Exploring Molecular Cytogenetics: Concepts and Applications
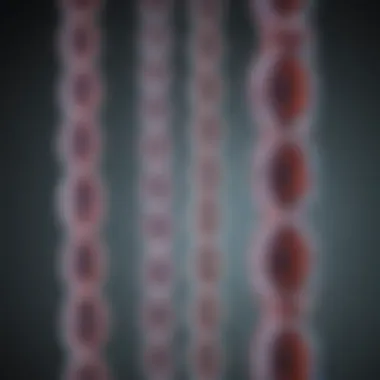
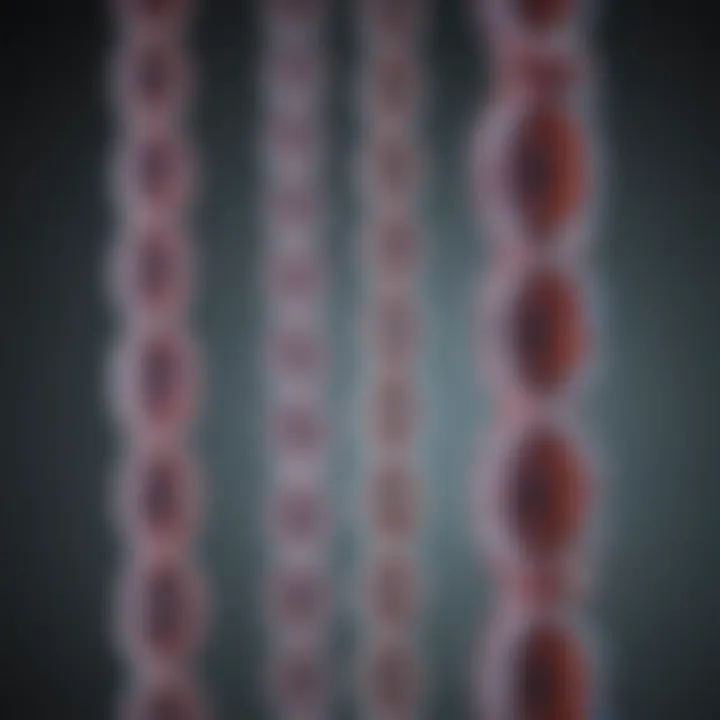
Intro
Molecular cytogenetics stands at the intersection of cytogenetics and molecular biology. This discipline provides insights into the behavior and structure of chromosomes, as well as their molecular components. In recent years, it has led to significant advances in understanding chromosomal abnormalities, which can have profound implications in various fields, particularly genetics and oncology.
As technology progresses, so does the potential for molecular cytogenetics to influence diagnostic and therapeutic strategies. The integration of tools like fluorescence in situ hybridization (FISH) and array comparative genomic hybridization (array CGH) has transformed our approach to resolving genetic anomalies and elucidating cancer mechanisms. Thus, this overview aims to highlight key developments, techniques, and applications of molecular cytogenetics.
Research Overview
Summary of Key Findings
Recent advancements in molecular cytogenetics have yielded remarkable findings. Researchers have discovered that detailed mapping of chromosomal regions can identify genetic markers associated with diseases. For instance, specific deletions or duplications within chromosomes can indicate predispositions to certain types of cancer.
Another crucial finding is the role of molecular markers in tracking disease progression. By employing techniques that allow real-time monitoring of chromosomal changes, scientists can better understand tumor heterogeneity and response to therapies.
Importance of the Research in Its Respective Field
The relevance of molecular cytogenetics cannot be overstated. It is instrumental in the following areas:
- Genetic Research: Facilitating the discovery of new genetic syndromes and understanding the pathology of known conditions.
- Oncology: Offering insights into tumor genetics, aiding in targeted therapies, and providing information to tailor treatment.
- Diagnosis: Enhancing the accuracy of diagnosing chromosomal abnormalities through refined methodologies.
This research is not just academic; it has practical implications in clinical settings, making it vital for the future of personalized medicine.
Methodology
Description of the Experimental or Analytical Methods Used
Molecular cytogenetic techniques are diverse. FISH, for instance, utilizes fluorescent probes that bind to specific DNA sequences on chromosomes. This allows visualization of chromosomal arrangements and any alterations.
Array CGH is another significant technique. It provides a high-resolution analysis of the entire genome, enabling the detection of copy number variations. These methods, among others, have revolutionized the ability to assess genetic material.
Sampling Criteria and Data Collection Techniques
In conducting molecular cytogenetic studies, sampling criteria are paramount. Samples often come from patient biopsies, blood draws, or cell cultures. These specimens must be carefully processed to ensure genomic integrity. Data is collected using rigorous protocols to maintain reliability, often involving high-throughput approaches that enhance the quality and scope of findings.
"Molecular cytogenetics is pivotal to unraveling the complexities of chromosomal behavior and its impact on human health."
The tools and methods in this field are continuously evolving, making adherence to precise sampling and analysis essential for cultivating accurate scientific knowledge.
Prolusion to Molecular Cytogenetics
Molecular cytogenetics represents an intersection of two significant fields—cytogenetics and molecular biology. This field is crucial for understanding the dynamic relationships between chromosomes and their molecular components. By integrating the principles of genetics, molecular biology, and cytogenetics, researchers develop a framework to study the structure, function, and behavior of chromosomes at a molecular level. The importance of molecular cytogenetics extends beyond mere academic curiosity. It has practical applications in clinical settings, such as diagnosing genetic disorders, identifying cancer-related chromosomal changes, and guiding treatment decisions.
Molecular cytogenetics enhances our comprehension of complex genetic interactions and provides insights into how chromosomal abnormalities manifest in various diseases. Recognizing the importance of this field allows scientists and clinicians to address pressing challenges in genetics and medicine. As techniques advance, the utility of molecular cytogenetics grows, making it an essential topic for this article.
Definition and Scope
Molecular cytogenetics can be defined as the study of the genetic material within cells by integrating molecular biology techniques to analyze chromosomal structures. This field provides a comprehensive understanding of chromosomal abnormalities by exploring the relationship between chromosome behavior and their genetic content. The scope of molecular cytogenetics encompasses various methodologies, including fluorescence in situ hybridization (FISH) and array comparative genomic hybridization (aCGH), allowing for precise investigation of chromosomal variations at both high resolution and scale.
The relevance of this field is underscored by its application in clinical diagnostics, particularly in identifying genetic predispositions to diseases and providing targeted cancer therapies. As technology advances, the continuous evolution of techniques enhances the depth of analysis and breadth of potential applications, thereby elucidating the intricacies of genetic disorders.
Historical Development
The historical trajectory of molecular cytogenetics is marked by several key milestones. The discipline emerged in the 20th century with the advent of cytogenetics, a field dedicated to understanding chromosomes and their functions. Early researchers focused primarily on identifying chromosomal abnormalities through microscopic examination of stained chromosomes.
In the 1970s and 1980s, advances in molecular biology propelled the field forward. Techniques such as DNA cloning and the polymerase chain reaction (PCR) laid the groundwork for molecular cytogenetics. The introduction of fluorescence in situ hybridization in the late 1980s allowed for the visualization of specific DNA sequences within chromosomes, revolutionizing the ability to identify chromosomal abnormalities in real-time.
As these techniques continued to develop, array comparative genomic hybridization emerged in the early 2000s. This offered a high-throughput method for analyzing chromosomal abnormalities, enabling the detection of copy number variations across the genome.
Over the years, molecular cytogenetics has gained recognition as an indispensable tool in clinical genetics, particularly in understanding cancer biology and diagnosing genetic disorders. The development of next-generation sequencing technologies has further extended the reach and utility of molecular cytogenetics into cutting-edge research domains.
Foundational Concepts
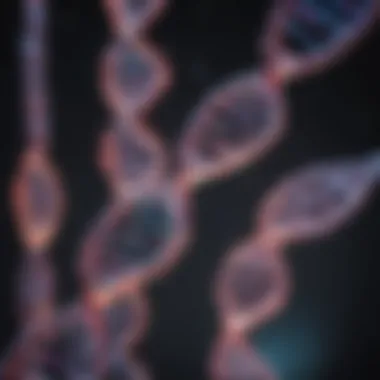
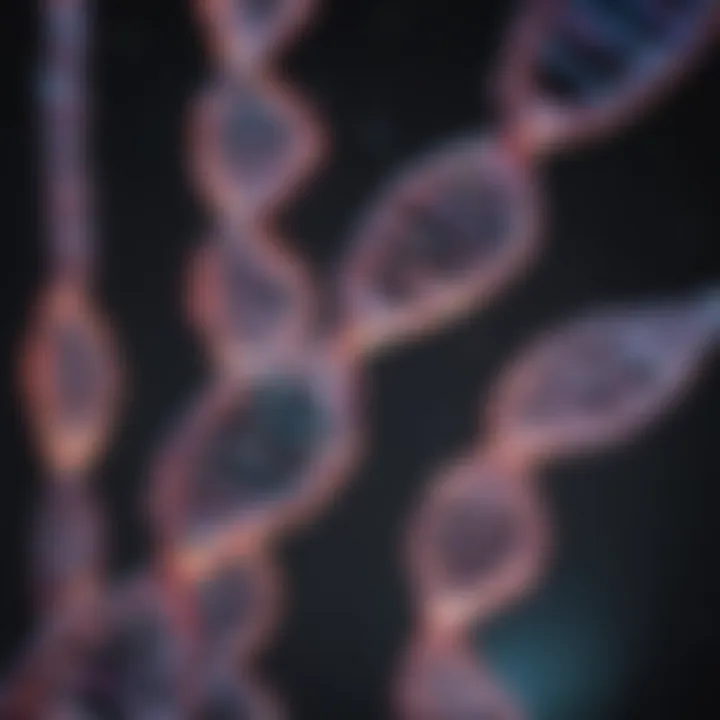
Foundational concepts are crucial to understanding molecular cytogenetics. This area combines cytogenetics, which studies chromosomes, with molecular biology, focusing on the interactions at the molecular level. By grasping these concepts, students and researchers can better appreciate how chromosome structure influences genetic function and stability. It also lays the groundwork for utilizing modern techniques that aid in diagnosing various genetic conditions.
Chromosomal Structure and Function
Chromosomes are the carriers of genetic information. Each chromosome consists of DNA tightly coiled around proteins called histones. This structure not only protects the DNA but also plays a vital role in gene regulation. The number of chromosomes varies across species; humans typically have 46. They are organized into 23 pairs, with one set inherited from each parent.
The principal functions of chromosomes include:
- Genetic Information Storage: Chromosomes serve as templates for genes, which are the instructions for making proteins essential for all cellular functions.
- Reproduction: During cell division, chromosomes ensure that genetic material is accurately copied and distributed to new cells.
- Regulation: The way chromosomes are structured can influence gene activity. For example, tightly packed DNA is often less accessible for transcription than loosely packed DNA.
In molecular cytogenetics, understanding the structure and organization of these chromosomes is vital. It explains how chromosomal abnormalities can lead to diseases like cancer or genetic disorders.
Genomic Architecture
Genomic architecture refers to the organization of genetic material within the nucleus. This structure is complex and involves not just chromosomes but also how they are spatially arranged. Each chromosome has regions that can be more or less compacted, impacting gene expression.
The significance of genomic architecture includes:
- Three-dimensional Structure: The spatial arrangement of chromosomes in the nucleus affects interactions between genes and regulatory elements. This structure can influence which genes are turned on or off.
- Chromatin Dynamics: Chromatin exists in two forms: euchromatin (less condensed, active in transcription) and heterochromatin (more condensed, generally inactive). The balance between these forms affects overall gene expression levels.
- Genomic Interactions: Chromosomes do not act in isolation; they interact with each other to regulate gene activity. These interactions can give insight into disease mechanisms and help identify potential therapeutic targets.
Molecular Techniques in Cytogenetics
Molecular techniques in cytogenetics form the backbone of modern genetic analysis. These methods allow researchers and clinicians to investigate chromosomal variations at a molecular level. By bridging cytogenetics and molecular biology, these techniques enhance our understanding of genetic disorders, cancer, and evolutionary biology. Their significance lies in the ability to reveal intricate details about chromosome structure, function, and genetic information that were not previously accessible through traditional karyotyping. This section will focus on key methodologies including Fluorescence In Situ Hybridization (FISH), Array Comparative Genomic Hybridization (aCGH), and Next-Generation Sequencing Technologies.
Fluorescence In Situ Hybridization (FISH)
Principle of FISH
Fluorescence In Situ Hybridization, or FISH, relies on the hybridization of fluorescent probes to specific DNA sequences on chromosomes. This technique allows for the visualization of chromosomal aberrations, such as deletions, duplications, and translocations. One key characteristic of FISH is its ability to target specific regions of interest within the genome, providing insights into chromosomal composition. This precision makes it a popular choice for diagnostic purposes. FISH is beneficial because it allows for the direct observation of chromosomes in their natural context, which is crucial for understanding genetic disorders. However, it does have limitations. For instance, FISH requires prior knowledge of the target sequences and may not detect all types of chromosomal abnormalities.
Applications of FISH in Diagnostics
FISH has numerous applications in clinical diagnostics. It is particularly effective in identifying genetic disorders, where it can pinpoint specific chromosomal abnormalities linked to conditions like Down syndrome or chronic myeloid leukemia. A major benefit of FISH is its rapid turnaround time compared to more traditional methods. Moreover, FISH can be used on various types of samples, including blood, bone marrow, and biopsy tissue. However, FISH results must be interpreted carefully. The presence of abnormal signals can be challenging to analyze and may require confirmation through additional testing methods.
Array Comparative Genomic Hybridization (aCGH)
Methodology
Array Comparative Genomic Hybridization (aCGH) is a powerful tool that allows for genome-wide analysis of chromosomal alterations. The methodology involves labeling DNA from the test sample and reference sample with different fluorescent dyes, then hybridizing them to a microarray containing known DNA sequences. This process provides an overview of copy number variations across the genome. One notable aspect of aCGH is its high resolution, enabling detection of smaller genomic changes compared to traditional methods. The advantages of aCGH include its ability to analyze multiple regions of the genome simultaneously, making it efficient for large-scale studies. Nonetheless, aCGH has its limitations, particularly when it comes to identifying balanced rearrangements or small mutations that might not alter copy number.
Interpretation of Results
Interpreting results from aCGH requires careful analysis to determine the clinical significance of detected genomic imbalances. The key characteristic of aCGH interpretation lies in differentiating between benign variations and pathogenic alterations. This assessment plays a critical role in making informed clinical decisions. The unique feature of aCGH is its ability to provide a comprehensive view of the genome, which can aid in diagnosing a range of genetic conditions. Nevertheless, the growing database of genomic variants makes interpretation increasingly complex. False positives and unknown significance findings present ongoing challenges that must be navigated in clinical practice.
Next-Generation Sequencing Technologies
Next-Generation Sequencing (NGS) represents a revolutionary approach in molecular cytogenetics, facilitating the sequencing of entire genomes at unprecedented speed and volume. NGS can detect a variety of genetic alterations, including single-nucleotide variants, structural variations, and copy number variations, thereby enriching our understanding of genetic disorders and cancers. Model systems and technologies such as Illumina sequencing offer improved throughput and cost-effectiveness, making NGS a preferred choice for many research and diagnostic applications. As NGS continues to evolve, it promises to enhance insight into complex genetic phenomena, though challenges related to data interpretation and ethical considerations remain significant.
Applications of Molecular Cytogenetics
Molecular cytogenetics plays a pivotal role in understanding and applying genetic information across various fields. Its applications are broad, impacting clinical diagnostics, oncology, and research endeavors. This section explores the major applications of molecular cytogenetics, highlighting their contributions and some challenges they bring.
Clinical Diagnostics
Identification of Genetic Disorders
Identifying genetic disorders is one key application of molecular cytogenetics. This process involves detecting chromosomal abnormalities, such as duplications or deletions that can lead to conditions like Down syndrome or Turner syndrome. The ability to pinpoint these disorders accurately is vital for patient care and genetic counseling.
A significant characteristic of this identification method is its capacity for high-resolution analysis. Techniques such FISH or aCGH provide detailed insights into chromosomal structures, enabling clearer diagnoses. This high level of resolution makes it a popular choice for clinicians. An important aspect is how these techniques benefit early diagnosis, which can facilitate timely intervention.
Nonetheless, there are disadvantages as well. Sometimes, the results can be ambiguous. Not all detected changes have clinical significance, causing potential confusion for patients and clinicians alike. Balancing thorough analysis with clear communication is essential.
Prenatal Diagnosis
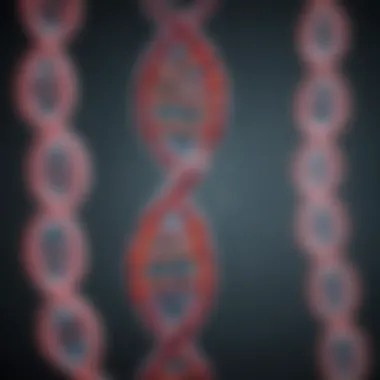
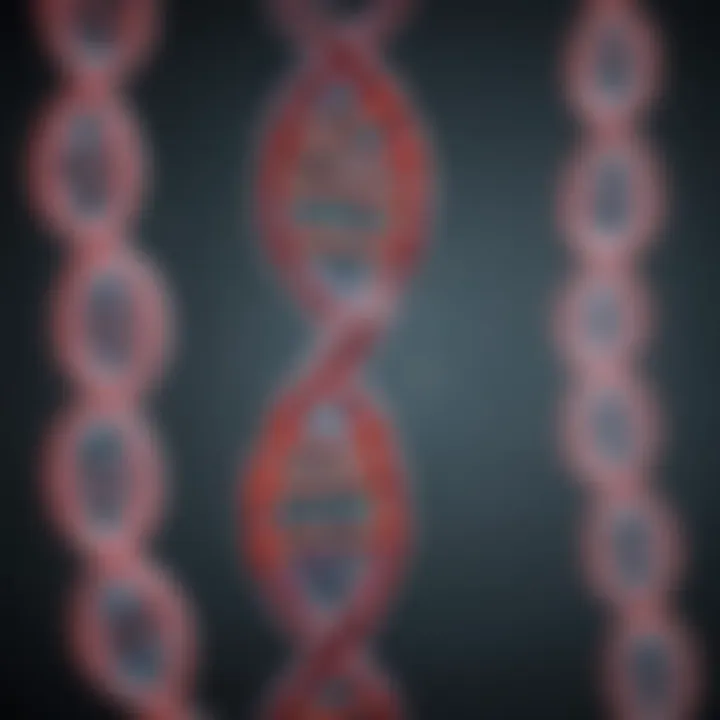
Prenatal diagnosis is another critical aspect of clinical diagnostics in molecular cytogenetics. This approach allows scientists and clinicians to evaluate the genetic health of a fetus before birth. Techniques can be non-invasive, like cell-free fetal DNA analysis, or more invasive procedures such as amniocentesis.
The ability to provide information about genetic disorders before birth is its primary advantage. This early insight can help parents make informed decisions. The unique feature of prenatal diagnosis is its ability to identify conditions early, which is crucial for preparing medical care and support.
However, with this technology comes the ethical consideration. The option for enhancing genetic health also raises questions about selective practices. This debate is important to consider in the context of prenatal diagnostics in a rapidly evolving field.
Oncology
Detection of Tumor-Specific Aberrations
In oncology, detecting tumor-specific aberrations is a significant application of molecular cytogenetics. Tumors often have distinct genetic profiles, which can be analyzed through various techniques. This identification is crucial for understanding cancer biology and tailoring effective treatment plans.
The key characteristic is that these aberrations can be specific to individual tumors, leading to personalized treatments based on the genetic makeup of the cancer. This targeted approach has revolutionized oncology practices, allowing for more effective interventions than generalized treatments.
However, a challenge remains in the need for continuous monitoring. Tumor evolution can lead to changes in genetic profiles over time, and therapies may need adjustments as a result. This dynamic nature of cancer genetics requires constant adaptation by healthcare providers.
Personalized Medicine Approaches
Personalized medicine approaches integrate molecular cytogenetics into cancer treatment. This strategy utilizes individual genetic data to tailor therapies for maximum effectiveness. The fundamental characteristic of personalized medicine is its reliance on genetic information to become more targeted and specific in treatment.
The benefit of this approach is that it enhances treatment outcomes, as therapies are designed around the patient’s unique genetic needs. However, it can also lead to complexities in management. Not every patient will respond positively to targeted therapies, creating a gap in expected outcomes. Understanding these nuances is crucial for practitioners.
Research Applications
Gene Mapping and Localization
Gene mapping and localization are foundational elements within research applications of molecular cytogenetics. Identifying the location of genes on chromosomes provides insights into their functions and interactions. This information is vital in establishing connections between specific genes and phenotypes.
An important feature of gene mapping is the ability to elucidate gene functions based on their relative positions. This underpinning characteristic allows scientists to hypothesize about gene roles in various biological processes. However, achieving precise localization can be challenging due to genomic complexity. It requires meticulous techniques to ensure accuracy, which can be resource-intensive.
Evolutionary Studies
Molecular cytogenetics also finds significance in evolutionary studies. This application involves examining chromosomal alignments across species to understand evolutionary relationships. By analyzing genomic stability and chromosomal changes over time, researchers can glean insights about evolutionary processes.
A notable characteristic here is its ability to provide a timeline for evolutionary events. This understanding helps reconstruct phylogenetic trees, which are valuable in studying the history of life on Earth. Nevertheless, the potential for misinterpretation persists. Different methodologies might score chromosomal features variably, causing possible discrepancies in conclusions drawn from such studies.
In sum, the applications of molecular cytogenetics are expansive and impactful. They have transformed clinical practice, personalized medicine, and research, providing deeper insights into genetic underpinnings across different domains.
Challenges in Molecular Cytogenetics
Molecular cytogenetics encompasses a range of methodologies and techniques, but it is not without its challenges. Understanding these challenges is crucial for students, researchers, and professionals as it shapes future directions in the field. From technical obstacles to interpretational complexities, acknowledging these issues allows for deeper insights into the reliability and applicability of research findings. Moreover, tackling such challenges can foster innovation and better methodologies, underscoring their significance in driving the entire field forward.
Technical Limitations
The realm of molecular cytogenetics faces numerous technical limitations. One primary concern is the resolution of the techniques employed. For instance, while Fluorescence In Situ Hybridization (FISH) is adept at detecting large chromosomal alterations, it often falls short in identifying smaller, submicroscopic changes. Consequently, relying solely on this method may lead to incomplete or misleading conclusions.
Additionally, sample quality can severely affect results. Poorly preserved samples can result in degraded DNA, compromising the accuracy of techniques such as aCGH. These limitations necessitate stringent protocols for sample collection and storage, further complicating the research process.
Moreover, the high complexity of genomic rearrangements poses another hurdle. Many alterations may have similar phenotypic outcomes, making it challenging to ascertain their clinical significance solely based on genetic findings. Researchers must often traverse this ambiguity, requiring a combination of genetic and clinical insights to arrive at sound conclusions.
Interpretational Challenges
Interpreting molecular cytogenetic data demands a level of expertise that is continually evolving. As new techniques arise, the information gained can be complex and sometimes contradictory. For example, next-generation sequencing generates vast amounts of data, but discerning meaningful biological implications from this data can be daunting. This influx of information can overwhelm researchers and clinicians alike, leading to potential oversights.
There is also the issue of variant classification. Not all genetic variants are well understood. Some may be benign, while others carry substantial risk of disease. Distinguishing between these can prove to be a significant hurdle. Many researchers rely on databases and literature to inform their interpretations, but these resources may not be comprehensive or up-to-date. This can lead to inconsistent interpretations among different labs and, ultimately, affect patient outcomes.
"Interpretation of molecular cytogenetic results can sometimes be as challenging as generating the data itself."
Lastly, the ethical implications surrounding the use of molecular cytogenetic findings must be acknowledged. Questions about privacy, informed consent, and the implications of genetic knowledge on patients and families can complicate interpretation. As researchers navigate these challenges, they must remain conscious of the broader ethical landscape surrounding their work.
Recent Advances in Molecular Cytogenetics
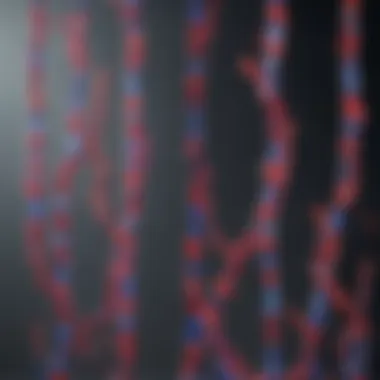
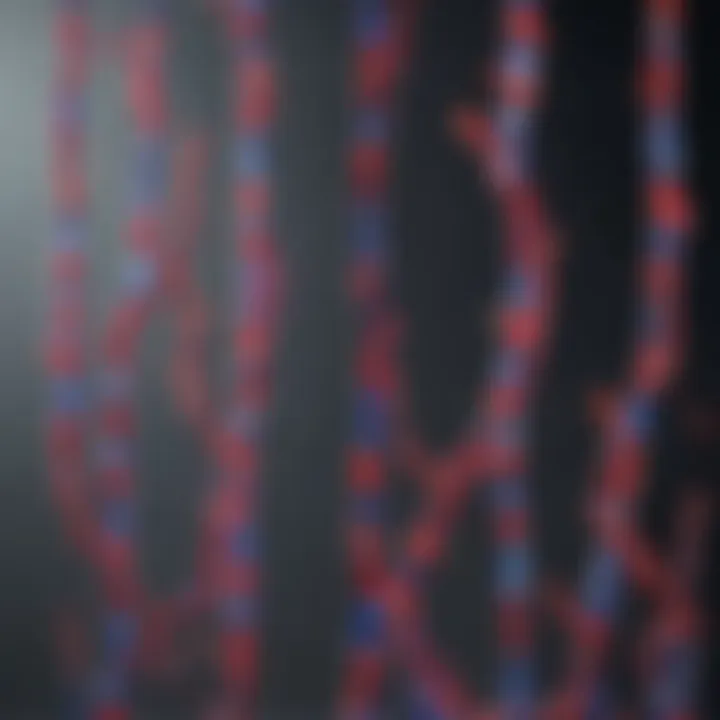
Recent advances in molecular cytogenetics have significantly reshaped the landscape of genetic research and diagnostics. This field combines classical cytogenetics with the insights provided by molecular biology. The ultimate goal is to offer a more detailed understanding of chromosomes at the molecular level. These advancements are crucial for uncovering the complexities of genetic disorders and other chromosomal aberrations.
One key aspect of these advances is the development of more precise molecular techniques. Techniques such as high-resolution array comparative genomic hybridization and improved fluorescence in situ hybridization methods have emerged. These innovations allow researchers to detect chromosomal anomalies with greater accuracy and at a much higher resolution than traditional methods could achieve. Consequently, this fosters not only a more accurate diagnosis but also a better understanding of the genetic basis of various diseases.
Furthermore, advances in next-generation sequencing technologies, such as whole exome sequencing, enable comprehensive analyses of genomic variation. This sort of technology can easily identify mutations that are either inherited or somatic in nature, making it particularly relevant in cancer genomics. As molecular cytogenetics integrates with these newer methods, the ability to generate vast amounts of data paves the way for novel insights into genetic functions and relationships.
"The integration of molecular techniques with cytogenetic methods is a game changer for genetic diagnostics and research."
The importance of these advancements cannot be overstated. They lead to more accurate diagnostic capabilities, personalized treatment plans, and enhanced understanding of disease mechanisms. With each new technique and discovery, the potential for research expands, pushing boundaries and allowing deeper explorations of genetic pathology.
Emerging Techniques
Emerging techniques in molecular cytogenetics hold significant promise for the future of the field. These cutting-edge methods provide powerful tools for exploring complex genetic questions and can lead to breakthroughs in understanding human health and disease.
One such emerging technique is the use of whole genome sequencing. It allows for not only the detection of large-scale genomic rearrangements but also for identifying smaller mutations that may contribute to genetic disorders or cancer. This kind of thorough analysis provides a complete view of an individual’s genomic landscape, which is invaluable for precision medicine.
Another noteworthy development is the advent of single-cell sequencing technologies. Traditional methods often analyze bulk tissue samples, which can mask the heterogeneity present at a single-cell level. With single-cell sequencing, researchers can pick apart these differences, leading to a better appreciation of how different cells may contribute to disease processes such as tumorigenesis.
Case Studies of Novel Discoveries
Several case studies illustrate the impact of advances in molecular cytogenetics. For example, researchers at Stanford University utilized advanced array comparative genomic hybridization to identify a novel chromosomal alteration associated with a rare genetic disorder called Smith-Magenis syndrome. This finding not only clarified the genetic basis of the condition but also helped in developing strategies for early diagnosis and family counseling.
Another case study involved the analysis of pediatric tumors, where next-generation sequencing was used to uncover recurrent genetic alterations in neuroblastoma. Understanding these mutations can lead to tailored treatments, making significant improvements in outcomes for affected children.
In summary, recent advances in molecular cytogenetics are not merely innovations; they represent a fundamental shift in how we understand and approach genetic diseases. Such progress highlights the need for continued research in this area, as the potential for novel discoveries continues to grow.
Future Directions in Molecular Cytogenetics
The landscape of molecular cytogenetics is continuously evolving, driven by advances in technology and the increasing complexity of biological systems. Understanding future directions in this field is vital for students, researchers, educators, and professionals who are engaged with genetic research and clinical applications. This section explores various elements such as integrative approaches and potential research pathways that can enhance the capabilities of molecular cytogenetics.
Innovations in this discipline are crucial not only for enhancing diagnostic methodologies but also for increasing our understanding of genetic disorders and cancer biology. The integration of multi-omics data and novel analytical techniques will likely reshape how researchers approach genome analysis and understanding chromosomal functions. Emphasizing these directions ensures that molecular cytogenetics remains relevant in both academic and clinical settings.
Integrative Approaches
Integrative approaches in molecular cytogenetics involve the combination of various genetic, epigenetic, and environmental data to provide a holistic view of genome function. By integrating different omics layers, such as genomics, transcriptomics, and proteomics, researchers can elucidate the interactions among biological systems more effectively.
- Big Data Analysis: The rise of big data analytics allows for the examination of vast datasets through advanced computational techniques, facilitating more precise predictions regarding genetic variations and their implications.
- Machine Learning Application: Employing machine learning models can optimize the analysis of cytogenetic data. These models can uncover subtle patterns and associations in large datasets that traditional methods may not detect.
- Collaborative Research: Collaborative efforts across disciplines enhance the interpretation of cytogenetic findings. When molecular biologists, clinicians, and data scientists work together, it increases the likelihood of discovering significant associations between genetic variations and phenotypic outcomes.
Adopting integrative approaches helps in tailoring personalized medicine strategies, ultimately improving clinical outcomes for patients affected by genetic disorders or cancers.
Potential Research Pathways
The future of molecular cytogenetics also hinges on the exploration of potential research pathways that address the critical questions in the field:
- Chromosomal Instability in Diseases: Future studies can focus on the mechanisms underlying chromosomal instability in cancer and genetic disorders. Understanding these pathways can inform therapeutic targets, leading to more effective treatments.
- Functional Genomics of Abnormalities: Researching the functional impact of specific chromosomal abnormalities can provide insights into how these changes contribute to disease pathways. Combining molecular cytogenetics with functional assays can enhance the relevance of findings.
- CRISPR and Genome Editing: The adoption of CRISPR technology opens new avenues for research. Assessing the impact of targeted genome editing on chromosomal structures could provide answers to longstanding questions about gene function and regulation.
The evolution of this discipline will undoubtedly lead to more refined diagnostic protocols and transformative therapies, which will shape the future of genetic research and patient care.
The End
The conclusion of this article synthesizes the critical elements and findings within the realm of molecular cytogenetics. This field has emerged as a cornerstone in modern biological research, bridging the gap between cytogenetics and molecular biology. Understanding the intricate relationships between chromosomes and their molecular constituents offers profound insights into genetic disorders and enhance diagnostics.
Summary of Key Points
In reviewing molecular cytogenetics, several key aspects emerge:
- Definition and Importance: Molecular cytogenetics combines the principles of cytogenetics and molecular biology, making it crucial for understanding genetic abnormalities and disease mechanisms.
- Methodologies: Techniques such as Fluorescence In Situ Hybridization (FISH) and Array Comparative Genomic Hybridization (aCGH) are foundational for diagnosing genetic conditions and conducting genomic research.
- Applications: The approach has substantial applications in clinical diagnostics, oncology, and genetic research, highlighting its versatility in addressing various medical issues.
- Challenges and Advances: While certain technical limitations exist, ongoing innovations and case studies continually push the field forward, demonstrating its potential.
- Future Directions: Integration of advanced methodologies will likely lead to enhanced understanding and new opportunities in genetic research and therapies.
The Importance of Continued Research
Continued effort in research is fundamental to unlocking the full potential of molecular cytogenetics. As this field advances, researchers must address multiple factors:
- Novel Discoveries: The dynamic nature of genetic research necessitates persistent exploration of new techniques and theories.
- Improved Techniques: Each advance holds promise for refining diagnostic processes and enhancing our understanding of chromosomal abnormalities.
- Broader Implications: As society faces an increasing burden of genetic diseases, research in this area becomes paramount for developing effective therapies.
The intersection of technological innovation and research in molecular cytogenetics serves as a model for addressing complex biological questions. Emphasizing this field's evolution and significance will foster a deeper understanding and encourage further investigation, ultimately benefiting both academic inquiry and clinical practices.
"Understanding molecular cytogenetics not only improves diagnostic capabilities but also enriches our approach to personalized medicine in response to genetic conditions."
Continued commitment to molecular cytogenetics research will provide the foundation for groundbreaking advancements that can significantly transform genetic medicine.