Electrophoresis Process: An In-Depth Exploration
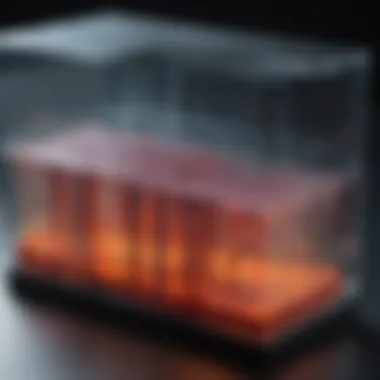
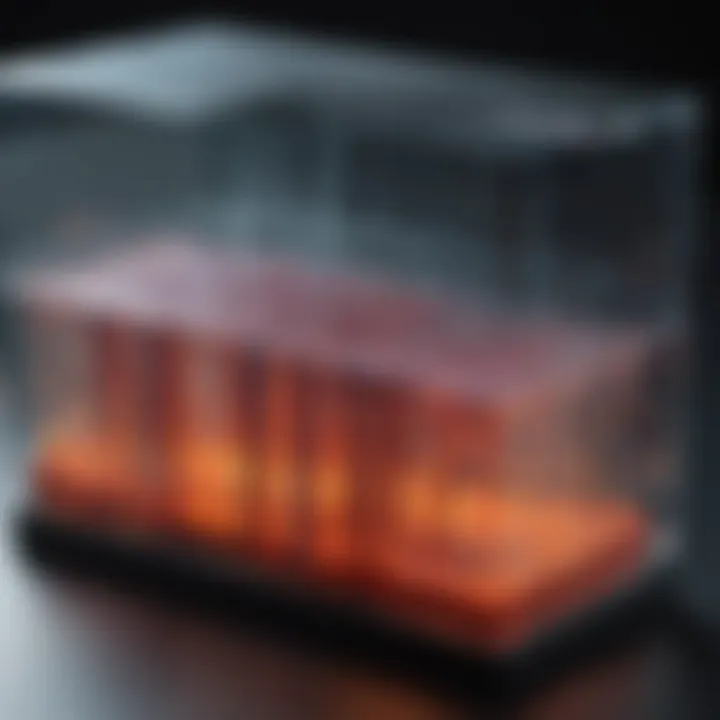
Intro
Electrophoresis is a critical technique in molecular biology, biochemistry, and analytical chemistry. As a method for separating charged particles, it plays a vital role in various applications ranging from DNA analysis to protein purification. Understanding the complexities of the electrophoresis process can significantly enhance research capabilities and diagnostic accuracy in scientific fields.
This article provides a comprehensive guide to the electrophoresis process, detailing the fundamental principles, types, and applications of this essential laboratory technique. We aim to elucidate how electrophoresis operates and its significance in modern scientific research.
Research Overview
Summary of Key Findings
Through careful examination, the key findings indicate that electrophoresis is influenced by several factors that determine the mobility of particles during separation. These factors include the buffer composition, voltage applied, gel matrix, and temperature. Different types of electrophoresis, such as agarose gel electrophoresis and capillary electrophoresis, cater to specific applications and sample types.
Importance of the Research in Its Respective Field
Understanding electrophoresis is crucial for researchers and practitioners. It helps in key tasks such as genetic analysis, disease diagnosis, and quality control in pharmaceuticals. The ability to separate and analyze biological molecules with precision has unprecedented implications for fields like genetics and proteomics.
"Electrophoresis not only aids in research but is also pivotal for clinical applications, enhancing our understanding of complex biological systems."
Methodology
Description of the Experimental or Analytical Methods Used
Electrophoresis typically involves applying an electric field to a gel or liquid medium containing charged particles. The direction and speed of their movement depend on their charge and size. Different techniques, such as SDS-PAGE for proteins or PCR for DNA, are used depending on the desired outcome.
Sampling Criteria and Data Collection Techniques
Choosing the right samples is essential for successful electrophoresis. Factors influencing sample selection include the nature of the molecules, purity, and concentration. Data collection involves analyzing the bands or tracks formed after electrophoresis, often using imaging systems or densitometry for quantification.
Electrophoresis remains a cornerstone technique in molecular biology and beyond. Understanding its intricacies not only assists academic pursuits but also addresses practical challenges in various scientific disciplines.
Prelims to Electrophoresis
Electrophoresis is a crucial technique in many scientific fields, especially in molecular biology and biochemistry. This method enables the separation of charged particles, such as nucleic acids and proteins, based on their size and charge. Understanding electrophoresis is fundamental for researchers and students as it serves various practical applications in laboratories.
Definition and Historical Context
The term "electrophoresis" comes from the Greek words "electro" for electric and "phoresis" for carrying. It describes the movement of charged particles in a fluid under the influence of an electric field. This technique was first introduced by Arne Tiselius in the early 20th century, who utilized it to analyze proteins. Since then, it has evolved significantly, becoming central to techniques used in many biological and chemical contexts.
The historical development of electrophoresis parallels advancements in understanding molecular structure. Early methods employed simple paper chromatography, which laid down the foundation for more sophisticated techniques like agarose gel electrophoresis and polyacrylamide gel electrophoresis. These innovations have opened doors for many breakthroughs in genetic research and diagnostics.
Importance in Scientific Research
The role of electrophoresis in scientific research cannot be overstated. It is particularly valuable in the fields of molecular biology and genetics, as it allows for the analysis of DNA, RNA, and proteins. The ability to distinguish molecular sizes and charge provides insights into various biochemical processes and helps in identifying genetic disorders.
Electrophoresis also plays a vital role in clinical diagnostics. It enables the profiling of proteins in blood serum, contributing to the understanding of diseases like multiple myeloma and other health conditions. Moreover, its applications extend into environmental sciences, where it aids in the detection of pollutants in water samples.
In summary, electrophoresis is not just a laboratory technique; it is a pivotal tool that drives research and innovation across various scientific disciplines. Its historical roots underline its importance, while current applications highlight its relevance in modern scientific inquiry.
Basic Principles of Electrophoresis
Understanding the basic principles of electrophoresis is crucial for anyone working in molecular biology and related fields. This section explains the foundational concepts, addresses the types of charge and migration, and examines the factors affecting electrophoretic mobility. The knowledge gained here will help in improving experimental designs and achieving better results in various applications.
Fundamental Concepts
Electrophoresis involves the movement of charged particles in a fluid under the influence of an electric field. This process separates molecules based on their size, shape, and charge. The fundamental concept revolves around the interaction between the electric field and the ions or molecules within the medium. Generally, smaller and more highly charged molecules migrate faster than larger ones. Understanding this fundamental principle is essential for scientists, as the choice of conditions directly affects separation quality and resolution.
Types of Charge and Migration
Charges on molecules influence their movement in an electric field. There are two primary types of charge: positive and negative. Cations move toward the negative electrode, while anions migrate toward the positive electrode. This migration occurs as the electric field exerts force on the charged particles, leading to their movement through the medium. The type of medium used, whether agarose, polyacrylamide, or other, directly impacts the rate and quality of separation by providing different levels of resistance to particle movement.
Factors Affecting Electrophoretic Mobility
Understanding factors affecting electrophoretic mobility is key for optimizing results. Several elements contribute:
Electric Field Strength
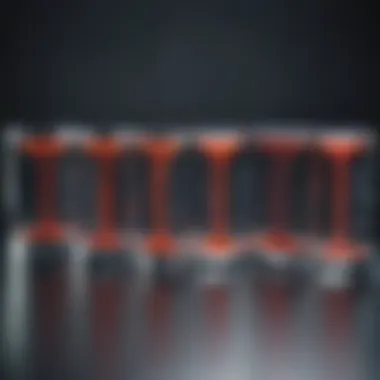
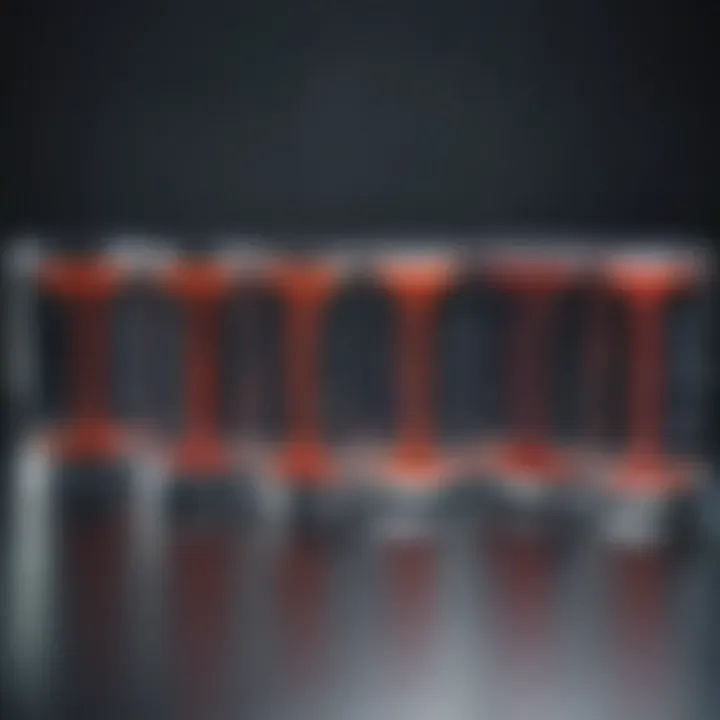
The electric field strength is a vital aspect. It determines how quickly charged particles move. Higher field strengths increase mobility but can also result in overheating and decreased resolution. The choice of electric field strength must balance speed and separation quality. It serves as a fundamental control in experimental designs, impacting data outcome significantly.
Viscosity of the Medium
Viscosity affects how easy or difficult it is for particles to migrate through the medium. A higher viscosity slows down movement, while a lower viscosity allows faster migration. The choice of buffer and gel concentration plays a critical role in achieving the desired viscosity. Thus, selecting the right medium viscosity is important for operational efficiency and data clarity.
Size and Shape of Molecules
The size and shape of molecules contribute significantly to their mobility. Larger molecules experience more resistance than smaller molecules. Similarly, elongated shapes may present different resistance than spherical ones. This variation in mobility allows the separation of components during electrophoresis. It is essential to understand these traits when designing experiments for specific applications.
Charge Density
Charge density is crucial in determining how charged molecules behave in an electric field. Higher charge density can lead to more rapid migration, while lower charge density results in slower mobility. Evaluating charge density helps in predicting movement patterns, thus facilitating better separation techniques. Balancing charge density with other factors is essential to obtain reliable results in electrophoresis experiments.
"The efficiency of electrophoresis largely depends on the understanding of fundamental principles and the ability to manipulate various factors."
In summary, mastering the basic principles of electrophoresis allows researchers and technicians to make informed decisions in their work, optimizing results across a wide range of applications.
Types of Electrophoresis Techniques
Understanding the different types of electrophoresis techniques is essential for anyone involved in molecular biology, biochemistry, or analytical chemistry. Each method has specific applications, advantages, and limitations. Thus, the choice of technique can significantly influence the results of experiments. Here, we explore key techniques, including agarose gel, polyacrylamide gel, capillary electrophoresis, and isoelectric focusing.
Agarose Gel Electrophoresis
Applications in DNA Analysis
Agarose gel electrophoresis is widely recognized for its role in DNA analysis. This technique is particularly effective for separating nucleic acids based on size. One of the key characteristics of agarose gel electrophoresis lies in its ability to create a porous medium where DNA fragments can migrate through. This property makes it a preferred choice for applications such as restriction fragment length polymorphism (RFLP) and dideoxy sequencing. The procedure is beneficial because it is relatively simple and cost-effective compared to other methods. Nevertheless, the challenge may arise when separating very small DNA fragments as their resolution might not be adequate.
Procedure Overview
The procedure for agarose gel electrophoresis involves the preparation of an agarose gel, loading of the sample, and the application of an electric field. This method is popular because it allows for straightforward visualization of DNA, typically by staining with ethidium bromide or a safer alternative. Visualization can be done using UV light, enhancing the ease of interpretation. However, the gel preparation time might be seen as a limitation, especially in high-throughput scenarios.
Polyacrylamide Gel Electrophoresis
Protein Separation Techniques
Polyacrylamide gel electrophoresis (PAGE) is another sophisticated method widely used for protein separation. The standout feature of PAGE is its tunable pore size. This characteristic allows for better resolution of proteins of various sizes. Researchers often use it for analyzing protein purity and estimating molecular weight. It is especially valuable in the context of complex sample types like cell lysates. One downside could be the requirement for toxins and chemicals in gel preparation.
Advantages and Limitations
When it comes to advantages and limitations of PAGE, high resolution is a key benefit. It provides clearer separation of similar-sized proteins, making it a reliable choice for detailed analysis. However, the technique requires specific knowledge and skills for optimization, which could be a barrier for novice users. Additionally, the gels must be well-cast, as imperfections can interfere with the results, making it critical to maintain technical precision.
Capillary Electrophoresis
Mechanisms of Operation
Capillary electrophoresis is especially intriguing due to its unique mechanism of operation. In this technique, samples are separated within narrow capillaries under a high electric field. The speed of separation can be significantly higher compared to traditional gel methods. The small sample volume is another appealing characteristic, making it cost-effective. However, the initial setup may require more investment.
Applications in Pharmacology
Capillary electrophoresis has opened new doors in pharmacology. Its rapid analysis capabilities allow for the study of drug interactions and metabolic pathways with greater precision. The ability to analyze multiple compounds simultaneously is a notable advantage. Nonetheless, user-friendliness remains a consideration; complex methods of data interpretation may pose challenges to some researchers.
Isoelectric Focusing
Principles and Setup
Isoelectric focusing is a highly specialized technique used for the separation of proteins based on their isoelectric points. The setup typically involves a gradient of pH within a gel. When an electric field is applied, proteins migrate until they reach a point where their net charge is zero. This method stands out due to its ability to resolve proteins with very similar molecular weights. However, it requires careful preparation of pH gradients which can be technically demanding.
Use in Protein Characterization
Isoelectric focusing plays a significant role in protein characterization. It helps in identifying and analyzing proteins based on their pI values. This analysis is crucial in various research applications, particularly in proteomics. The vertical profile of protein distribution can offer insights that are not readily accessible through other methods. The main limitation includes a longer time for setup and analysis compared to simpler gels, which may discourage some practitioners.
Applications of Electrophoresis
The applications of electrophoresis are pivotal across various scientific disciplines. It is particularly instrumental in molecular biology, clinical diagnostics, and environmental monitoring. Each application serves a unique purpose, providing valuable insights into biological systems, disease states, and environmental quality.
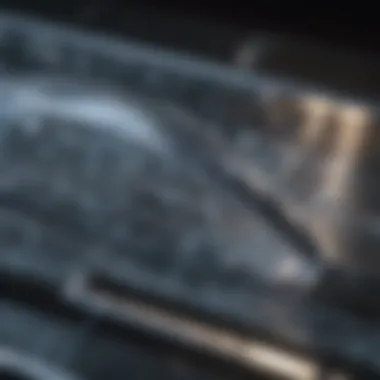
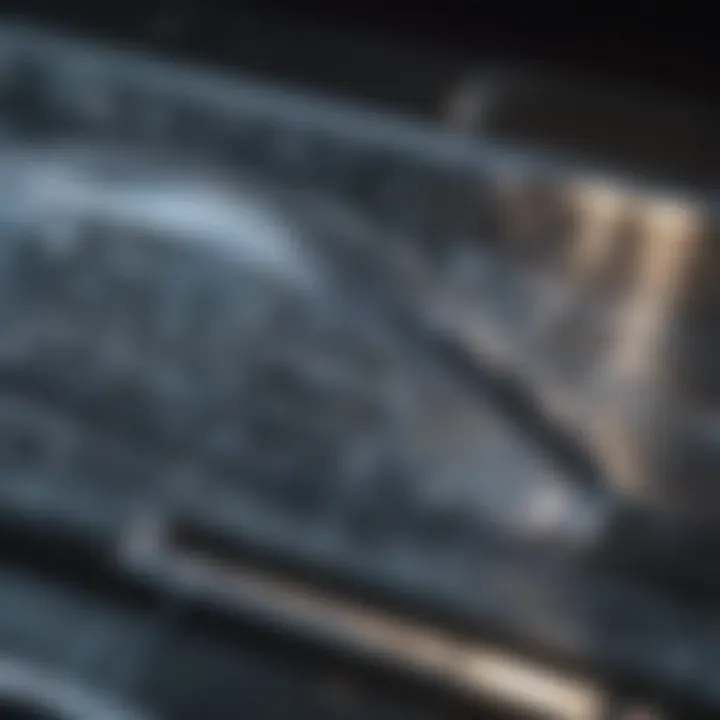
Molecular Biology
DNA Fragment Analysis
DNA fragment analysis plays a crucial role in molecular biology. This technique involves separating DNA fragments based on their size. It allows researchers to visualize genetic material effectively. The high-resolution separation of DNA fragments makes this method a preferred choice for many labs.
Key characteristic: It can detect even small differences in DNA sequence.
Unique feature: The ability to analyze a wide range of fragment sizes contributes to its popularity. Its applications include genotyping, cloning verification, and analyzing PCR products. One disadvantage might be the potential for artifacts during separation that can mislead analysis.
Genotyping Techniques
Genotyping techniques are essential for studying genetic variation. They help identify differences within a population's DNA. This information is important for understanding genetic diseases and traits.
Key characteristic: These techniques can resolve variations at single nucleotide levels.
Unique feature: The speed and accuracy of genotyping methods enhance research productivity. Genotyping contributes to personalized medicine approaches, understanding ancestry, and population genetics. However, challenges include the requirement for rigorous controls to ensure data reliability.
Clinical Diagnostics
Protein Profiling in Disease States
Protein profiling is significant for identifying biomarkers in diseases. This application uses electrophoresis to separate proteins from various biological samples. It can indicate the presence of certain diseases, enabling early diagnostics.
Key characteristic: It provides a comprehensive overview of protein expression.
Unique feature: The quantitative aspect of protein profiling helps in monitoring disease progression. The integration with other analytical methods optimizes diagnostic processes. However, it may require expensive equipment and can be time-consuming.
Screening for Genetic Disorders
Screening for genetic disorders is another critical application. Electrophoresis can identify specific genetic markers associated with disorders. This function ensures timely interventions and better patient management.
Key characteristic: It enables the detection of inherited conditions before symptoms appear.
Unique feature: Early detection can significantly alter treatment outcomes. This ability makes it a vital tool in pediatric medicine. Challenges include the ethical implications of genetic testing and the potential for false positives.
Environmental Monitoring
Detection of Pollutants
Detection of pollutants through electrophoresis helps in assessing environmental health. This application can determine the presence of hazardous substances in samples such as water, soil, and air.
Key characteristic: Sensitivity to low concentrations allows for early detection of environmental hazards.
Unique feature: The versatility in analyzing different types of pollutants aids researchers in understanding ecosystem health. However, it may necessitate extensive sampling and specificity for various pollutants.
Study of Microbial Communities
Studying microbial communities via electrophoresis has implications in ecology and health. Electrophoretic techniques can separate microbial DNA, allowing researchers to study diversity and abundance.
Key characteristic: Provides clear identification of microbial species in various environments.
Unique feature: This method contributes to understanding microbial interactions within ecosystems. It also aids in identifying potentially harmful species. Limitations include the complexity of interpreting results due to microbial community dynamics.
Optimizing Electrophoresis Conditions
Optimizing electrophoresis conditions is crucial for ensuring reproducibility and accuracy in experimental results. This section will delve into various aspects that scientists must consider to achieve optimal performance in electrophoresis, including buffer selection, gel concentration, and temperature control. Each of these factors directly impacts the resolution and efficiency of molecule separation, which are essential for the quality of data obtained from electrophoretic techniques. Proper optimization not only enhances data integrity but also reduces potential errors during analysis, making this an indispensable part of electrophoresis protocols.
Choosing Appropriate Buffers
Buffers play a vital role in the electrophoresis process. They provide the necessary environment for the molecules being analyzed to migrate effectively under an electric field. The choice of buffer can influence multiple aspects, including pH, ionic strength, and compatibility with the samples.
Some common buffer systems include Tris-Glycine for proteins and TBE for nucleic acids. Adjusting pH levels is essential, as it affects the charge and solubility of the molecules. Higher ionic strength buffers can lead to increased conductivity, thus aiding in ion migration but also may create heat, which can distort gel integrity. It is crucial to balance these properties to ensure the best performance.
Key considerations for buffer selection include:
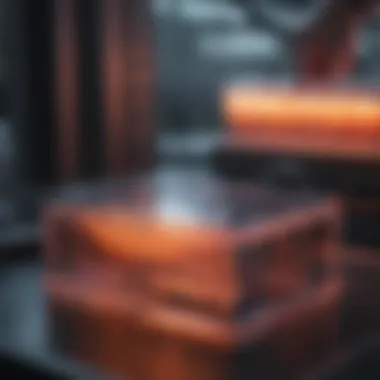
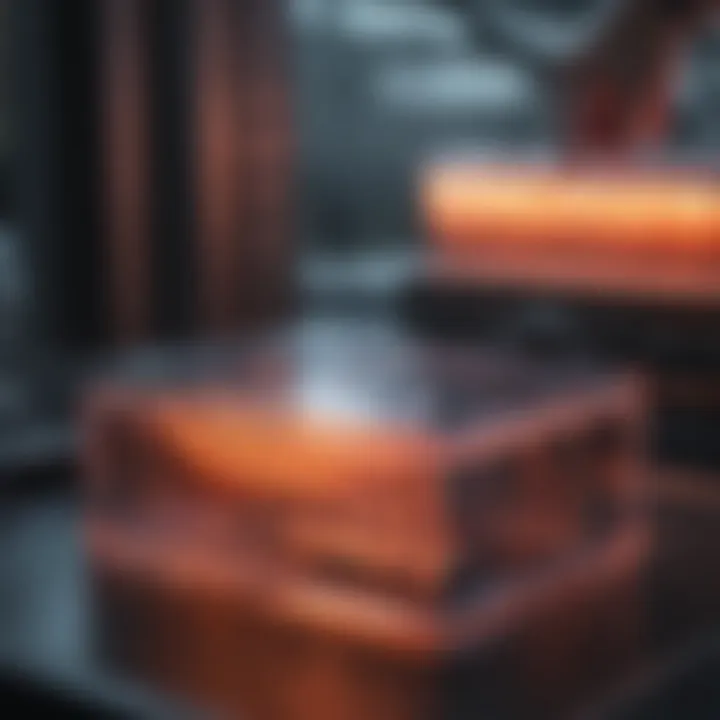
- Type of molecules: Different molecules require different buffers.
- Ionic strength: Higher ionic strength could lead to heat-related issues.
- pH stability: Ensure buffer can maintain stable pH during electrophoresis.
In summary, appropriate buffer selection directly affects the resolution and quality of results in electrophoresis.
Gel Concentration and Thickness
The concentration and thickness of the gel are equally important in optimizing electrophoresis. Gel concentration determines the pore size and, thus, the resolving power of the gel. A higher concentration gel has smaller pores, while a lower concentration gel provides broader spaces for larger molecules to migrate through.
Once the right concentration is determined, gel thickness comes into play. A well-prepared gel not only influences the some clarity of lanes but also affects heat distribution during electrophoresis. Uneven gel thickness can lead to inconsistent results.
Identifying the optimal concentration often requires experimental calibration, but some general rules include:
- Agarose gel: Typically, 0.5% to 2% concentration is used for DNA, depending on the size of the fragments.
- Polyacrylamide gel: Generally ranges from 5% to 15% for proteins, tailored to the particular protein size of interest.
Overall, proper gel conditions promote high efficiency and clear separation, which is vital for accurate analysis.
Temperature Control during Electrophoresis
Temperature control during electrophoresis is often overlooked, yet it has a significant impact on the quality and reproducibility of results. During electrophoretic runs, especially at high voltages, heat generation can distort molecular behavior and gel stability. Excessive heat can cause band broadening and poor resolution, leading to inaccurate data interpretation.
A few techniques to manage temperature include:
- Low-voltage runs: Slower migration at lower voltages produces less heat.
- Cooling systems: Using coolers or water baths can help maintain a consistent temperature, especially in larger setups.
- Monitoring equipment: Implementing thermocouples or temperature sensors can provide real-time data, allowing for adjustments during critical runs.
By paying attention to temperature variations, researchers can ensure that electrophoresis results are reliable and reproducible.
Interpreting Electrophoresis Results
Interpreting the results from electrophoresis experiments is a crucial phase in the analysis. This section elaborates on the significance of generating clear insights from the banding patterns observed in electrophoresis gels and their implications for scientific conclusions. Successful interpretation impacts the validity of the results, allowing scientists to draw accurate deductions about molecular behavior and composition. This analysis is key for confirming hypotheses and facilitates ongoing research.
Analyzing Band Patterns
The analysis of band patterns forms the backbone of data evaluation in electrophoresis. When samples undergo electrophoresis, they separate based on size, charge, or other properties, resulting in distinct bands on the gel. Understanding how to interpret these bands accurately contributes enormously to confirming purposes within biological assays.
Quantifying Band Intensity
Quantifying band intensity is essential for determining the relative concentrations of analytes in a sample. By measuring how dark or sturdy a band appears, researchers can estimate the amount of substance present. This process often employs imaging software or densitometry for precise measurements. The key characteristic of quantifying intensity is its quantitative nature, making it a beneficial choice for studies requiring numerical data on sample amounts.
The unique feature of this method is its objectivity, which minimizes human error in visual assessments. However, researchers should be cautious as band intensity can also be influenced by factors such as gel composition and running conditions. Thus, it's essential to establish standardized procedures to ensure consistency across experiments.
Identifying Contaminants
Identifying contaminants is another facet of analyzing band patterns. This aspect focuses on detecting any undesired molecules present in the sample. It plays a vital role in ensuring the purity and reliability of the data obtained from electrophoresis. The key characteristic of identifying contaminants is its ability to enhance the credibility of results.
The unique feature of this part is that it can reveal unexpected results or artifacts, which may lead to incorrect interpretations if not addressed. The challenge, however, lies in distinguishing between legitimate signals and background noise. Careful optimization of the electrophoresis conditions can mitigate these issues, leading to clearer and more informative readouts.
Software Tools for Data Analysis
In the modern laboratory, several software tools facilitate data analysis from electrophoresis runs. These programs allow for sophisticated quantification and cleaner imaging of gel patterns. Software such as Bio-Rad's Image Lab or GelAnalyzer has gained popularity among researchers for their features in analyzing complex gel images and providing high-resolution data interpretations.
Being adept at utilizing these tools can significantly speed up the analysis process. However, they require user familiarity and practice to be used effectively. Additionally, choosing software should align with specific research objectives, ensuring that it meets the requirements of the intended analysis.
Future Perspectives in Electrophoresis Technology
The field of electrophoresis technology continues to evolve, with ongoing innovations aimed at enhancing its efficiency and applicability across various scientific domains. Future perspectives in electrophoresis encompass advancements in materials, methodologies, and the integration of new technologies that aim to improve the resolution and speed of the separation processes. Understanding these developments is crucial for researchers and professionals who rely on electrophoresis techniques for molecular analysis.
Advancements in Material Science
Material science plays a pivotal role in the future of electrophoresis technology. The evolution of new gel matrices and buffer systems has significant potential to enhance the performance of electrophoretic methods. For example, novel polymers and nanomaterials may improve the resolution of separations, allowing for finer discrimination between similar biomolecules.
Additionally, advancements in surface modifications can lead to reduced nonspecific binding and heightened sensitivity in results. By optimizing material properties, researchers can achieve better control over the structural and functional aspects of electrophoretic environments. The introduction of biodegradable gels also addresses environmental concerns, promoting sustainability without sacrificing performance.
Integration with Other Techniques
The integration of electrophoresis with other analytical techniques is another area with promising future prospects. Combining electrophoresis with mass spectrometry, for instance, can provide enhanced data about the molecular weight and composition of analytes. This integrated approach can yield comprehensive insights that single methods cannot deliver alone.
Furthermore, the amalgamation with chromatography can create a powerful synergy, facilitating the analysis of complex mixtures and improving throughput. Techniques like microfluidics can also shift traditional electrophoresis paradigms. They enable miniaturization and increased automation, leading to faster analysis cycles and lower reagent usage. The potential for real-time data acquisition through these integrations can significantly enhance the relevance and functionality of electrophoresis in dynamic research settings.
"The future of electrophoresis is not only about improving separation techniques but also about integrating them seamlessly with other technologies for a holistic analytical approach."
Research and education in these interdisciplinary strategies are essential, as they prepare the next generation of scientists to leverage these advancements effectively. The continuing exploration of novel materials and the integration with complementary methodologies will undoubtedly shape the future of electrophoresis, making it a more versatile tool in scientific research.