Exploring Force Microscopy: Techniques and Applications
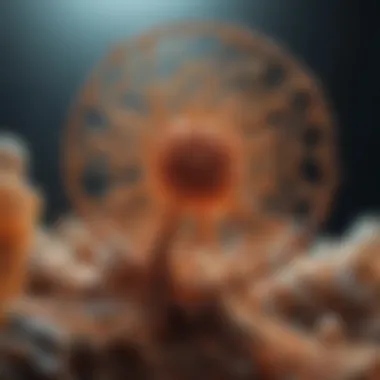
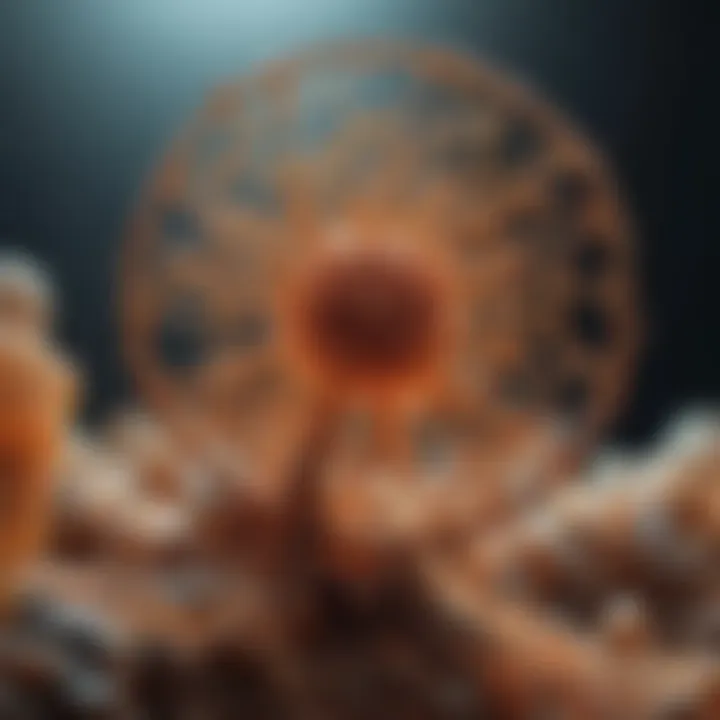
Intro
Force microscopy, particularly atomic force microscopy, has transformed how scientists examine materials at a nanoscale level. This remarkable technique allows researchers to probe individual molecules, cells, and various other structures with high precision. By utilizing a cantilever with a sharp tip, these systems can interact with surfaces to determine not only topography but also mechanical, electrical, and thermal properties. Understanding force microscopy is essential in several fields including biology, materials science, and nanotechnology, where advanced imaging and characterization are critical.
Research Overview
Summary of Key Findings
Recent studies have highlighted the versatility of force microscopy in various applications. For instance, in biology, researchers have elucidated cellular mechanisms by imaging the mechanical properties of cellular surfaces. In materials science, atomic force microscopy aids in the investigation of material fractures and surface roughness, providing insights crucial for developing stronger and more durable materials. In nanotechnology, precise control and manipulation at the atomic level have opened avenues for constructing novel nanostructures, influencing both academic research and industrial applications.
Importance of the Research in Its Respective Field
The research and developments surrounding force microscopy carry significant implications. Enhanced imaging techniques facilitate better understanding of complex biological processes, which can lead to breakthroughs in medical diagnostics and therapeutics. In nanotechnology, improved methodologies are crucial for innovative advancements in electronics and materials design. These insights underscore the importance of force microscopy in bridging theoretical research with practical applications.
Methodology
Description of the Experimental or Analytical Methods Used
Force microscopy employs various methods, primarily focusing on the interactions between the cantilever tip and the sample surface. The most widely used technique is the tapping mode, which minimizes damage to soft samples while providing high-resolution images. Another significant approach is contact mode, which measures the actual force exerted on the sample. Utilization of these methods demands careful calibration and environment control to reduce noise and improve accuracy.
Sampling Criteria and Data Collection Techniques
When conducting experiments, selecting an appropriate sample is critical. Samples can range from biological cells to nanomaterials, each requiring tailored preparation methods. Data collection typically involves monitoring changes in cantilever deflection and leveraging feedback mechanisms to maintain constant force. This precision allows for quantitative analysis of surface characteristics, enabling researchers to derive meaningful conclusions from their observations.
"Force microscopy reveals not just the structure but also the properties of materials at an unprecedented level of detail.β
By continuously advancing our knowledge of force microscopy techniques and their broad applications, researchers are effectively pushing the boundaries of what is scientifically achievable.
Preface to Force Microscopy
Force microscopy is a critical technique in various fields of scientific research. Understanding its principles and applications can illuminate much about nanoscale interactions and surface properties. It provides insights that are essential for the advancement of technologies in biology, materials science, and nanotechnology.
Definition and Historical Context
Force microscopy encompasses several techniques, with atomic force microscopy (AFM) being the most prominent. Developed in the 1980s by Gerd Binnig and Heinrich Rohrer, AFM marked a pivotal advancement in surface imaging and nanotechnology. This method allows scientists to visualize and manipulate materials at the atomic level. The development of these techniques has facilitated the examination of surfaces and their properties, linking them to broader scientific phenomena.
Fundamental Principles of Force Microscopy
The fundamental principle of force microscopy relies on measuring interaction forces between a sharp probe and the surface of a sample. The force exerted by the tip on the surface can vary depending on several factors such as distance, surface composition, and environmental conditions. Force microscopy utilizes a cantilever, which bends under the force exerted when the probe approaches the sample. By monitoring this bending, researchers can acquire detailed information about the sample at a nanometer resolution.
Force microscopy is versatile. It operates in various modes: contact mode, non-contact mode, and tapping mode. Each mode presents distinct advantages suited for different types of samples and specific detailed inquiry. This adaptability has made force microscopy a staple in modern experimental physics and material characterization.
"Understanding the interaction at the nanoscale can lead to breakthroughs in various scientific fields."
By combining the principles of mechanical force, this technique opens avenues for high-resolution imaging and analysis which are not accessible through traditional optical microscopy. In summary, the importance of force microscopy lies in its ability to unveil intricate details about materials and biological cells, ultimately driving innovation and discovery.
Types of Force Microscopy Techniques
The study of force microscopy techniques provides essential insights into the field of nanotechnology and materials science. Each type of force microscopy technique offers unique advantages, helping researchers to investigate surfaces at an atomic or molecular level. This depth of analysis possesses the potential to transform understanding across various scientific disciplines. By delineating the strengths and limitations of these techniques, we can appreciate their contributions to scientific research and technological advancements more profoundly.
Atomic Force Microscopy (AFM)
Atomic Force Microscopy (AFM) stands out as one of the most versatile techniques within force microscopy. Its capability to measure surface topography on a nanoscale is unmatched. AFM operates by scanning a sharp tip, usually made of silicon or silicon nitride, across the specimen's surface. The tip interacts with surface forces, providing incredibly detailed topographical images.
One of the advantages of AFM includes its ability to operate in various environments, including air, liquid, and vacuum. This feature is particularly beneficial for biological applications where samples need to remain in a physiological state. Additionally, AFM allows for the measurement of mechanical properties such as stiffness and adhesion, giving researchers insights into material behavior. Overall, Atomic Force Microscopy brings high resolution and versatility to the analysis of nanoscale structures, making it a significant tool in both materials science and biological research.
Magnetic Force Microscopy (MFM)
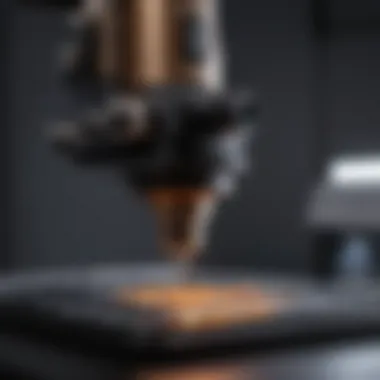
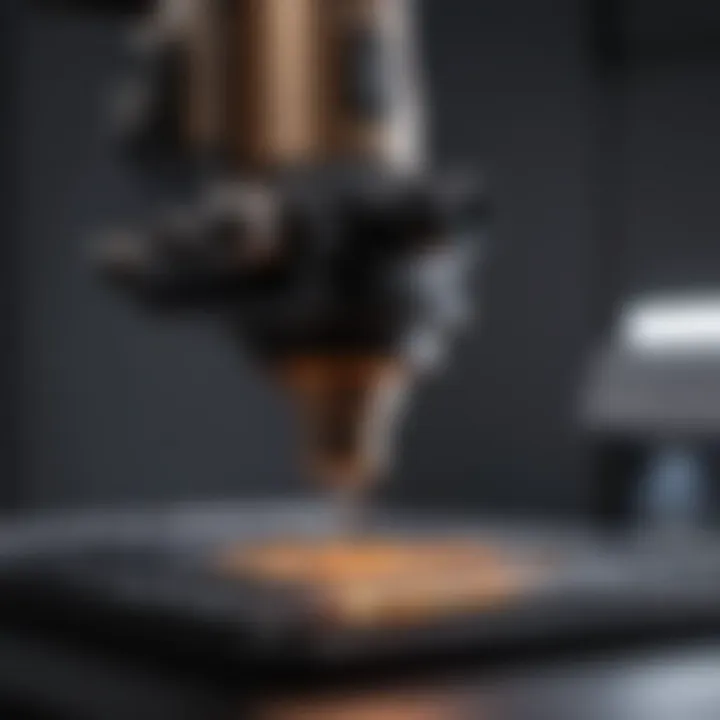
Magnetic Force Microscopy (MFM) is another technique that focuses on the magnetic properties of materials. In contrast to AFM, MFM is specifically designed to map magnetic fields at the nanoscale. This technique utilizes a magnetic tip to sense the magnetic interactions between the tip and the sample. MFM can provide detailed images of magnetic domains and their distributions in materials, which help understand various phenomena, including magnetic storage and spintronics.
MFM is advantageous in applications related to information technology and materials science. For instance, it can identify defects in magnetic materials and assist in developing advanced magnetic devices. By using MFM, researchers can access the magnetic properties of materials without destroying the sample, maintaining its integrity for further study.
Electrical Force Microscopy (EFM)
Electrical Force Microscopy (EFM) extends the capabilities of traditional force microscopy into the realm of electrostatics. EFM operates similarly to AFM but uses a conductive tip to measure variations in electrical forces between the tip and the sample. This capability allows EFM to measure surface charges and electric fields, thus unveiling critical information in semiconductor materials and nanoscale electronic devices.
The importance of EFM is particularly highlighted in the study of heterogeneous materials and their interfaces. Researchers can extract valuable data on charge distributions and local capacitance. Furthermore, EFM can provide information about ferroelectric materials, enhancing our understanding of their properties and behaviors. Given the growing relevance of electronics in modern technology, the role of Electrical Force Microscopy cannot be understated. Its application in both research and industrial settings underscores the integral nature of this technique in advancing knowledge in the field.
"Matrix of possibilities opens up when using different force microscopy techniques to understand materials at the nanoscale."
In summary, the distinct types of force microscopy techniques, namely AFM, MFM, and EFM, highlight the versatility and depth of analysis in this field. Each technique offers unique benefits and applications, underscoring the significance of force microscopy in scientific discovery. The integration of these methodologies enables researchers to explore new dimensions in their study of materials, pushing the boundaries of knowledge across multiple disciplines.
Operational Mechanics of Force Microscopy
Understanding the operational mechanics of force microscopy is essential for appreciating its role in advancing scientific research. These techniques provide a framework that underlies how force microscopy achieves high-resolution imaging and molecular-scale characterization. The operational mechanics encompass the methods used to sense interactions at the atomic or molecular level. Additionally, they highlight the importance of adequate sample preparation and environmental control, ensuring consistency and reliability in the results obtained.
Force microscopy approaches utilize different principles of interaction, which play a critical role in determining their effectiveness. From the specific sensing mechanisms to the procedures of sample preparation, each step in the process must be executed with precision to yield quality data, making this topic vital to the overall understanding of force microscopy and its effectiveness in various applications.
Sensing Mechanisms and Signal Detection
The sensing mechanisms in force microscopy are essential for accurately detecting forces between the probe and the sample. The choice of sensing mechanism depends on the specific technique employed, such as Atomic Force Microscopy (AFM) or Magnetic Force Microscopy (MFM). These techniques often use sensors like cantilevers or magnetic tips to measure forces resulting from interactions between charged or magnetic elements in the sample.
In AFM, for example, the cantilever bends when a force acts upon it, altering its deflection, which is measured using a laser beam focused on the back of the cantilever. This method allows for high-resolution topographical data and can also provide information about the mechanical properties of the sample. Similarly, in MFM, the probe detects magnetic interactions, enabling imaging of magnetic domains at the nanoscale.
The effectiveness of the sensing mechanism directly impacts the quality of data. Precise signal detection requires calibration and proper hardware setup. The sensitivity and resolution can be greatly influenced by factors like the probe's material and geometry, as well as the environmental conditions during imaging. These considerations also lead to better signal-to-noise ratios, resulting in clearer data representation.
Sample Preparation and Environmental Control
Sample preparation is another crucial aspect of the operational mechanics. The way a sample is prepared can significantly affect the accuracy of the measurements. For instance, biological samples may need to be immobilized on a substrate to prevent movement that could introduce variability in the data. In material science applications, ensuring a clean and defect-free surface can provide more reliable insights into material properties.
Environmental control is equally significant. Force microscopy is sensitive to ambient conditions. Variations in humidity, temperature, and vibrations can introduce noise that impacts measurements. To counteract this, many setups incorporate vibration isolation systems, as well as control over atmospheric conditions. Maintaining a stable environment ensures that the resulting data is reflective of the true characteristics of the sample rather than being skewed by external factors.
By focusing on these operational mechanics and their intricacies, researchers and practitioners can improve their use of force microscopy, yielding more reliable results across various scientific disciplines.
"The operational mechanics of force microscopy is not just about the devices used, but also the environments and preparation methods that enhance data integrity."
In summary, the operational mechanics should not be underestimated, as they constitute a foundation upon which the effectiveness and reliability of force microscopy rest.
Applications Across Disciplines
The versatility of force microscopy is evident through its applications across various scientific fields. Each discipline leverages the unique capabilities of this technique. Understanding these applications allows for deeper insights into complex materials and biological systems. The integration of force microscopy into research methodologies has enhanced the capability to analyze samples with great precision. This section will cover significant applications in biology, material science, and nanotechnology, showcasing the impact and relevance of this technique.
Biological Applications
Cellular Structure Analysis
Cellular structure analysis refers to examining the physical and structural characteristics of cells using force microscopy techniques. This process allows for the detailed visualization of cellular components at nanometer resolution. A key characteristic of this analysis is its ability to provide real-time imaging and manipulation of live cells. Thus, it becomes a beneficial choice for studies related to cellular dynamics.
A unique feature of this application is its capacity to discern differences in mechanical properties of various cellular structures. For instance, it can distinguish between the elastic behavior of different organelles inside cells. However, one disadvantage is that sample preparation can affect the observed outcomes if cells are not handled properly.
Protein Interactions Study
The protein interactions study examines how proteins interact with one another and with other biomolecules. This segment is crucial for understanding biochemical pathways and cellular functions. A defining characteristic is its sensitivity to molecular forces at play in these interactions. This sensitivity makes it an ideal tool for studying weak interactions that are often overlooked in other methods.
The unique feature of this application lies in its ability to quantify binding forces on a molecular scale. By evaluating these forces, researchers can derive insights into the stability and dynamics of protein complexes. Despite its advantages, challenges include potential interference from environmental factors during experiments, which can skew results.
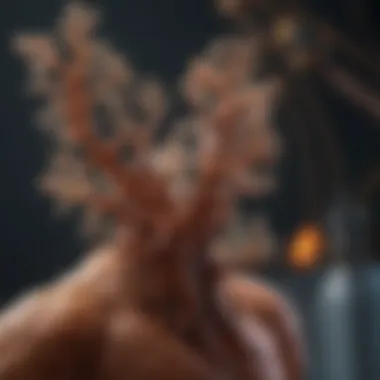
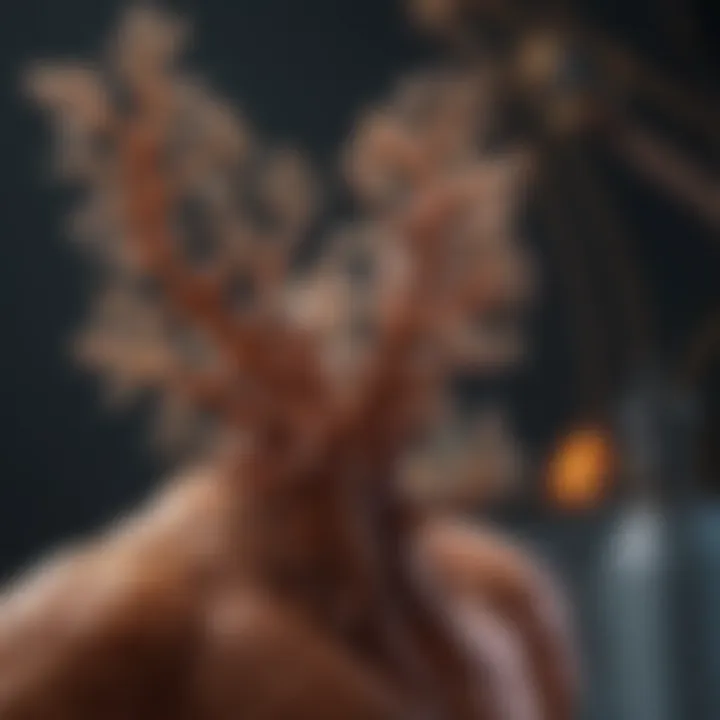
Material Science Applications
Nanostructure Characterization
Nanostructure characterization is essential for evaluating materials at the nanoscale. This application plays a crucial role in material science, helping researchers understand structural properties and defects in nanomaterials. A significant advantage is the precision it offers in mapping the topography and mechanical properties of materials.
One key characteristic of nanostructure characterization is the ability to study the surface qualities of materials under varying conditions. This adaptability makes it attractive for researchers developing new materials. However, the challenges include the requirement for sophisticated equipment and extensive calibration to ensure accurate readings.
Mechanical Property Assessment
Mechanical property assessment focuses on determining the physical behaviors of materials under different conditions. This process is vital for understanding material strength and durability, making it an important choice in material science. A defining aspect is its capability to generate force-displacement curves that detail how materials respond to applied forces.
The unique feature of mechanical property assessment is its applicability in both bulk materials and thin films. This flexibility is beneficial for a range of research and industrial applications. Nevertheless, limitations include sensitivity to external factors, which can vary the results.
Nanotechnology Applications
Nanoparticle Imaging
Nanoparticle imaging involves visualizing nanoparticles at high resolutions to study their properties and behaviors. This process is critical for applications ranging from drug delivery to material synthesis. A notable characteristic is its ability to provide real-time imaging, invaluable for tracking dynamic changes.
A unique feature of nanoparticle imaging is the capability to analyze interactions at the nanoscale. This feature allows understanding how nanoparticles interact with their environment, crucial for their applications in medicine and technology. However, the complexity of interpreting images can present challenges for researchers.
Surface Manipulation Techniques
Surface manipulation techniques refer to methods that allow researchers to modify surfaces at the nanoscale. This application is importan for developing customized materials with specific functionalities. A key characteristic is the ability to manipulate surface properties with high precision, making it a popular choice in nanotechnology.
The unique feature is its capacity for creating patterns or structures on surfaces, enabling advancements in nanofabrication. However, difficulties may arise in maintaining consistency across large areas, which can hinder practical applications.
Through the diverse applications across disciplines, force microscopy stands as a pivotal tool for advancing scientific knowledge and technological innovation.
Data Interpretation and Analysis
Data interpretation and analysis form the backbone of force microscopy studies. These processes enable researchers to derive meaningful insights from the data collected during experiments. Effective interpretation allows for a deeper understanding of the physical properties and behaviors of materials at the nanoscale. In force microscopy, particularly in techniques like Atomic Force Microscopy and Magnetic Force Microscopy, the quality of data analysis can significantly influence conclusions drawn about material characteristics and biological mechanisms.
A few key aspects concerning data interpretation in force microscopy include:
- Quantitative Analysis: The ability to quantify forces and structural properties enhances reproducibility and validity of results. This data is crucial for comparing experiments and for applications across various disciplines, including biology and materials science.
- Comparative Assessment: By comparing force-distance curves, significant differences in behaviors among various samples can be discerned. This is essential in applications like cellular interaction studies, where understanding minute differences is vital.
- Clear Visualization: Proper analysis leads to clear and effective visual representation of data, which facilitates better understanding among viewers. Topographical maps enable researchers to visualize surface characteristics and nanostructures in a comprehensible manner.
Effectively interpreting data also involves addressing potential limitations and errors introduced during the experimental process. This helps in refining methodologies for future research.
Force-Distance Curves
Force-distance curves are a fundamental element in analyzing interaction forces between a probe and a sample surface in force microscopy. These curves represent the relationship between the applied force and the distance the probe moves toward or away from the sample. The resulting data offers critical insights into adhesion forces, molecular interactions, and mechanical properties of materials.
During the measurement process, several phases can be characterized in a typical force-distance curve:
- Approach Phase: The probe moves towards the sample, and the force indicates the interaction type. This phase can show how molecules start to interact before contact is made.
- Contact Phase: Once the probe touches the surface, a rapid change in force might occur. This reaction can reveal key insights into surface properties and adhesion characteristics.
- Retracting Phase: As the probe is pulled away, the curve illustrates how forces diminish, providing insight into the molecular bonds that may be breaking. The analysis of this phase is crucial for understanding both reversible and irreversible interactions.
Topographical Mapping
Topographical mapping is an essential capability of force microscopy that provides valuable information about the surface structure of samples at the nanoscale. It delivers detailed images of surface features, helping researchers explore the physical topography of materials or biological samples. The generation of these maps relies on the interaction between the probe and the sample, where variations in height result in changes in detected forces.
Typical applications of topographical mapping include:
- Surface Roughness Assessment: Determining roughness is fundamental in materials science, as it can significantly affect properties like adhesion, friction, and optical performance.
- Feature Recognition: Identifying structures such as pores, fibers, or grains on a sample provides insight into the sample's composition and processing history.
- Nanostructural Analysis: In nanotechnology, mapping reveals how nanoscale structures conform to design specifications, which is crucial in developing new materials and devices.
Data from topographical maps is often used alongside other analyses to corroborate findings, enhancing overall research accuracy. Proper interpretation of these maps can lead to advancements in various fields by facilitating a more profound understanding of material properties and behaviors.
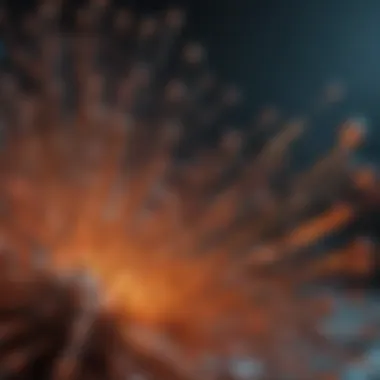
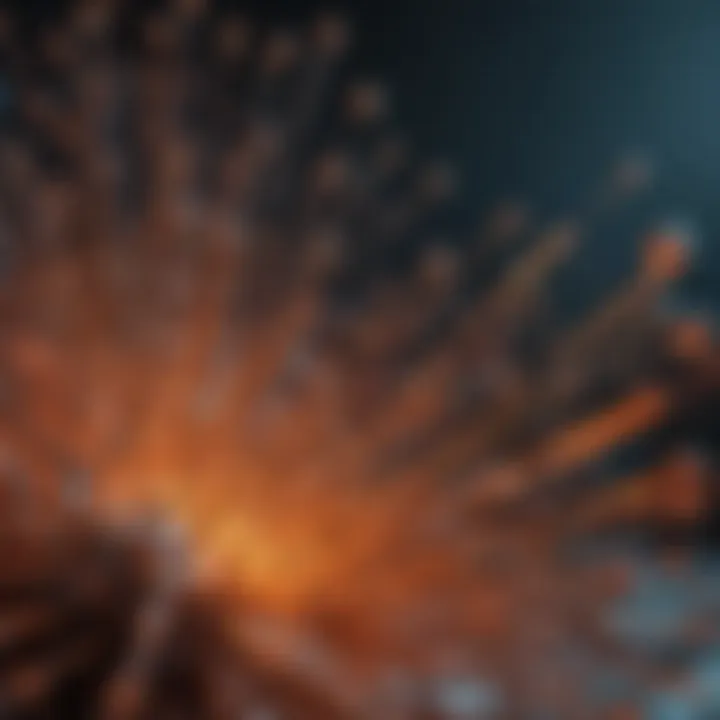
Challenges and Limitations of Force Microscopy
Force microscopy, while a powerful and versatile tool, faces several challenges and limitations that can impact its efficacy in various applications. Understanding these issues is crucial for researchers and practitioners who rely on this technology. A thorough analysis of these challenges can lead to better interpretation of data and the refinement of techniques used in force microscopy research.
Sensitivity to External Factors
One of the most significant challenges in force microscopy is its sensitivity to external factors. Variations in environmental conditions can cause notable fluctuations in the measurements obtained. For instance, vibrations from nearby machinery or even human activity can disturb sensitive measurements, leading to inaccuracies. Additionally, temperature fluctuations can affect the material properties under study, thereby skewing results.
Wind or air currents can also interfere with the measurement process. Force microscopy operates at a nanometer scale, where minor disturbances can have outsized effects. It becomes imperative for researchers to minimize external interference as much as possible. Employing vibration isolation tables and conducting experiments in controlled environments can mitigate some of these sensitivity issues.
Key Points to Consider:
- Environmental Control: Maintain stable conditions to enhance data integrity.
- Vibration Isolation: Use specialized tables or platforms to reduce disturbances.
- Temperature Regulation: Keep the operational temperature as constant as possible.
Sample Limitations
Another critical limitation of force microscopy is the nature of the samples being investigated. Certain materials may not be compatible with all types of force microscopy techniques. For instance, delicate biological specimens can be altered or damaged during analysis. The selection of probe tips is also vital; different materials exhibit various interaction forces that can impact the quality of the data obtained.
Moreover, sample preparation often necessitates specific protocols, which can be time-consuming and may introduce additional variables that affect results. Complex samples, such as those involving heterogeneous materials, present their own challenges. Observing interactions at the nanoscale with such samples requires precise and careful methodology.
Considerations:
- Sample Compatibility: Understand which techniques suit specific materials.
- Minimize Sample Preparation Bias: Take care to follow protocols closely and be aware of how they may affect outcomes.
- Probe Selection: Choose the right probe to match the interactions being studied.
"Recognizing the limitations of force microscopy allows researchers to set realistic expectations and to refine methodologies for better scientific outcomes."
Future Directions in Force Microscopy Research
Exploring the future of force microscopy research is vital. This field has been central to advancements in several scientific domains. Understanding future directions not only demonstrates the evolution of techniques but also sheds light on potential applications that could reshape current scientific paradigms. With the ongoing development in technology, it is important to highlight specific advancements and integrations that are promising.
Advancements in Instrumentation
The advancement in instrumentation plays a significant role in enhancing the capabilities of force microscopy. Research is pushing the boundaries of resolution, sensitivity, and speed in measurement. Current innovations focus on miniaturization and automation of the instruments. This means researchers can achieve higher resolutions while using simpler operational methods.
One of the impactful advancements is the development of high-speed atomic force microscopy. This technology allows for the real-time observation of dynamic processes at the nanoscale, which was not feasible before. For example, monitoring cellular interactions in real-time can lead to deeper insights into biological mechanisms.
Additionally, improving the tip design and coating materials used in force microscopy probes can provide better signal-to-noise ratios. Choosing the right materials can minimize artifacts that often complicate data interpretation. In essence, the enhancement of instrumentation enables more precise and reliable data acquisition, which is crucial for nuanced analysis.
Integration with Other Techniques
Integration of force microscopy with other techniques is another critical direction in research. Combining methodologies can enhance the overall data richness and contextual understanding. For instance, integrating atomic force microscopy with techniques like fluorescence microscopy can facilitate a more comprehensive view of sample interactions.
Such collaboration allows for simultaneous collection of topographical, mechanical, and biochemical information. This holistic approach can yield insights that are unattainable through individual methods alone. Moreover, integrating computational methods with force microscopy can improve data analysis and interpretation.
The use of machine learning to analyze force spectroscopy data is gaining traction. Algorithms can detect patterns in complex datasets much faster than manual methods. This empowers researchers to identify correlations that may not be immediately obvious.
"The future directions of force microscopy research lie in its capability to evolve through innovation and integration. This will potentially revolutionize the way we understand material and biological systems."
The End
The conclusion of this article serves as an essential synthesis of the numerous insights discussed regarding force microscopy. Analyzing the various techniques, their applications, and the implications of findings allows us to appreciate the scope and depth of this powerful scientific tool. As researchers and practitioners navigate through the complexities within fields such as biology, materials science, and nanotechnology, understanding these elements becomes increasingly critical.
Summary of Key Insights
Force microscopy encompasses a suite of techniques that provide unique advantages for probing material properties at the nanoscale. Here are some pertinent insights:
- Diverse Techniques: The article elaborates on methods like Atomic Force Microscopy (AFM), Magnetic Force Microscopy (MFM), and Electrical Force Microscopy (EFM), highlighting their specific operations and relevance to different research areas.
- Cross-Disciplinary Applications: Applications spread across various disciplines indicate force microscopy's integral role in modern science. Whether elucidating cellular components or characterizing nanostructures, the versatility of these techniques is evident.
- Analytical Insight: Understanding data interpretation, especially force-distance curves, and topographical mapping, is vital for making sound scientific conclusions.
- Challenges Identified: Acknowledging the limitations and challenges faced by force microscopy, encompassing sensitivity to environmental factors and sample constraints, provides a rational basis for future advancements.
Implications for Future Research
The future implications for research in force microscopy are vast and warrant attention. Here are some pivotal considerations:
- Technological Advancements: Ongoing developments in instrumentation will likely enhance the resolution and speed of gathering data, making force microscopy an ever-more powerful tool.
- Integration with Emerging Techniques: As we move forward, integrating force microscopy with other analytical techniques can provide more comprehensive insights. For example, coupling AFM with spectroscopic methods can yield richer data sets.
- Novel Applications: Exploring new materials and biological systems presents opportunities for discovery. Force microscopy may play a pivotal role in understanding complex interactions at the nanoscale, impacting fields such as drug delivery and materials engineering.
- Standardizing Protocols: Establishing best practices and standardization in measurements can enhance reproducibility and reliability across different research teams, facilitating collaborative efforts.