Exploring Fluorescence Microscopes: Types and Applications
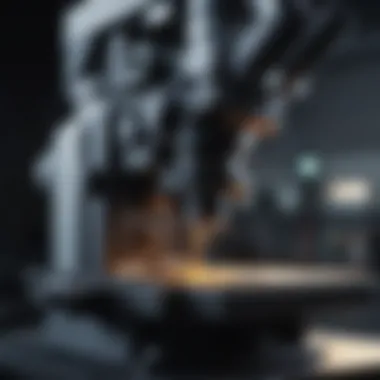
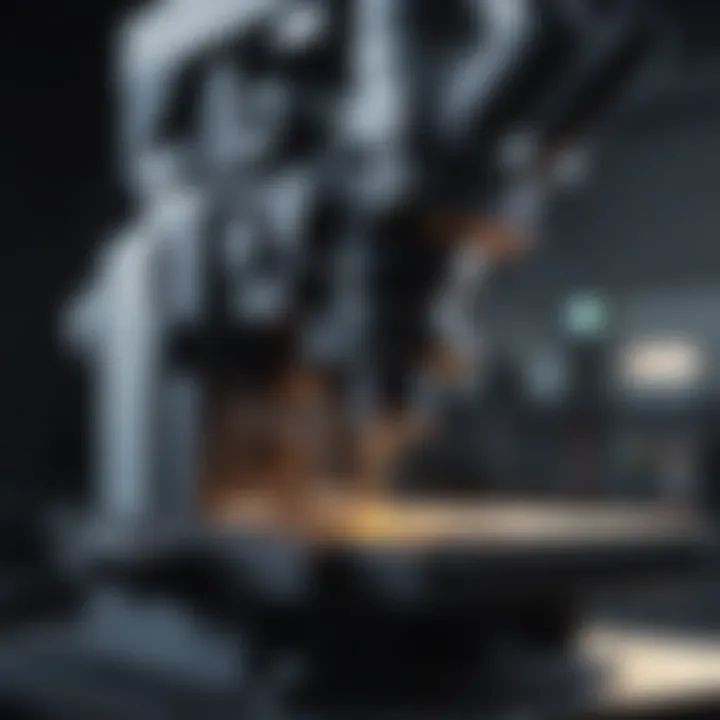
Intro
Fluorescence microscopy represents a pivotal advance in the field of imaging techniques, providing unparalleled insights into the intricate details of biological specimens. The technique harnesses the principle of fluorescence, where certain substances emit light upon excitation by specific wavelengths. This method is crucial in various scientific disciplines, particularly in biology and materials science.
As research expands and evolves, fluorescence microscopes have undergone significant innovations and improvements, enhancing their capabilities for detailed observation. Ranging from basic to advanced systems, these microscopes serve diverse applications, including cellular imaging and live-cell studies. Understanding these various types, along with their operational principles, advantages, and limitations, sets the foundation for profound appreciation of their role in contemporary research.
Research Overview
Summary of key findings
In studying fluorescence microscopes, several key findings emerge:
- Operational Principles: Fluorescence microscopes operate on the emission of light by fluorescent markers that bind to specific cellular components.
- Diverse Techniques: Techniques such as confocal microscopy, two-photon microscopy, and super-resolution microscopy offer distinct advantages for various applications.
- Applications: These microscopes are extensively used in the fields of cell biology, biochemistry, and medical diagnostics, contributing to both basic and applied research.
Importance of the research in its respective field
The importance of fluorescence microscopy cannot be overstated. This technique not only allows for detailed visualization of cellular structures but also aids in tracking dynamic processes in real-time. As new fluorescent dyes and imaging systems emerge, researchers continually refine their methodologies.
"Fluorescence microscopy has revolutionized our understanding of cellular mechanisms and has opened up new avenues in biomedical research."
This advancement enhances scientific inquiry, drives innovation, and supports the progress of knowledge in multiple disciplines by providing a window into the cellular world that was previously unattainable.
Methodology
Description of the experimental or analytical methods used
Fluorescence microscopy methodologies encompass various experimental designs:
- Sample Preparation: Samples are often treated with fluorescent tags, which may include antibodies conjugated with fluorophores for specific localization.
- Imaging Techniques: Different microscopy techniques are applied based on the requirement of resolution, speed, and depth of imaging.
Sampling criteria and data collection techniques
Data collection involves meticulous techniques:
- Image Capture: Digital cameras collect images, which are then analyzed using software tailored for fluorescence imaging.
- Quantitative Analysis: Techniques such as flow cytometry and quantitative image analysis provide statistical insights into samples, enhancing the interpretive power of collected data.
In summary, fluorescence microscopy stands as a cornerstone of modern imaging techniques, with ongoing research and techniques continuously shaping its landscape. Understanding the nuances and applications of various types of light microscopy facilitates deeper insights into biological processes and enhances our capacity for innovation in science.
Preamble to Fluorescence Microscopy
Fluorescence microscopy stands as a cornerstone in the field of biological imaging, providing a dynamic platform for scientists across various disciplines. This technology allows researchers to visualize biological samples at the molecular level, revealing details that would otherwise remain hidden. The advent of fluorescence microscopy has revolutionized techniques in cellular biology, allowing for an enhanced understanding of cellular processes and structures. This section delves into the definition of fluorescence microscopy and its historical significance, illuminating the journey of this powerful technique.
Definition and Importance
Fluorescence microscopy is a specialized form of microscopy that employs the phenomenon of fluorescence to visualize specimens. Fluorescence occurs when certain molecules, known as fluorophores, absorb light at a specific wavelength and subsequently emit light at a longer wavelength. This technique is critical for studying the localization and dynamics of molecules within cells. The importance of fluorescence microscopy lies in its ability to provide high-contrast images, enabling researchers to observe and quantify various biological processes in real time.
The use of fluorescence microscopy has widespread applications in fields such as molecular biology, biochemistry, and medical diagnostics. It permits the exploration of cellular structures, enables the tracking of molecular interactions, and aids in elucidating functional relationships within cells. The technique not only enhances the visualization of live specimens but also allows for multiple labeling, where different fluorophores can be used simultaneously, producing complex images that provide deeper insights into cellular behavior.
Historical Context
The history of fluorescence microscopy is rich and intricately tied to advancements in both optics and fluorescence technology. The origins of fluorescence microscopy can be traced back to the discovery of fluorescence itself in the mid-19th century. Sir George Gabriel Stokes, in 1852, was the first to describe the principles of fluorescence, laying the groundwork for future innovations.
In the early 20th century, researchers began to utilize fluorescence in microscopy, with the advent of ultraviolet light sources and advancements in photographic techniques. Notably, the development of specific stains and dyes revolutionized the ability to visualize cellular components. The introduction of fluorescence filter sets and advanced lenses further improved image quality and resolution, allowing for more detailed studies.
With the continuous growth of molecular biology in the late 20th century, fluorescence microscopy experienced exponential advancement. Innovations like confocal microscopy and super-resolution techniques fundamentally transformed research capabilities, enabling unprecedented imaging power.
Now, fluorescence microscopy stands as a vital tool in laboratories worldwide, facilitating groundbreaking discoveries in cellular biology and beyond.
Basic Principles of Fluorescence
Understanding the basic principles of fluorescence is crucial for anyone engaged in fluorescence microscopy. It forms the foundation upon which various microscopy techniques are built. Fluorescence microscopy has transformed biological imaging, making it essential in many scientific fields. Knowing how fluorescence works helps researchers choose appropriate methods for their studies and enables them to interpret results more effectively.
Fluorescence Phenomenon
The fluorescence phenomenon involves the absorption of light by a substance, followed by the re-emission of that light at a longer wavelength. This process occurs in two main stages.
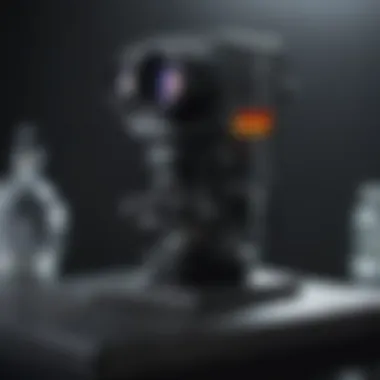
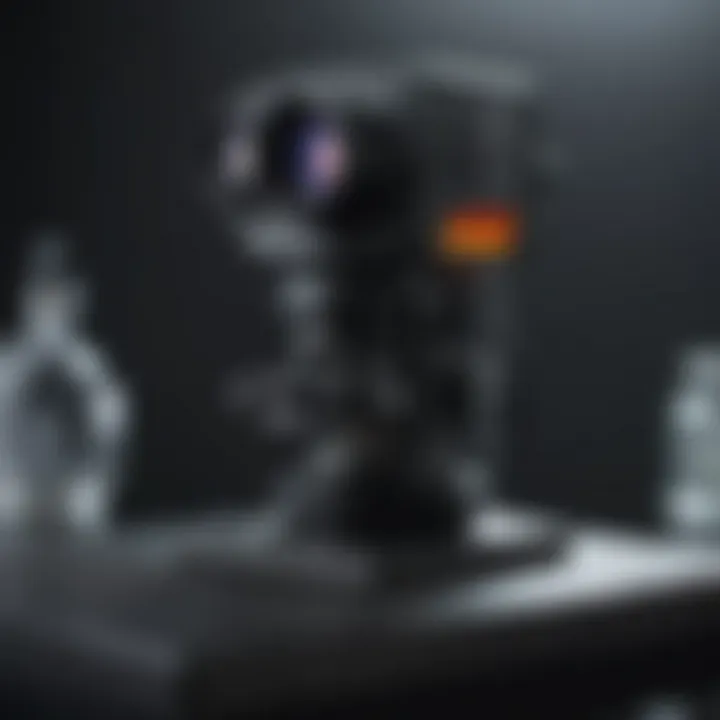
- Absorption: Molecules, known as fluorophores, absorb photons from a light source. This energy excites electrons in the fluorophores, elevating them to a higher energy state.
- Emission: Once the electrons return to their original state, the energy is released in the form of light, but at a longer wavelength than the absorbed light. This characteristic makes fluorescence useful for imaging structures within cells and tissues.
Understanding the behavior of fluorophores is vital as it affects signal strength and contrast in images. Different fluorophores have specific excitation and emission wavelengths, meaning they must be chosen based on the microscopy setup and the biological question being investigated.
"Fluorescence microscopy leverages the unique properties of materials that emit light when excited, offering powerful insights into cellular structures."
Excitation and Emission
Excitation and emission are critical concepts in fluorescence microscopy. Light sources, typically lasers or high-intensity lamps, produce light that excites fluorophores within the sample. The careful selection of wavelengths for excitation is key. It is crucial that the excitation wavelength aligns closely with the absorption spectrum of the fluorescent dye being used.
The emitted light is then collected and filtered to distinguish it from the excitation light. This results in a clear image of the fluorescent structures in the sample. Often, multiple fluorophores can be used simultaneously, each with unique excitation and emission properties.
In practical terms, when setting up a fluorescence microscopy experiment, researchers must consider:
- Wavelength matching: Ensuring the excitation light matches the fluorophore's absorption maxima.
- Filter selection: Using the right filters to separate excitation light from emitted light to enhance image quality.
This combination of excitation and emission processes drives the effectiveness of fluorescence microscopy, allowing visualization of cellular and molecular dynamics in real-time.
Understanding these principles equips researchers with the knowledge to design experiments that take full advantage of fluorescence microscopy's capabilities.
Types of Fluorescence Microscopes
Fluorescence microscopy has revolutionized the way researchers and scientists observe biological samples. Understanding the different types of fluorescence microscopes is crucial for selecting the right methodology in various applications. Each type has unique features, strengths, and weaknesses that cater to specific research needs.
Widefield Fluorescence Microscopes
Widefield fluorescence microscopes are perhaps the most commonly used types in laboratories. They illuminate the entire sample with a broad excitation light source, which allows for rapid image acquisition. This method is advantageous for observing large areas and multiple fluorescent markers simultaneously. However, one drawback is that it can suffer from background noise, as out-of-focus light is also collected along with the desired signal.
These microscopes are particularly useful in cellular biology and general imaging, where a quick overview is crucial. The ability to visualize different fluorescence signals in a single plane adds to its utility.
Confocal Microscopes
Confocal microscopes improve upon the widefield technique by using a focused laser beam for illumination. The key feature is a pinhole that allows only the light from a specific focal point to reach the detector. This results in images with enhanced contrast and decreased background fluorescence.
Confocal systems are ideal for obtaining high-resolution images of thicker specimens, as they enable z-stack imaging, where multiple thin slices of a sample are taken and reconstructed into three dimensions. This capability is indispensable in advanced biological research and allows for more detailed imaging of cellular structures.
Total Internal Reflection Fluorescence (TIRF) Microscopes
Total Internal Reflection Fluorescence applies a unique method of excitation that enhances the signal from molecules located in close proximity to a glass coverslip. This technique uses an evanescent wave that penetrates only a few hundred nanometers into the sample. The main advantage of TIRF is its ability to minimize background fluorescence while focusing on near-membrane phenomena, making it ideal for studying processes such as membrane dynamics and protein interactions at the interface of cellular membranes.
While TIRF is superb for specific applications, it is limited to thin regions, often requiring precise sample positioning and careful preparation to avoid unwanted artifacts.
Multiview 3D Microscopes
Multiview 3D microscopy represents a fascinating advancement in imaging technology. These systems capture images from multiple angles, allowing for the reconstruction of three-dimensional structures. This is particularly useful in complex biological samples, such as tissues or large cells, where a comprehensive view is essential for understanding spatial relationships.
They facilitate advanced imaging with reduced phototoxicity and improved data collection efficiency. However, the complexity of setup and data analysis may present challenges, meaning that users require prior experience with advanced imaging techniques.
In summary, the choice of fluorescence microscope significantly influences the quality of visual data and thus the outcomes of scientific investigations.
Understanding the differing principles and applications of each type enables researchers to select the most suitable technology for their specific needs in biological research.
Advanced Techniques in Fluorescence Microscopy
Advanced techniques in fluorescence microscopy have revolutionized the way researchers analyze biological samples. These methods provide enhanced resolution, specificity, and quantification capabilities. They are crucial for understanding complex cellular processes. Researchers can visualize interactions at the molecular level, which helps in drug development, cellular behavior studies, and more.
A few key advanced techniques are Fluorescence Recovery After Photobleaching (FRAP), Fluorescence Resonance Energy Transfer (FRET), and Super-Resolution Microscopy. Each of these methods offers unique advantages and applications that can significantly improve the analytical capabilities of scientists.
Fluorescence Recovery After Photobleaching (FRAP)
Fluorescence Recovery After Photobleaching (FRAP) is a powerful technique used to measure the dynamics of molecules within live cells. The principle behind FRAP involves the selective bleaching of fluorescently labeled molecules in a defined area within the specimen. After this, researchers monitor the reappearance of fluorescence in that area over time. This recovery occurs as unbleached, fluorescent molecules diffuse back into the bleached region.
This technique allows scientists to assess diffusion rates, binding kinetics, and interactions between different biomolecules. It is particularly useful in studying cell membrane dynamics, protein interactions, and even cellular signaling pathways. By quantifying recovery, insights into molecular mobility and concentration can be gained, informing about cellular processes.
Fluorescence Resonance Energy Transfer (FRET)
Fluorescence Resonance Energy Transfer (FRET) is another sophisticated method that measures distances between molecules at a nanometer scale. In essence, FRET occurs when two fluorophores are positioned close together, enabling energy transfer from a donor fluorophore to an acceptor. This transfer can be influenced by various factors such as distance and orientation between the two molecules.
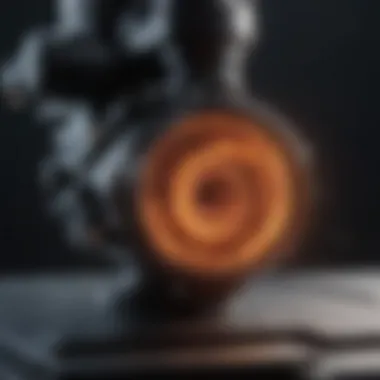
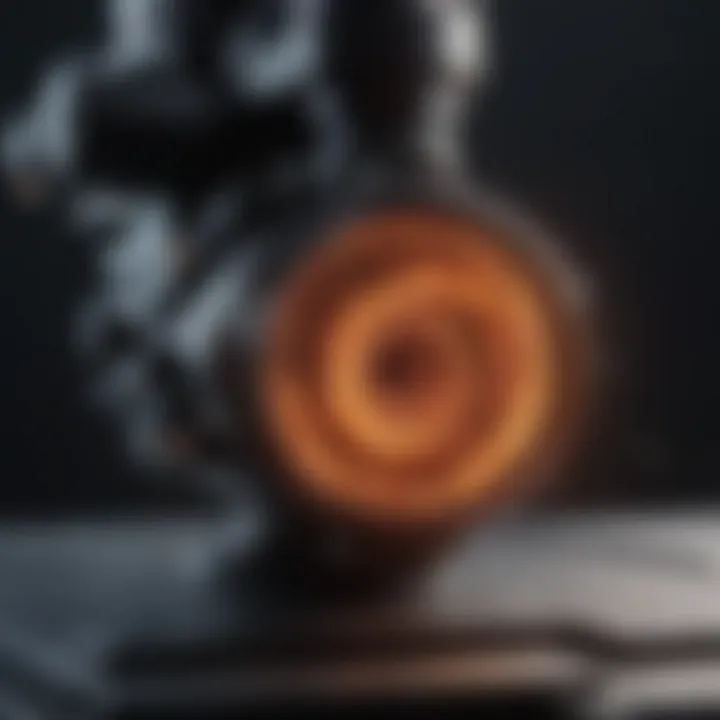
FRET can be used to study protein interactions, molecular conformations, and dynamics within cells. The efficiency of energy transfer provides quantitative data, allowing researchers to explore conformational changes or binding events in real-time. This information is vital in understanding biological mechanisms and pathways, as it provides insight into how molecules interact at such close ranges.
Super-Resolution Microscopy
Super-Resolution Microscopy represents a significant leap forward from conventional microscopy techniques. This approach allows for imaging beyond the diffraction limit, enabling visualization of structures at a resolution of tens of nanometers. Techniques such as Stimulated Emission Depletion (STED) and Photoactivated Localization Microscopy (PALM) are key examples of super-resolution methods.
The implications of super-resolution microscopy are vast. It can visualize protein complexes, cytoskeletal structures, and intricate cellular architectures. This high-resolution imaging can reveal ultra-fine details that are essential for understanding complicated biological processes. For example, it can help decipher molecular assemblies, which are crucial in various cellular functions and signaling pathways.
Applications of Fluorescence Microscopy
Fluorescence microscopy serves as a vital tool in contemporary scientific research. This technique enables precise visualization of cellular components and dynamic processes within living cells. The importance of this section lies in understanding how fluorescence microscopy is applied across distinct fields, transforming the way we study biological systems. Recognizing the practical applications can help researchers harness the full potential of this technology.
Cell and Molecular Biology
In cell and molecular biology, fluorescence microscopy is indispensable. It allows scientists to observe intricate details of cellular structures like nuclei, mitochondria, and cytoskeletons. Common applications involve the use of specific fluorescent dyes that can bind to particular proteins or nucleic acids within cells.
- Protein Localization: Researchers tag proteins with fluorophores to visualize their distribution. For example, the use of GFP (Green Fluorescent Protein) helps in tracking the movement of a protein in live cells.
- Cell Cycle Analysis: Fluorescence techniques facilitate investigating the cell cycle phases. Dyes like propidium iodide can stain DNA, allowing the identification of cells in various stages of division.
- Labeling of Cellular Organelles: Using specific fluorophores, organelles can be labeled. This gives insights into organelle function and interactions within the cell.
Through these applications, fluorescence microscopy advances our understanding of the fundamental processes that underlie cellular function and organization.
Neuroscience Research
The application of fluorescence microscopy extends profoundly into neuroscience. This field utilizes the capability of fluorescence to study neural structures and functions. By employing various imaging techniques, researchers explore complex brain activity and connectivity.
- Imaging Synapses: The localization of fluorescent markers in synaptic regions allows the observation of synaptic activity. Changes in fluorescence can indicate neurotransmitter release and receptor activation.
- Mapping Neural Pathways: Fluorescent tracing methods help delineate neural circuits. By using transgenic techniques, specific populations of neurons can be labeled, revealing their connections within the brain.
- Calcium Imaging: Calcium indicators are employed to monitor electrical activity. Changes in intracellular calcium levels can be visualized, providing insight into neural excitability and signaling.
These applications contribute to a deeper comprehension of neurological processes and the underlying mechanisms of various neural diseases.
Clinical Diagnostics
Fluorescence microscopy is increasingly important in clinical diagnostics. Its ability to provide detailed images of pathological samples plays a crucial role in disease identification and management.
- Cancer Diagnostics: Fluorescence in situ hybridization (FISH) allows the detection of specific genetic alterations in tumors. This helps in diagnosing various cancer types and determining treatment options.
- Infectious Disease Detection: Fluorescent antibodies can identify pathogens in clinical specimens. For instance, fluorescent staining can distinguish between bacterial and viral infections based on specific markers.
- Histopathology: Fluorescence microscopy is applied in examining biopsy samples. The visualization of specific markers can provide valuable information regarding disease progression and prognosis.
In summary, fluorescence microscopy enhances diagnostic capabilities, aiding clinicians in making informed decisions and improving patient outcomes.
"The precise imaging capability of fluorescence microscopy enables researchers to explore biological systems at unprecedented levels of detail."
Through the applications of fluorescence microscopy detailed above, it is clear that this technology plays an integral role in various scientific and clinical domains. Each unique application highlights a different aspect of biology or medical science, showcasing the versatility and essential nature of fluorescence microscopy.
Advantages and Limitations
Understanding the advantages and limitations of fluorescence microscopy is essential for researchers and practitioners in the field. These factors influence the choice of microscopy techniques for specific applications in biological imaging. Knowledge about the strengths ensures optimal use in research, while being aware of the limitations helps mitigate potential issues.
Advantages of Fluorescence Microscopy
Fluorescence microscopy stands out for several reasons that enhance its application in various scientific disciplines. Some of its key advantages include:
- High Sensitivity: Fluorescence microscopy offers remarkable sensitivity, allowing the detection of low-abundance molecular species. This sensitivity is particularly imperative in cellular studies, where the molecules of interest often exist in limited quantities.
- Specificity in Detection: The use of specific fluorescent probes enables selective imaging of target structures within complex biological systems. This selectivity is particularly valuable in cellular biology, where distinct cellular components need to be scrutinized.
- Functional Imaging: Beyond structural visualization, fluorescence microscopy can also provide insights into the functionality of biological systems. Techniques like FRET allow for the monitoring of interactions between proteins or other biomolecules in live cells.
- Real-Time Observation: The ability to observe live cells in real-time is a significant advantage. Researchers can study dynamic processes, such as cancer cell migration or neuronal activity, providing a deeper understanding of biological phenomena.
- Multicolor Imaging: The development of various fluorophores permits imaging multiple targets simultaneously, enhancing the ability to study cellular interactions and processes at a higher complexity.
These advantages contribute significantly to advancements in research, particularly in fields such as cell biology, neuroscience, and molecular diagnostics.
Limitations and Challenges
Despite its numerous advantages, fluorescence microscopy is not without limitations and challenges. It is crucial to recognize these aspects to make informed choices regarding its application:
- Photobleaching: Prolonged exposure to excitation light can lead to the irreversible loss of fluorescence from dyes. This photobleaching limits the duration of imaging and may affect data quality.
- Background Fluorescence: Non-specific binding of fluorophores can create background fluorescence that obscures signal detection. This can hinder the discernibility of the targets of interest.
- Depth Penetration: The ability to visualize structures diminishes with increased tissue depth. This challenge restricts the use of fluorescence microscopy in thick or dense specimens, particularly in vivo.
- Cost of Equipment: High-quality fluorescence microscopes can be expensive, both in terms of initial purchase and ongoing maintenance. Budget constraints may limit access for some researchers.
- Sample Preparation: The preparation of samples for fluorescence microscopy can be time-consuming and often involves complex staining protocols that can introduce variability between experiments.
"While fluorescence microscopy offers powerful advantages for biological imaging, being aware of its limitations is crucial for successful application in research."
Overall, balancing the advantages against the limitations is key to utilizing fluorescence microscopy effectively in research projects.
Recent Innovations in Fluorescence Microscopy
Recent innovations in fluorescence microscopy have significantly changed the landscape of biological imaging. They enhance our understanding of cellular processes and improve diagnostic capabilities in clinical settings. Key advancements include the development of novel fluorophores and the integration of artificial intelligence. These innovations are crucial for researchers and educators who rely on precise and high-quality imaging in various scientific studies.
Development of Novel Fluorophores
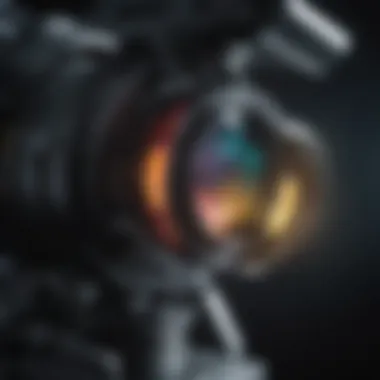
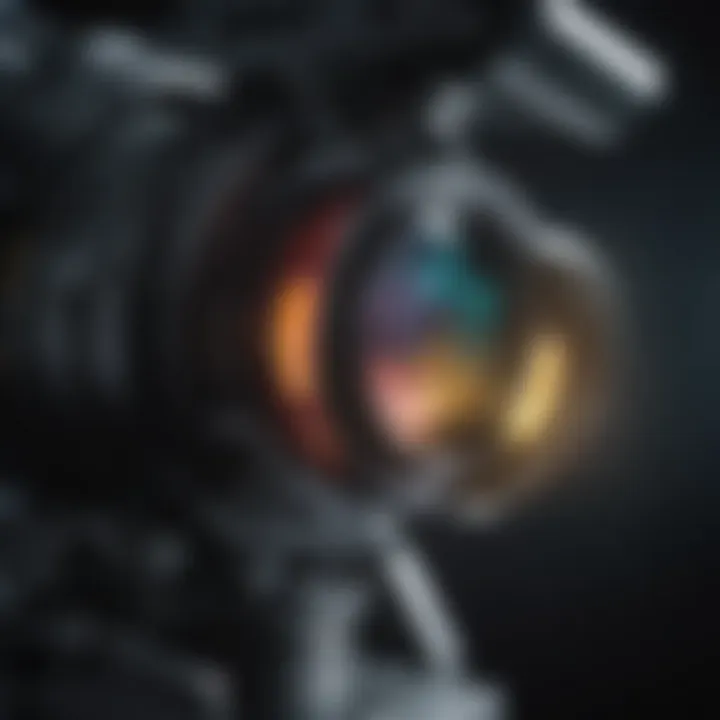
The introduction of novel fluorophores has transformed fluorescence microscopy. Fluorophores are molecules that absorb light at a certain wavelength and emit it at another. Innovations have led to more stable and brighter options, which ensure better visibility and longer observation times. Examples of new fluorophores include organic dyes with distinct spectral properties and quantum dots, which provide exceptional brightness and photostability.
Using advanced fluorophores contributes to improving signal-to-noise ratios in images, allowing for the detection of weak signals that might typically be overlooked. This is particularly important in studying low-abundance biomolecules. Additionally, specificity has increased with enhanced labeling techniques. Researchers can now target specific proteins or cells with remarkable precision, allowing investigations into their roles in health and disease.
Overall, the development of novel fluorophores not only provides versatility but also expands the potential applications in cell and molecular biology.
Integration with Artificial Intelligence
Artificial intelligence (AI) is becoming an essential tool in fluorescence microscopy. It operates as a support system, aiding in image acquisition, processing, and analysis. Machine learning algorithms can analyze large datasets quickly, revealing patterns that a human analyst might miss.
One key benefit of AI integration is automation. Automated image acquisition reduces human error, increasing reproducibility in experiments. Moreover, machine learning methods have improved image reconstruction methods, particularly in super-resolution imaging. These algorithms can enhance the quality of images by reconstructing details lost due to various physical limitations.
In practical terms, AI methods can efficiently classify complex biological structures, allowing researchers to focus on interpreting results rather than spending extensive time on data analysis. As a result, the impact on research is substantial: faster results, improved accuracy, and the ability to manage larger datasets than ever before.
"AI's role in microscopy is accelerating discoveries, facilitating deeper insights into biological processes."
In summary, the integration of artificial intelligence alongside the development of novel fluorophores represents the forefront of innovation in fluorescence microscopy. These advancements empower researchers to extract detailed information from biological samples more effectively and efficiently, making significant contributions to various fields of scientific inquiry.
Best Practices for Fluorescence Microscopy
Understanding best practices for fluorescence microscopy is vital for achieving accurate and reproducible results. This section delves into effective methods for optimizing both sample preparation and image acquisition, which are essential for capturing high-quality fluorescence images. By adhering to these guidelines, researchers can enhance their imaging protocols, leading to more reliable data in various biological contexts.
Sample Preparation Techniques
Effective sample preparation is crucial in fluorescence microscopy, as it directly influences the quality of the images obtained. The following techniques can be adopted to ensure optimal conditions:
- Fixation: Proper fixation methods, such as using formaldehyde or paraformaldehyde, help preserve cellular structures while maintaining fluorescence signals. It's important to choose fixation protocols that minimize the loss of fluorescence intensity.
- Staining: Selecting appropriate fluorophores is key. Each fluorophore has specific excitation and emission wavelengths. Researchers should avoid overlapping emissions from different fluorophores to maintain clarity in multicolor experiments. Choosing a balanced mix of fluorophores helps distinguish among tagged proteins.
- Mounting Medium: The choice of mounting medium can impact stability and fluorescence emission. Using antifade reagents prevents photobleaching during imaging. Ensure that the refractive index of the medium is compatible with your objective lenses to avoid distortions.
Making these preparations with care is essential for increasing the quality of your experiments.
Image Acquisition and Analysis
Image acquisition and subsequent analysis form the backbone of fluorescence microscopy. Both need attention to detail, ensuring that the images produced are of the highest quality. Here are some best practices:
- Acquisition Parameters: Set optimal exposure times and gain settings. It's ideal to avoid saturation of the camera sensor, which can lead to loss of information. Using the lowest exposure time that still provides a clear image is commonly recommended.
- Z-Stacking: In cases where samples have depth, using Z-stack imaging allows the capturing of multiple focal planes. This helps create a three-dimensional representation of the sample, which is beneficial for complex structures.
- Post-Processing: Software tools like ImageJ can be effective for analyzing fluorescence images. Techniques such as background subtraction and filtering can enhance image clarity. Proper element selection during analysis is crucial to prevent misinterpretation of the results.
"High-quality images are paramount for understanding biological systems, as they serve as visual evidence of underlying processes."
Adopting these best practices will help researchers maximize the potential of fluorescence microscopy, leading to more reliable and informative outcomes in their work.
Future Directions in Fluorescence Microscopy
The field of fluorescence microscopy is continuously evolving. As technology advances, so do the capabilities and applications of these powerful imaging tools. This section examines upcoming trends and potential impacts on research. Understanding the future directions in fluorescence microscopy is essential. It highlights its significance in enhancing biological imaging and improving scientific research across various disciplines.
Emerging Trends
Several trends are shaping the future of fluorescence microscopy. Among them, the following are particularly noteworthy:
- Development of Novel Fluorophores: New fluorophores are emerging with advanced properties. These include enhanced brightness, photostability, and specificity.
- Integration with AI and Machine Learning: Researchers are increasingly using artificial intelligence to analyze large datasets generated by fluorescence microscopy. This helps in achieving accurate interpretations rapidly.
- Miniaturization of Microscope Designs: Smaller and more portable microscope systems are being developed. This can make fluorescence microscopy accessible in field studies.
- Live Cell Imaging Advancements: Techniques for monitoring dynamic cellular processes in real-time are improving. This offers insights into cellular functions and interactions.
These trends reflect a shift towards more efficient, precise, and user-friendly microscopy tools.
Potential Impact on Research
The potential impact of these advancements in fluorescence microscopy on research is substantial. Here are some key areas where change may occur:
- Better Understanding of Disease Mechanisms: Improved imaging techniques will aid in elucidating the complex mechanisms underlying various diseases, from cancer to neurodegenerative disorders.
- Enhanced Drug Development: Fluorescence microscopy can help researchers track the interactions between drugs and their targets in live cells. This accelerates the process of drug discovery and testing.
- Interdisciplinary Applications: The integration of fluorescence microscopy with other scientific fields, such as nanotechnology and bioengineering, may lead to new methods for diagnosing and treating diseases.
- Training and Education Improvements: As new trends emerge, educational resources will adapt and provide training for students and researchers on innovative microscopy techniques.
Such advancements can redefine biological imaging.
End
In this article, the importance of fluorescence microscopy is underscored by its pivotal role in advancing biological imaging. It enables researchers to visualize and study cellular processes with remarkable clarity. Advances in this field have led to significant developments that not only enhance our understanding of complex biological mechanisms but also improve our capacity for clinical diagnostics and therapeutic applications.
Summary of Key Points
- Fundamental Principles: We explored the basic principles of fluorescence—excitation, emission, and the fluorescence phenomenon that are foundational to this imaging technique.
- Types of Microscopes: Various types of fluorescence microscopes were detailed, including widefield, confocal, TIRF, and multiview 3D microscopes, each with their specific applications and advantages.
- Advanced Techniques: Techniques such as FRAP, FRET, and super-resolution microscopy signify the depth and versatility of fluorescence methodologies, allowing for innovative approaches to research challenges.
- Applications: Fundamental applications in cell biology, neuroscience, and clinical diagnostics showcase the significant contributions of fluorescence microscopy in various domains.
- Innovations and Future Directions: Recent innovations, including novel fluorophores and AI integrations, illustrate the ongoing evolution of this field, while potential future trends promise to further expand its capabilities and impact.
In summary, fluorescence microscopy stands as a crucial tool in modern science, bridging gaps in understanding and opening new avenues for exploration.
Final Thoughts
As we conclude, it is clear that fluorescence microscopy is not merely a technique but a cornerstone of contemporary research in biological sciences. The ability to visualize and quantify biological processes at a molecular level has transformed how we study life. The ongoing advancements and diversification of this technology indicate a promising future. For students, researchers, and professionals, it is important to stay informed about industry trends and innovations to leverage fluorescence microscopy effectively in their respective fields. This understanding will foster continued progress and exploration in scientific disciplines, ultimately enhancing human knowledge and health.