from the exploration of gene regulation mechanisms and their applications across various fields. Understanding the role of DNA methylation, histone modifications, and the innovative approaches such as CRISPR-based gene editing creates a robust framework for future research and applications. Additionally, it highlights the ethical implications that accompany these advancements, reinforcing the responsibility of researchers and practitioners in this space.
Summary of Key Points
- Epigenetic Mechanisms: The article detailed key mechanisms of epigenetic regulation such as DNA methylation and histone modification, and their roles in gene expression. These processes are fundamental in determining how genes are expressed without altering the underlying DNA sequence.
- Technological Innovations: Innovations like CRISPR-based epigenome editing offer unprecedented control over gene regulation. The capabilities of next-generation sequencing have also transformed our understanding of epigenetics, allowing more precise data collection and analysis.
- Applications: The applications discussed cover significant areas, including medical fields such as cancer treatment and biotechnology for agricultural enhancement. These applications demonstrate the transformative potential of epigenetic technologies in addressing real-world problems.
- Ethical Considerations: Ethical issues surrounding genetic data privacy and the potential for misuse of epigenetic information remain critical discussions. It is imperative to establish clear guidelines and regulations as the technology continues to evolve.
- Challenges and Future Directions: The article also highlighted the challenges in epigenetic research, such as technical limitations and the need for standardized methods. Future research must prioritize interdisciplinary approaches to further unravel the complexities of gene regulation.
The Importance of Ongoing Research
Ongoing research in epigenetics is essential for multiple reasons. Firstly, it expands our understanding of how environmental factors influence gene expression and cellular behavior. A more profound comprehension of these mechanisms could lead to breakthroughs in personalized medicine and tailored therapies, allowing interventions that are specific to an individual’s genetic makeup.
Moreover, the continual advancement of epigenetic technologies may pave the way for innovative treatments in various diseases beyond cancer, such as neurological disorders and metabolic conditions. By exploring the interplay between genetics and environment, researchers can develop multifaceted approaches to health that consider both biological and external influences.
Ultimately, the pursuit of knowledge in this domain cultivates a culture of scientific inquiry that is critical in our quest to harness the benefits of epigenetic technology. As we move forward, the combination of rigorous research, ethical considerations, and interdisciplinary collaboration will be key in shaping the future trajectory of this field.