Exploring RNase A: Mechanisms and Significance
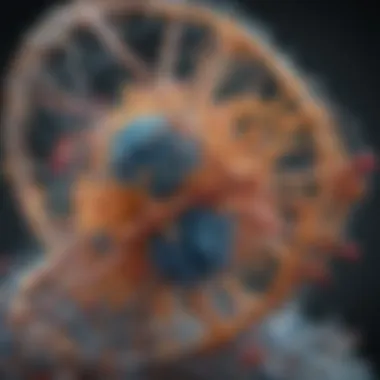
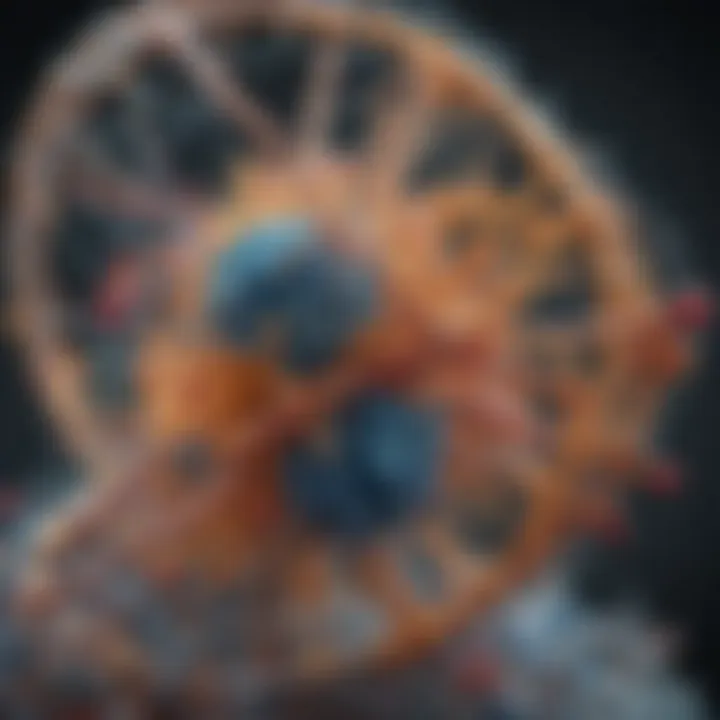
Intro
RNase A, a quintessential enzyme in the realm of molecular biology, plays a pivotal role in numerous cellular processes. Its influence spans from basic nucleic acid metabolism to complex regulatory pathways. This article aims to unpack the intricate workings of RNase A, emphasizing its structure, catalytic activity, and functional significance within the biological sphere. We will also explore how this seemingly simple enzyme connects with broader research implications and therapeutic advancements.
Research Overview
Summary of key findings
Research surrounding RNase A has unveiled an array of fascinating insights. At its core, RNase A exhibits a robust ability to cleave RNA, a property that is not only essential for maintaining cellular equilibrium but also vital in various biochemical applications. One of the notable findings is its capability to interact with RNA substrates in a highly specific manner, ensuring that only targeted molecules undergo hydrolysis. The enzyme is known for its high catalytic efficiency, which is attributed to its unique structural conformation that facilitates substrate binding and turnover.
Additionally, studies have revealed that RNase A does not operate in isolation. Instead, it often works in concert with other cellular components, enhancing its functional capacity. This synergistic interaction highlights not only the enzyme’s versatility but also its evolutionary significance in different biological contexts.
Importance of the research in its respective field
The research on RNase A holds immense value in various fields including molecular biology, genetics, and even therapeutic development. Understanding the mechanisms of RNase A has potential implications in RNA-based technologies, such as CRISPR and RNA interference, which are gaining traction in gene editing and therapy. Moreover, its role in RNA turnover and processing underscores its importance in maintaining genomic integrity and regulating gene expression.
By dissecting the mechanisms of RNase A, researchers can bridge theoretical knowledge with practical applications, paving the way for innovations in drug development and biotechnological advancements.
Methodology
Description of the experimental or analytical methods used
To unravel the complexities surrounding RNase A, a blend of experimental approaches has been employed. Structural biologists have utilized X-ray crystallography and nuclear magnetic resonance (NMR) spectroscopy to elucidate the three-dimensional configuration of the enzyme. These techniques allow scientists to visualize the atomic arrangement within RNase A, offering insights into how its structure contributes to its function.
Moreover, kinetic assays have been performed to assess the enzyme's catalytic activity. By measuring the rate of RNA cleavage under different conditions, researchers can pinpoint the factors that influence RNase A's efficiency. Such studies often involve varying substrate concentrations and environmental conditions, which helps to chart a comprehensive profile of the enzyme's operational dynamics.
Sampling criteria and data collection techniques
When conducting research on RNase A, specificity in sampling is crucial. Typically, the enzyme is sourced from bovine pancreas, where it is abundantly produced. The sampling criteria focus on ensuring the enzyme's purity and activity, often involving purification techniques like affinity chromatography. Data collection integrates high-performance liquid chromatography (HPLC) to analyze the products of RNA hydrolysis.
Through these methodologies, the extensive operational narrative of RNase A begins to unfold, allowing for a deeper exploration into its characteristics and wider implications in biological systems.
Prologue to RNase A
Understanding RNase A is crucial for grasping its role in molecular biology, particularly in the realms of RNA metabolism and cellular defense mechanisms. This enzyme, a staple in biochemistry, provides insight into how cells manage RNA and respond to various stresses. In the following sections, we will encapsulate its definition, historical significance, and its far-reaching implications in biological processes.
Definition and Historical Context
RNase A, or ribonuclease A, is a specific type of enzyme that catalyzes the degradation of RNA into smaller components. Its primary action involves cleaving the phosphodiester bonds in RNA molecules, which are essential for maintaining RNA’s structural integrity. Discovered in the early 20th century, RNase A has since been a focal point in enzymology studies. In particular, its relationship with the broader family of ribonucleases showcases the evolution of enzyme function.
Historically, the study of RNases began when researchers were exploring the effects of pancreatic extract on RNA. The isolation of RNase A from bovine pancreas by William Kaunitz highlighted its significant functions. Later, in the 1950s, scientists like Sidney Brenner and François Jacob considered RNase A as a model system for understanding enzyme kinetics, which has paved the way for modern molecular biology techniques. This historical backdrop not only reveals the enzyme's journey but also underscores its essential nature in research and practical applications.
Importance in Molecular Biology
Within molecular biology, RNase A holds a position of notable importance. Its catalytic function is integral for the dynamic regulation of RNA within cells. Here are some key roles that emphasize its significance:
- RNA Processing: RNase A is pivotal in the maturation of various RNA molecules, contributing to the separation of introns and exons, a crucial aspect of gene expression.
- Responses to Viruses: The enzyme helps defend the cell against viral infections by degrading viral RNA, which can enhance cellular resilience.
- Biochemical Research Tool: It has been widely used in laboratories to remove RNA from DNA preparations, facilitating the study of genomes.
The ability of RNase A to act on a wide array of RNA substrates makes it a valuable tool for researchers delving into RNA biology. By understanding its properties and mechanisms, scientists can employ this knowledge in various fields, from medicine to agricultural research.
"RNase A epitomizes the elegance of nature's biochemical tools, bridging fundamental research and practical innovations in molecular biology."
In summation, exploring RNase A helps illuminate the foundational concepts around RNA and its multifaceted roles in life processes. The historical insights and its vital functions lay a solid platform for the deeper exploration of its structural characteristics, catalytic mechanisms, and functionality that will follow in this article.
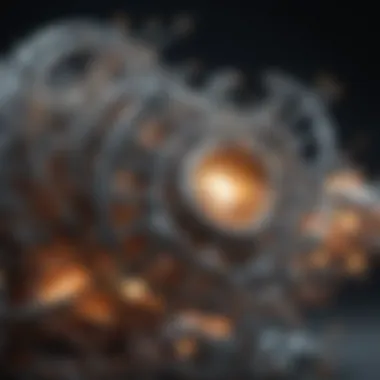
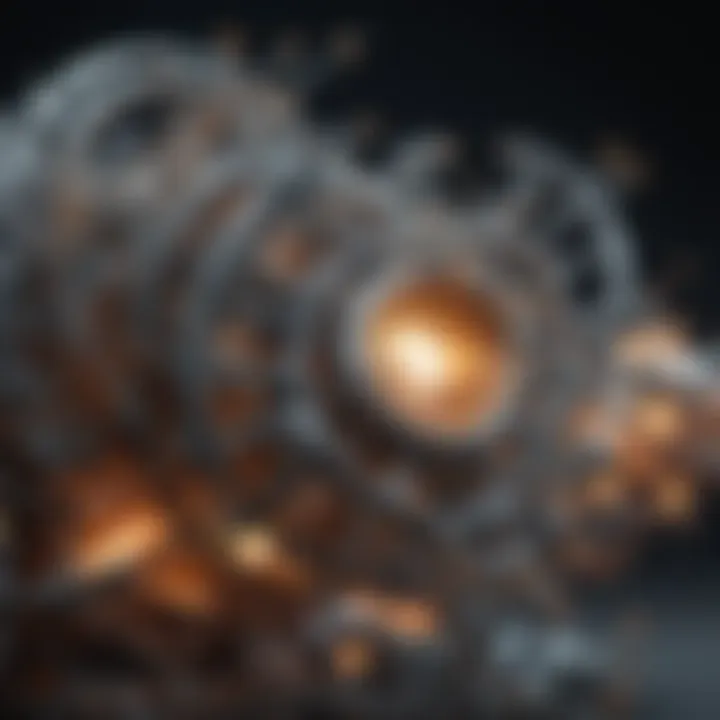
Structural Characteristics of RNase A
The structural characteristics of RNase A play a crucial role in its functionality and significance in molecular biology. This section sheds light on how the enzyme's unique architecture facilitates its functions in RNA metabolism and its vital contributions in various cellular processes. Understanding these structural nuances provides insight into how RNase A operates on a molecular level, thus emphasizing its importance within biological contexts.
Overall Structure and Folding
RNase A is a globular protein with a compact, well-defined structure. It typically consists of a single polypeptide chain that is folded into a specific three-dimensional shape, essential for its activity. The integrity of this structure is maintained through several types of interactions, including hydrogen bonds, ionic bonds, and hydrophobic interactions.
A notable feature is its characteristic core structure, which contains four helices and a bundle of beta strands. This arrangement is critical because it forms a stable scaffold that supports the enzyme's active site where RNA cleavage occurs. Efficient folding is necessary for RNase A to be functional. Misfolded proteins often become inactive and can accumulate in cells, potentially leading to malfunctions.
In an interesting twist, environmental conditions—such as pH and temperature—can influence the folding of RNase A. This means that the enzyme might adapt its structure under different cellular conditions to maintain optimal performance. Such plasticity is a reflection of evolutionary adaptation, allowing RNase A to thrive across various organisms and biological systems.
Active Site Architecture
The active site of RNase A is a masterpiece of molecular engineering. Located in a cleft formed by the protein structure, it is specifically shaped to accommodate substrate RNA. This pocket not only ensures that the RNA fits snugly but also creates an ideal microenvironment for catalysis.
At the heart of the active site are key residues that facilitate the enzymatic reaction. These residues can act as both proton donors and acceptors, effectively facilitating the chemical reactions necessary for RNA cleavage. The precise arrangement of these amino acids helps stabilize the transition state during the catalytic process, significantly enhancing the efficiency of RNA hydrolysis.
Moreover, the localization of the active site is crucial; it allows RNase A to effectively bind and cleave RNA molecules without detaching from its surrounding structural components, maintaining overall enzymatic integrity.
Role of Specific Amino Acids
A closer inspection reveals that specific amino acids within RNase A are not just passive contributors but are integral to its function. Each residue plays a distinct role; for instance, the amino acid histidine is often cited for its dual capability as a proton donor and acceptor, making it indispensable in the catalytic mechanism. Similarly, other residues such as aspartic acid are involved in stabilizing the positive charges that arise during the cleavage of RNA.
The importance of amino acids extends beyond just catalytic activity. The arrangement and properties of these residues can influence not just the enzyme's reactivity but also its stability. Mutations in these crucial amino acids can lead to conformational changes that may affect binding affinity and kinetic parameters of the enzyme.
Understanding the precise involvement of various amino acids in RNase A is not merely a matter of academic curiosity. It has profound implications, especially in the fields of biotechnology and medicine, where knowledge of how to manipulate these residues can lead to the design of more effective RNase variants for therapeutic uses.
The intricate balance of structure and function in RNase A underscores the elegance of molecular biology, demonstrating how small changes at the atomic level can lead to significant biological outcomes.
Catalytic Mechanisms of RNase A
The catalytic mechanisms of RNase A are central to understanding how this enzyme functions within biological systems. This section will shed light on the intricate processes that enable RNase A to cleave RNA—a crucial undertaking for maintaining the proper RNA profiles within cells. By examining detailed aspects of RNA cleavage, the role of metal ions and cofactors, along with the kinetics of the enzymatic action, we can appreciate why RNase A stands as a vital component in molecular biology.
Mechanism of RNA Cleavage
The primary function of RNase A revolves around the cleavage of RNA molecules. Understanding this mechanism is like peeling an onion—every layer reveals new insights. In essence, RNase A targets the phosphodiester bonds that link RNA nucleotides, breaking these connections to facilitate the degradation or processing of RNA.
The catalytic action begins with the enzyme recognizing its RNA substrate. It's a dance of sorts; RNase A binds to the RNA, positioning the substrate in a manner conducive to reaction. This orientation is essential, as it sets the stage for the subsequent chemical transformation.
Once bound, RNase A activates a water molecule to act as a nucleophile. This water molecule attacks the phosphorous atom in the RNA’s backbone, resulting in the hydrolysis of the phosphodiester bond. To simplify, think of this as a pair of scissors cutting a ribbon. The precision with which RNase A operates ensures that the cleavage process is efficient and specific, minimizing off-target effects and maximally benefiting cellular functions.
Role of Cofactors and Metal Ions
Cofactors often play unsung hero roles in enzymatic processes, and RNase A is no different. Metal ions, such as magnesium and potassium, serve as essential players in stabilizing the structure of the enzyme and facilitating the catalytic process. In RNase A, magnesium ions bind at the active site, where they help in orienting the substrate and stabilizing negative charges that form during the cleavage reaction.
- Magnesium ions enhance the overall activity by helping to coordinate the charging of the water molecule, ensuring that it is primed for nucleophilic attack.
- Potassium ions support the proper folding of RNase A, which in turn is crucial for maintaining the structural integrity of the active site.
In summary, without these metal cofactors, RNase A would struggle to perform its duty. They act as crucial facilitators, ensuring that the enzyme can effectively and efficiently cleave RNA.
Kinetics of RNase A Catalysis
When assessing any enzymatic process, the kinetics reveal essential insights into the efficiency and speed of each reaction. For RNase A, its kinetic parameters tell a compelling story of adaptability and performance efficiency.
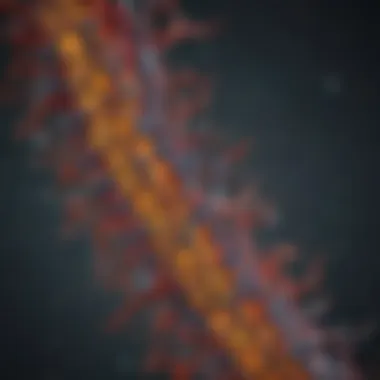
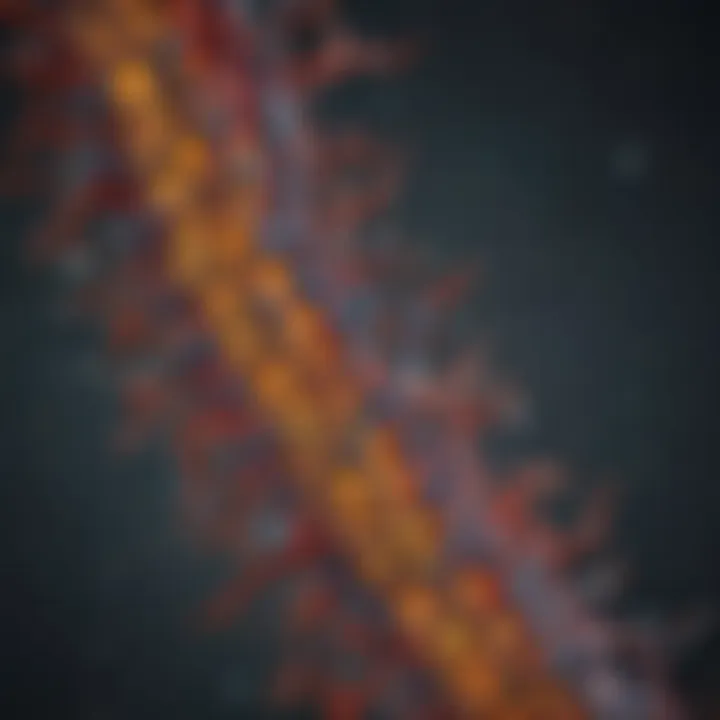
The study of RNase A kinetics can involve a number of parameters including:
- Enzyme kinetics: The speed at which RNase A catalyzes RNA cleavage can be measured using Michaelis-Menten kinetics, providing clear insight into its catalytic properties.
- Turnover number (kcat): This metric indicates how many substrate molecules an enzyme can convert into product per unit of time when the enzyme is fully saturated with substrate.
- Michaelis constant (Km): This value reflects the substrate concentration at which the reaction rate is at half its maximum. Lower Km values indicate higher affinity of the enzyme for its substrate.
Understanding these kinetic factors helps in appreciating how RNase A can adapt to varying cellular environments and sustain its functions under different conditions. By leveraging both its chemical makeup and its kinetic capabilities, RNase A ensures that RNA metabolism is finely tuned to the needs of cellular processes.
"The efficiency and precision of RNase A highlight its pivotal role in biological systems, demonstrating how a small enzyme can wield significant influence over RNA dynamics."
Functional Roles of RNase A
Understanding the functional roles of RNase A is crucial to appreciate its significance in various biological processes. This enzyme is not merely a molecular player; it wields considerable influence over RNA dynamics, immune responses, and interactions with pathogens. By examining these dimensions, one can see why RNase A is pivotal in research and therapeutic settings.
Role in RNA Metabolism
RNase A plays a vital part in RNA metabolism, typified by its involvement in RNA turnover and processing. It facilitates the degradation of RNA molecules, ensuring that cellular RNA levels are tightly regulated. This process is particularly important when considering how cells manage abnormal RNA transcripts, which can arise from faulty cellular functions or viral infections.
In a typical scenario, after transcription, various RNA products may need to be discarded if they are no longer needed. RNase A steps in to hydrolyze RNA, clearing the deck so that fresh transcripts can be synthesized when necessary. By doing so, it not only maintains the RNA pool but also helps unwind any tangled RNA networks, thereby upholding cellular health.
In essence, whether it’s the removal of faulty mRNA or the recycling of RNA components for new synthesis, RNase A contributes significantly to RNA metabolism, thereby supporting the overall functionality of the cell. It’s like a meticulous librarian who keeps the library organized and the books fresh.
Involvement in Cellular Defense Mechanisms
Another fascinating component of RNase A’s functionality is its role in cellular defense mechanisms. This enzyme acts as a frontline defense against RNA viruses and other pathogens. When viral RNA enters a host cell, RNase A can recognize and cleave this RNA, effectively neutralizing the threat and preventing viral replication.
This defensive action is a form of innate immunity, providing a rapid response mechanism that can act before more specialized immune responses are triggered. RNase A’s antimicrobial properties have garnered attention in research, linking it to potential therapeutic strategies involving antiviral treatments. By harnessing this natural mechanism, scientists hope to develop new drugs that can exploit RNase A’s RNA-cleaving capabilities against various viral pathogens.
A notable point is that these defensive functionalities are often enhanced through various cofactors, creating a collaborative network where RNase A operates alongside other immune components to maximize efficacy.
Impacts on Viral Replication
The interplay between RNase A and viral replication illustrates the enzyme’s importance beyond mere RNA degradation. When a virus infects a host, it hijacks the cellular machinery to replicate its own RNA. However, RNase A's RNA-cleaving action can disrupt this process, serving as a barrier to viral propagation.
In particular, RNase A has been investigated in the context of various viruses, showing promise in reducing viral loads in laboratory settings. For example, studies have shown that RNase A can inhibit the replication of certain types of RNA viruses by degrading their RNA, thus stunting their ability to reproduce within the host.
It's like a vigilant security guard, ensuring that no unauthorized copies are made in the vicinity. This characteristic of RNase A can potentially lead to innovative therapeutic strategies for treating RNA virus infections, positioning it as a compelling subject of study in virology and immunology.
"RNase A's multifaceted roles reflect its evolution as not just an enzyme but a guardian of cellular integrity and a key player in the complex interplay of biological systems."
In summary, the functions of RNase A extend well beyond simple RNA turnover. Its active participation in RNA metabolism, coupled with its defensive mechanisms against pathogens and roles in curtailing viral replication, underscore its significance in molecular biology. These insights pave the way for further investigation, highlighting RNase A not just as a topic of academic interest but as a potential avenue for practical applications in research and medicine.
Evolution of RNase A
The evolution of RNase A reflects not only the remarkable adaptability of this enzyme but also its critical role in various biological processes. Understanding how RNase A has evolved through time highlights its resilience and utility across different organisms. This historical timeline uncovers the pathways through which RNase A has optimized its structure and function, further solidifying its place in molecular biology.
Phylogenetic Analysis
Phylogenetic analysis serves as a powerful tool to trace the lineage of RNase A among different species. By examining the sequence variations, researchers can create a map of RNase A evolutionary history. This process is like piecing together a puzzle in which every sequence corresponds to a unique trait shaped by environmental pressures. One key observation from phylogenetic studies is the conservation of certain amino acids that are vital for RNase A's function.
"Studying the evolution of RNase A not only informs us about the past but also offers insights into its current roles and potential future adaptations."
Here are some insights from phylogenetic analysis:
- Conserved Regions: Specific sequences, often found in the active site, demonstrate significant conservation across species. These regions are crucial for maintaining the enzymatic activity of RNase A.
- Divergence Events: The timeline uncovers critical divergence points where RNase A variants adapted to new cellular environments or functions.
- Comparative Genomics: The comparison of RNase A homologs from various organisms can hint at evolutionary pressures, such as climate change or disease resistance, influencing its adaptation over time.
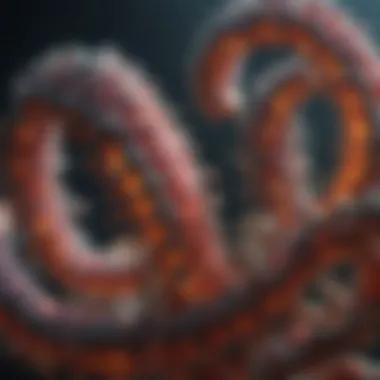
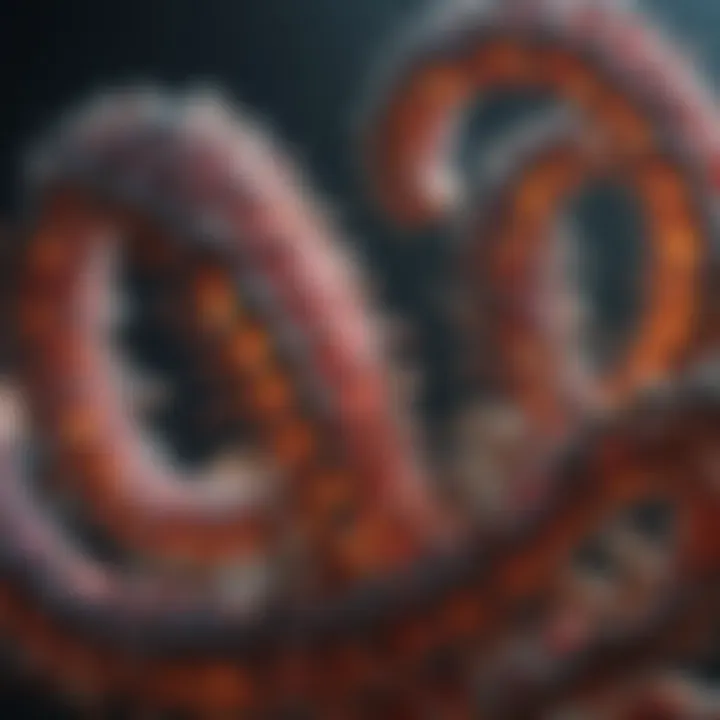
In sum, phylogenetic analysis of RNase A reveals a story of survival and adaptation, showcasing how this enzyme has distinctly evolved in different life forms to play a biochemical role that is integral to life.
Adaptive Evolution of RNase Variants
Adaptive evolution highlights the dynamic nature of RNase A, showcasing varying forms and functions of its variants across species. This phenomenon is fueled by selective pressures in differing environments.
One notable example comes from the RNase A variants found in plants and animals. Each has adapted to meet specific needs based on their ecological niches. For instance, plants often face unique challenges such as pathogen invasion, leading to the evolution of RNase variants that can target viral RNA effectively. This reflects a classic evolutionary adage: "Survival of the fittest."
Key elements of the adaptive evolution of RNase variants include:
- Functional Specialization: Variants may have evolved to optimize specific functions, such as defense against pathogens in certain plants versus digestive processes in mammals.
- Environmental Pressures: Changes in habitat or the introduction of new stresses can drive adaptive modifications to RNase A, allowing organisms to thrive.
- Post-Translational Modifications: These can alter enzyme activity, affecting how RNase A interacts with RNA substrates. This flexibility often contributes to evolutionary advantages.
One cannot overlook the significance of these adaptive changes. As organisms encounter new challenges or opportunities, RNase A variants illustrate evolutionary innovation, ensuring that these enzymes remain effective, functional, and relevant in ever-changing biological landscapes.
By exploring the evolution of RNase A, we uncover a vibrant tapestry woven through time, illustrating the intricate relationship between structure, function, and survival.
Applications of RNase A in Research and Medicine
The significance of RNase A within the fields of research and medicine cannot be overstated. This enzyme is not merely a tool for biochemists; it is an integral player in various biological processes and has substantial implications for therapeutic developments. Throughout this section, we will delve into the myriad of ways RNase A is employed, emphasizing its role in molecular cloning and its therapeutic potential.
Roles in Molecular Cloning
Molecular cloning is a fundamental technique in genetics and biotechnology, paving the way for advancements in genetic engineering and synthetic biology. RNase A plays a pivotal role in several stages of cloning processes, which include:
- RNA Analysis: RNase A is utilized to digest RNA contaminants from DNA preparations, thus ensuring cleaner samples for downstream applications. This is crucial when researchers need to amplify or analyze DNA without interference from RNA.
- Plasmid Preparation: The enzyme's ability to selectively degrade RNA makes it essential when isolating plasmids for cloning. In cloning experiments, purified DNA is often mixed with RNase A to remove any residual RNA, leading to higher-quality constructs for transformation.
- RT-PCR Applications: In reverse transcription PCR (RT-PCR), fresh RNA templates are often used to study gene expression. RNase A facilitates this process by eliminating unwanted RNA, ensuring that only the relevant transcripts are amplified and analyzed.
The reliability and efficiency of RNase A in these applications highlight its value in molecular cloning, enabling researchers to achieve more accurate and reproducible results. This is vital in contexts where precision is paramount, such as genetic modifications and developing recombinant proteins.
Therapeutic Applications and Future Prospects
As the medical field continues to evolve, the therapeutic applications of RNase A are emerging as a promising area of exploration. Key therapeutic potentials of RNase A include:
- Antiviral Properties: RNase A has been investigated for its ability to degrade viral RNA, showcasing a potential role in developing antiviral strategies. Some studies suggest that it can be effective against certain viruses by inhibiting viral replication, thereby reducing the infection's severity.
- Cancer Research: Research into the mechanisms of RNase A has unveiled its interesting role in apoptosis. Some cancer treatments are looking into RNase A as an adjunct therapy that can sensitize tumor cells to chemotherapeutic agents, making them more vulnerable to treatment.
- Drug Delivery Systems: Innovatively, RNase A is being explored for utilization in drug delivery systems. Encapsulating RNase A within nanoparticles may enhance the selective degradation of RNA in targeted cells, which could improve the efficacy of RNA-based therapeutics.
Despite the vast potential, certain challenges remain. The specificity of RNase A's activity, for instance, can lead to unintended consequences, such as triggering immune responses when used in therapeutic contexts. Therefore, ongoing research must focus on optimizing delivery methods and ensuring the safety of RNase-based therapies.
"Through continued innovation and exploration, RNase A stands to bridge the gap between molecular biology and clinical medicine, offering novel solutions to long-standing problems."
With a robust foundation in molecular cloning already established, RNase A’s future in therapeutic applications holds great promise. As understanding deepens regarding its mechanisms and interactions, further advancements in research and clinical applications are anticipated, pushing the boundaries of biomedical science.
Closure and Future Directions
The exploration of RNase A mechanisms propels a profound understanding of molecular biology, offering a window to its structural intricacies and operational dynamics. As this enzyme plays a pivotal role in RNA metabolism and cellular defenses, the insights gleaned from its study extend beyond mere academic interest. The implications stretch into therapeutic applications and biotechnological innovations, providing fertile ground for future research. The continuous evolution of this field implies we stand on the brink of potential breakthroughs that could redefine our approach to traditional and emergent diseases.
Summary of Key Insights
Throughout this article, several critical elements about RNase A have been emphasized:
- Key Functions: RNase A is a crucial player in RNA metabolism, necessary for cellular homeostasis and defense mechanisms against viral infiltration.
- Structural Features: The enzyme's three-dimensional architecture is intimately linked to its activity, where active site architecture and specific amino acids align perfectly to facilitate catalysis.
- Catalytic Mechanisms: Understanding RNA cleavage methods not only augments our knowledge of enzyme kinetics but also affirms the broader impact of enzyme-substrate interactions across various biological contexts.
This synthesis of insights reveals that RNase A is more than an enzyme; it is a fundamental component that interlaces various biological pathways, ushering a deeper appreciations of cellular functionality.
Future Research Directions
Looking ahead, there are several promising avenues for future exploration regarding RNase A:
- Variant Studies: Investigating the adaptive evolution of RNase A variants across different organisms could unveil novel catalytic properties that might be harnessed in genomic technologies.
- Structural Dynamics: High-resolution structural studies, particularly using nuclear magnetic resonance spectroscopy or cryo-electron microscopy, could shed light on dynamic changes during catalysis or substrate binding.
- Therapeutic Innovations: Pioneering research in targeted RNase delivery systems might reconfigure strategies in RNA-targeted therapies, particularly for viral infections.
- Bioinformatics Approaches: Integrating computational and bioinformatics tools can refine our understanding of RNase A pathways, identifying potential regulatory mechanisms that have yet to be unraveled.
The importance of this journey into RNase A's terrain cannot be understated. As new technologies and methodologies continue to emerge, so too does the potential for discovering life-altering applications for this vital enzyme in health and disease management.