Tunnel Assays: Principles, Applications, and Advancements
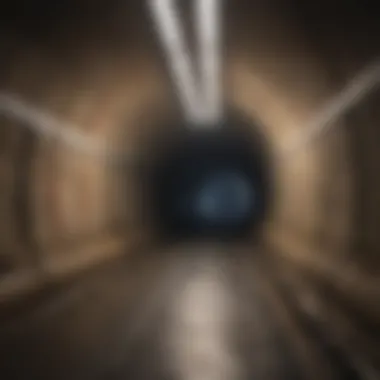
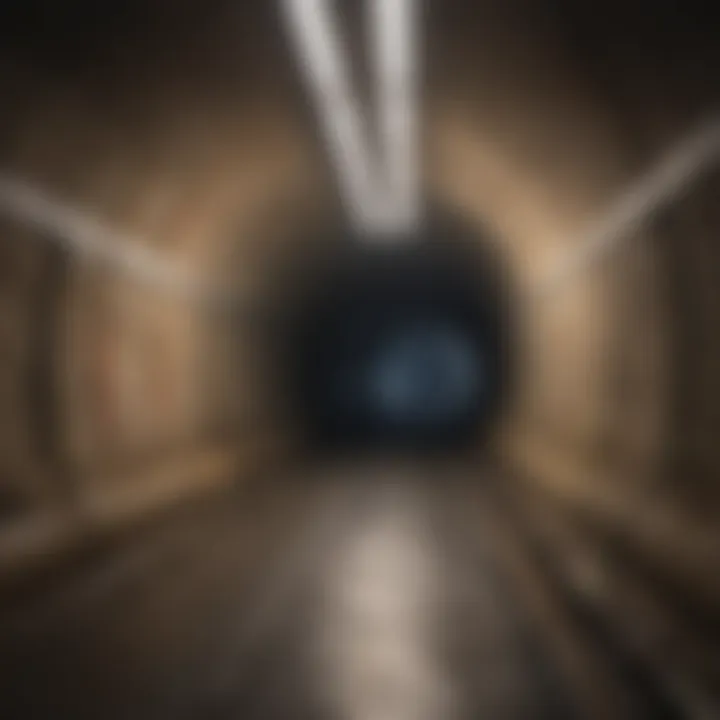
Intro
Tunnel assays represent a pivotal technique in the realm of scientific research, bridging gaps between theoretical exploration and empirical validation. They offer a structured approach to assess a variety of biological and chemical phenomena, fostering a deeper understanding in diverse disciplines from biochemistry to materials science. As researchers dig into these assays, they not only uncover the mechanisms at play but also lay the foundation for applicable innovations.
This comprehensive discourse aims to dissect the foundational principles, showcasing how tunnel assays function, their applications across various fields, and the strides made in methodology that have propelled their relevance. With an intent to empower students, researchers, educators, and professionals, the subsequent sections will illuminate the intricacies of these assays, emphasizing their essential role in advancing scientific knowledge.
Research Overview
Summary of key findings
Through meticulous analysis, studies reveal that tunnel assays are highly effective in quantifying molecular interactions and reactions. Key findings indicate that these assays can discern subtle differences in behavior of compounds, allowing scientists to make pointed conclusions based on recorded data.
In recent years, enhancements in precision and sensitivity have marked a significant evolution in their effectiveness. Now, researchers can monitor real-time changes in molecular activity, shedding light on processes that were previously elusive. This robust dataset underscores the versatility of tunnel assays across disciplines, supporting investigations whether in pharmacology, toxicology, or engineering.
Importance of the research in its respective field
The implications of tunnel assays stretch far beyond academic curiosity. In clinical settings, these assays facilitate drug development by enabling high-throughput screening of potential candidates. The capacity to accurately predict interactions at the molecular level can significantly reduce the time and cost associated with developing new therapies. Similarly, environmental applications see tunnel assays being employed to assess contamination levels, fostering a proactive approach in addressing ecological challenges.
As such, the ongoing development and refinement of tunnel assays is crucial, not only for scientific inquiry but for real-world applications that impact health, safety, and the environment.
Methodology
Description of the experimental or analytical methods used
To effectively harness the power of tunnel assays, researchers typically employ a range of analytical techniques. Techniques such as fluorescence resonance energy transfer (FRET) and surface plasmon resonance (SPR) are commonly utilized to analyze interactions in real time, providing vital insights into molecular dynamics.
Collaborative experiments often integrate computational models to complement the empirical findings. This synergistic approach allows for more robust data interpretation and predictive modeling, ultimately enhancing the overall research quality.
Sampling criteria and data collection techniques
Proper sampling is fundamental in tunnel assays. Criteria may vary based on the specific goals of the study, but often, researchers prioritize high-quality, well-characterized samples. Data collection usually involves meticulous tracking of baselines and real-time measurements using advanced instruments capable of delivering high sensitivity.
In some cases, researchers engage in multiple rounds of testing to validate initial results, ensuring that the findings are both reproducible and reflective of the underlying phenomena at play. Ultimately, it is this commitment to rigor that lends credibility to research utilizing tunnel assays.
Understanding Tunnel Assays
The concept of tunnel assays occupies a critical space in the realm of scientific investigation. When one digs deeper, it becomes clear that these assays are not just a footnote in experimental methodology but a comprehensive approach to understanding interactions that might otherwise go unnoticed. This section dissects the importance of grasping tunnel assays, laying the groundwork for what follows.
With their ability to assess various biological and chemical phenomena, tunnel assays have emerged as crucial tools. They enable researchers to detect subtle changes and interactions across multiple disciplines, from ecology to pharmacology. Perhaps one of the biggest benefits lies in their flexibility; these assays can adapt to the demands of different experiments while maintaining their robust reliability. Thus, becoming familiar with the mechanics of tunnel assays is essential for any scientist who aims to innovate or expand on previous work.
Definition and Overview
At its core, a tunnel assay is a method designed to evaluate interactions within complex systems, often at a microscopic level. Typically, this process involves creating a controlled environment where variables can be isolated and tested. For instance, in a biological context, a tunnel assay might focus on how a particular drug interacts with a specific enzyme, allowing scientists to observe outcomes without external interference.
A key feature of tunnel assays is their confinement of variables, making it easier to draw accurate conclusions about specific interactions. Researchers often employ various techniques such as fluorescence microscopy or electrical measurements to gain insights into interactions previously hidden from view.
Historical Context
The development of tunnel assays is woven into the fabric of scientific progress, reflecting innovations in both technology and methodology. In the early days, the rudimentary versions of these assays were quite limited, primarily used for basic chemical reactions. However, as understanding expanded and technology evolved, so did the complexity and applications of tunnel assays.
For example, consider the transition from simple glass tubes in labs to sophisticated microfluidic devices. These advancements not only enhanced the precision of the assays but also allowed researchers to explore interactions at the cellular level. This progression underscores an important point: understanding tunnel assays directly correlates to appreciating the history of scientific methodology itself.
"An awareness of the past is pivotal for paving the path to future discoveries."
As we explore further into the intricacies of tunnel assays, it becomes apparent that a thoughtful approach to this topic will enrich our understanding of its current applications and potential future directions. Familiarizing oneself with both the modern techniques and the historical backdrop of tunnel assays sets the stage for a deeper investigation into their principles and practices.
Key Principles of Tunnel Assays
Tunnel assays are instrumental in understanding the interactions and behaviors of various substances, whether they be biological, chemical, or physical. These principles form the backbone of how these assays function and why they are widely used in scientific research. By grasping the key principles of tunnel assays, one can appreciate their diverse applications and the innovations they bring to the scientific table.
Theoretical Framework
To appreciate tunnel assays, it's crucial to understand their underlying theoretical framework. At its core, a tunnel assay leverages the phenomenon of permeability, which is the ability of substances to pass through a medium. This concept plays a vital role in the design and implementation of these assays. The model often used is akin to the way water flows through soil: different substances, like compounds or cells, will navigate through a matrix based on their unique properties, including size, charge, and solubility.
The theoretical framework incorporates various aspects of chemistry and biology, such as diffusion and binding kinetics, emphasizing the relationship between concentration gradients and molecular interactions.
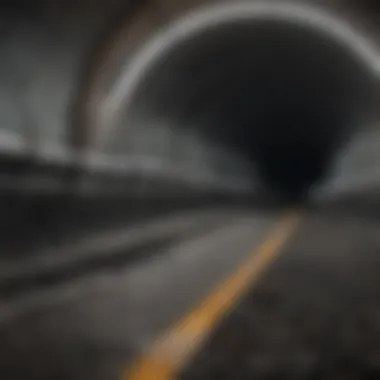
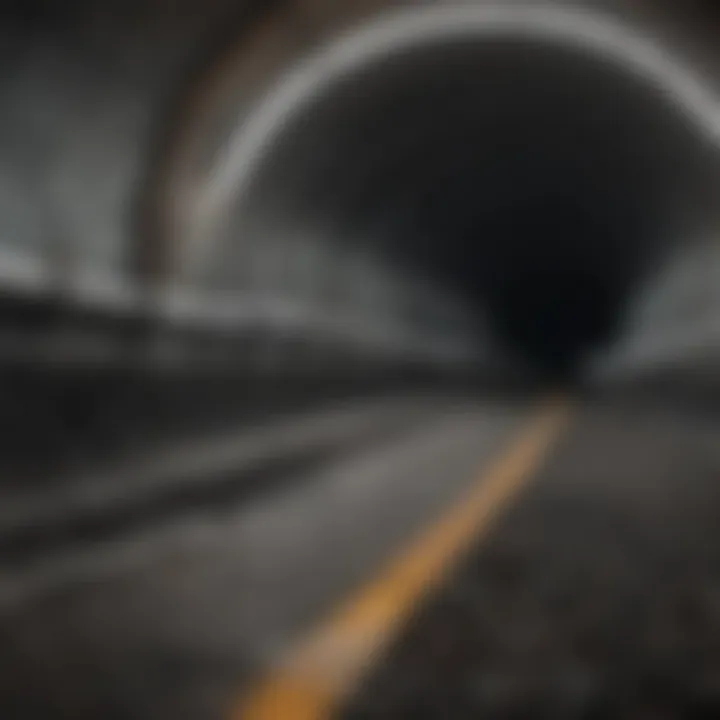
In practical terms, this means that researchers can predict how efficiently a given substance will move through a defined tunnel when affected by external variables like temperature and pH. Knowing this helps in fine-tuning experiments to qauantify results more accurately.
Mechanisms of Action
When examining the mechanisms of action in tunnel assays, one can think of it like a dance — different molecules have distinct ways of moving through a tunnel, influenced by their characteristics and the environment they find themselves in. Several important mechanisms are at play during a tunnel assay, including diffusion, convection, and advection.
- Diffusion: This is the primary mechanism where substances migrate from areas of high concentration to areas of low concentration. Thus, how quickly and efficiently a compound travels through the tunnel greatly impacts the assay’s outcome.
- Convection: Here, bulk movement of fluid carries substances along. This can happen in larger tunnel systems, especially in rapid experiments designed to observe real-time reactions.
- Advection: This involves the transport of one substance by the flow of another. In the context of a tunnel assay, an example would be a flowing liquid carrying solute particles through a tunnel as the liquid itself moves.
Understanding these mechanisms allows researchers to tailor their experiments more effectively. For instance, if a researcher needs to enhance the sensitivity of their assay, leveraging convection could lead to a faster response time, thereby improving the results.
As the emphasis on specificity and sensitivity continues to grow in scientific research, especially within the realms of drug discovery and environmental monitoring, comprehending and applying the principles of tunnel assays becomes ever more valuable. This pursuit not only aids in achieving precision but also opens up further avenues for innovation in the design of future experimental setups.
"The ability to control and predict the movement of compounds through a tunnel assay is what separates good research from groundbreaking discoveries."
Types of Tunnel Assays
Tunnel assays are not just a one-trick pony; they have a diverse range of types, each serving specific research purposes. Their significance lies in how they unfold various phenomena in biology, chemistry, and physical sciences. Understanding the nuances of these different assay types is crucial as they provide distinct insights, methodologies, and applications. The choice of assay often hinges on the nature of the experiment, the type of data needed, and the overall objectives of the research. Below, we delve into three primary categories destined to sprinkle light on biological, chemical, and physical tunnel assays, each with its own identity and utility.
Biological Tunnel Assays
Cell-based assays
Cell-based assays take center stage when it comes to the exploration of cellular responses, drug evaluations, and toxicological testing. Their key characteristic rests in utilizing living cells as the primary experimental unit, which mirrors in vivo conditions more closely than other methods might. This makes them a popular choice in recent times, especially for researchers focusing on personalized medicine and treatment efficacy.
One unique feature of cell-based assays is their ability to measure cellular activity in response to treatments, thus allowing for a more profound understanding of cellular mechanisms at play. However, they come with their share of complications. Issues with reproducibility, variability among cell lines, and the complexity of cellular interactions can present challenges in data interpretation. Still, when these hurdles are managed effectively, the insights gathered can be remarkably valuable in drug development and safety evaluations.
Organismal assays
Shifting the focus, organismal assays extend the observation from cells to entire organisms, often microorganisms or even small model organisms such as Drosophila or Caenorhabditis elegans. The essential aspect of organismal assays lies in their ability to evaluate systemic effects of interventions, whether they are drugs, environmental changes, or genetic modifications. Such studies offer a comprehensive perspective into how treatments manifest across different biological systems.
The beauty of organismal assays is their ability to accommodate more complex interactions that rely on the biology of the whole organism. This holistic approach adds a nuanced layer of data that cell-based assays sometimes lack. However, they do require more sophisticated setups and careful considerations regarding ethical implications and organismal care, which can prove resource-intensive at times.
Chemical Tunnel Assays
Kinetics of reactions
Diving into the realm of chemistry, the kinetics of reactions come to the forefront as a fascinating aspect of tunnel assays. The kinetic assessments allow researchers to dissect the rates of chemical reactions, giving insight into the mechanism and efficiency of these processes. This has notable implications in fields such as catalysis, drug formulation, and biochemical pathway elucidation.
A standout feature of kinetic assays is their precision in measuring reaction rates under various conditions. They can pinpoint the critical factors influencing reaction dynamics, enabling scientists to optimize conditions for desired outcomes. However, the downside is sometimes the need for elaborate instrumentation and expertise. Any slight deviation in experimental conditions can lead to variations in the kinetic data, making it essential to maintain stringent controls and validation throughout the experimental design.
Interaction studies
Next in line are interaction studies, which focus on the relationships between different chemical species, particularly how they interact in solution or solid-state. This area has garnered immense interest as it lays the groundwork for understanding complex networks of biochemical reactions. In drug discovery, for example, exploring these interactions can unearth crucial details about drug-target binding capabilities.
One of the primary advantages of interaction studies is their ability to provide real-time insights into how chemicals engage with one another. Techniques such as surface plasmon resonance and isothermal titration calorimetry epitomize this. Nevertheless, challenges can arise surrounding the interpretation of results, especially when unwanted reactions can obscure the desired signals. Careful experimental design and robust controls are essential to navigate these complexities and yield meaningful data.
Physical Tunnel Assays
Material properties
In the arena of material science, tunnel assays assessing material properties shine a light on the structural characteristics that define material behavior. This is vital for applications in engineering, electronics, and nanotechnology. Inspecting things like tensile strength, ductility, and thermal stability can unveil how materials respond under various conditions, thus guiding their appropriate applications.
A distinctive feature of these assays is their emphasis on quantifiable metrics that can be correlated with material performance. Utilizing methods such as scanning electron microscopy can uncover microstructural information that is crucial for understanding material behavior. On the flip side, one must consider that this analysis often demands advanced equipment which can be costly and may also necessitate a high level of technical skill to interpret the results properly.
Surface interactions
Lastly, surface interactions are pivotal in unraveling phenomena at the interface of materials and their environment. These assays investigate how materials interact with various substances at their surface, pivotal for coating technologies, adhesion science, and biosensor development. The key characteristic here centers around the complex phenomena that occur at that tiny boundary layer, where a lot of action is taking place.
What makes surface interaction studies beneficial is their applicability in real-world scenarios, showcasing how materials will behave outside of controlled lab environments. However, interpreting data accurately can be quite tricky due to the multifaceted nature of surface interactions. A comprehensive understanding of both physical chemistry and material science is vital to draw reliable conclusions from these assays.
Experimental Design in Tunnel Assays
Experimental design plays a crucial role in the efficacy of tunnel assays. It impacts not only the reliability of results but also the reproducibility of experiments. When constructing an experimental framework, certain fundamental elements must be considered: the selection of materials, development of protocols, and methods of data collection and analysis. Each of these components intertwines to create a robust structure that can yield meaningful insights in biological and chemical research.
Selection of Materials and Methods
Choosing the right materials and methods is the bedrock of any experimental design. It’s akin to laying a solid foundation before erecting a skyscraper. The specifics of the materials need to align with the objectives of the study. For instance, when performing a biological tunnel assay, one might select specific cell types that best represent the biological phenomenon under investigation. The method of delivery for the assay components must also be tactically considered.
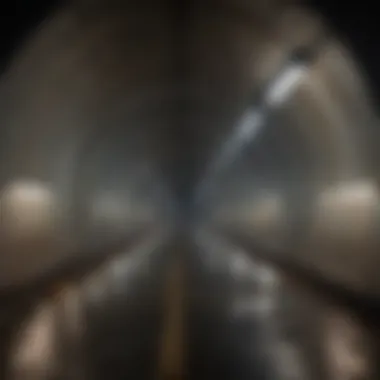
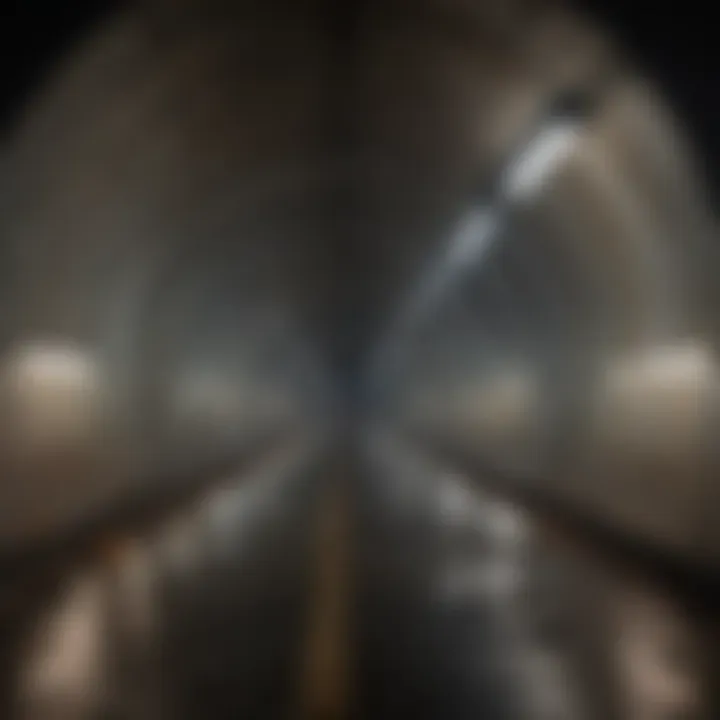
Key Considerations in Material Selection:
- Compatibility: Ensure the materials will interact correctly within the assay framework.
- Quality: The integrity and reliability of reagents must be above board, avoiding low-quality items that can distort results.
- Availability: Sometimes, even the best materials are not accessible. This can limit whether one can conduct a high-quality study.
A particularly interesting example can be found within chemical tunnel assays, where the properties of the chemicals—such as solubility and reactivity—dictate the experimental design. In these situations, methods often involve choosing between standard laboratory reagents or more specialized ones to achieve clearer results.
Protocol Development
Once the materials are decided upon, developing a protocol becomes the next step. This is where creativity meets precision. A well-crafted protocol serves as a roadmap, detailing how to navigate the experimental process. It requires attention to detail, ensuring that every variable is accounted for, thus minimizing unforeseen complications that can arise.
Essential Aspects of Protocol Development:
- Step-by-step Procedures: Clear instructions help avoid ambiguity. Each step should be defined meticulously to facilitate replicability.
- Control Systems: Implementing controls helps in comparison and assessing the validity of results.
- Timing: Not just a formality, the timing of each step can significantly impact the outcome. Certain reactions require precise timing for reliable data collection.
- Safety Precautions: This cannot be overstated. Ensuring safety protocols are in place safeguards all personnel involved in the experiment.
The intricacies involved in protocol development not only ensures a smooth process but also sets the stage for quality outcomes.
Data Collection and Analysis
After executing an experiment following a detailed protocol, the analysis of the collected data reveals whether the hypothesis holds water. Effective data collection and analysis provide insight into the success of the tunnel assay while also reflecting the overall quality of the experimental design.
Approaches to Effective Data Collection and Analysis:
- Standard Operating Procedures: Establishing consistent methods for data collection can enhance credibility.
- Data Management Systems: Utilize software tools that allow for easy sorting, analyzing, and visualization of the data.
- Statistical Methods: Implementing the right statistical analyses is critical for interpreting what the data shows.
- Feedback Loops: After initial interpretations, revisiting the original hypothesis and possibly refining it based on findings is essential for deeper understanding.
"In research, data doesn't just tell a story, it narrates the journey from hypothesis to conclusion."
This phase can be challenging, as it often requires specialized knowledge and tools. However, the richness of insights available through well-analyzed data justifies the effort.
To summarize, experimental design in tunnel assays is like orchestrating a symphony—every note, or in this case, every aspect must be meticulously planned and executed to achieve a harmonious outcome. By carefully selecting materials and methods, developing structured protocols, and rigorously analyzing data, researchers can uncover fundamental truths in science that drive progress across disciplines.
Applications of Tunnel Assays
Tunnel assays have transformed the landscape of scientific research and practical applications across multiple fields. Their unique ability to assess interactions and reactions in a controlled environment makes them invaluable tools for scientists and professionals. Understanding these applications is crucial, not only for advancing knowledge but also for driving innovations in areas where precision and sensitivity are paramount. By comprehensively exploring each application, we can appreciate the advantages and challenges presented by tunnel assays in distinct domains.
In Drug Discovery
Drug discovery is a complex, multi-phase process that demands rigorous testing and validation of potential therapeutic agents. Tunnel assays offer a methodologically robust framework for evaluating drug interactions at various levels, from individual cellular responses to systemic effects. The sensitivity of these assays allows researchers to detect subtle changes in biological systems, which is vital for identifying promising drug candidates.
For instance, cell-based tunnel assays can be designed to monitor how potential drugs influence specific cellular pathways. This method not only improves the rate of discovery but also minimizes the risk of late-stage failures in clinical trials. Furthermore, such assays can provide crucial insights into the pharmacokinetic profile of new compounds, informing dosage regimens and therapeutic effectiveness.
On another note, the versatility of tunnel assays extends to high-throughput screening processes, enabling researchers to evaluate thousands of compounds in a fraction of the time. This can significantly cut down the time and costs associated with drug development, optimizing resource utilization, and bolstering innovation.
In Environmental Monitoring
With environmental issues becoming increasingly pressing, tunnel assays have emerged as vital tools in monitoring pollutants and assessing ecosystem health. These assays can be adeptly employed to evaluate the bioavailability and toxicity of different contaminants. For example, they can pinpoint how various pollutants react with living organisms or ecosystems over time, helping to track their behavior in the environment.
A notable application is in assessing water quality. Tunnel assays can measure concentrations of pollutants like heavy metals or organic compounds, offering scientists reliable data while bypassing some traditional limitations. Additionally, through organismal tunnel assays, researchers can gauge the impact of environmental stressors on specific species, which can lead to informed policymaking and conservation strategies.
The insightful data derived from these assessments can also inform regulatory approaches, ensuring that environmental safety standards are met. Given the global emphasis on sustainability and ecological balance, the role of tunnel assays in environmental monitoring is more critical than ever.
In Material Science
In the domain of material science, tunnel assays have become an integral part of characterizing and evaluating new materials, particularly how they interact at the micro and nanoscale. These assays can provide insights into properties such as porosity, surface interactions, and mechanical strength, which are crucial for developing advanced materials used in technology and construction.
For example, employing physical tunnel assays can help material scientists analyze how different surface treatments influence adhesive properties or corrosion resistance. This ability to observe material behavior under specific conditions fosters innovation in designing more durable and effective products.
Moreover, tunnel assays contribute significantly to polymer science by facilitating the study of kinetic interactions and reaction dynamics, aiding in the development of new synthetic methodologies. These insights ultimately lead to enhanced application potential for materials in various industries, like renewable energy, where efficiency and efficacy are paramount.
"By bridging the gap between fundamental research and practical application, tunnel assays play an indispensable role in accelerating advancements across many fields."
Advantages of Tunnel Assays
Tunnel assays offer significant benefits in various research and application settings, making them essential tools in contemporary science. Their unique methodological advantages allow scientists to probe complex biological and chemical processes effectively, leading to advancements in understanding and application.
High Sensitivity and Specificity
One of the standout features of tunnel assays is their exceptional sensitivity. They can detect minute amounts of substances, even at trace levels, which is crucial in areas like drug discovery and environmental monitoring. This high sensitivity stems from the precise control that tunnel assays exert over experimental conditions, enabling even subtle changes to be captured. For instance, in monitoring environmental pollutants, these assays can identify contaminants at concentrations that would be easily overlooked in less precise methods.
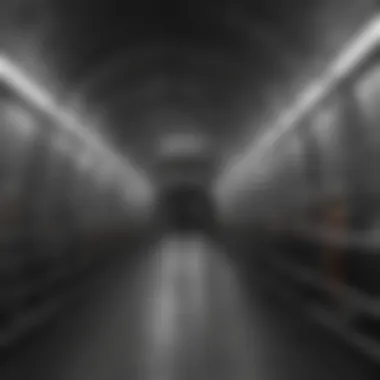
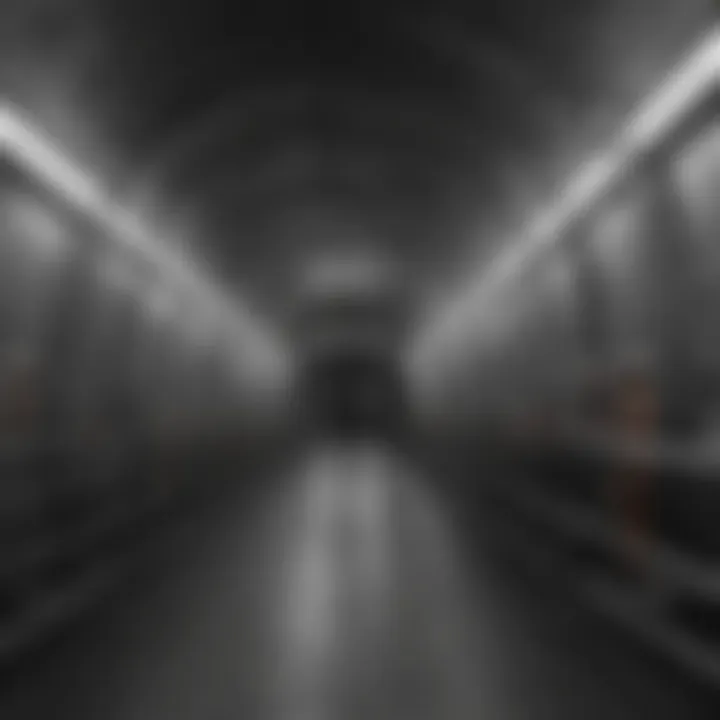
Moreover, the specificity of tunnel assays allows for accurate differentiation between similar molecules. This quality is pivotal when it comes to biological assays, where you may be interested in detecting specific enzyme activities among a myriad of others. It’s like finding a needle in a haystack, but with tunnel assays, the needle shines bright. The combination of high sensitivity and specificity creates a powerful advantage, making these assays reliable for research that demands precision.
Versatility across Disciplines
Tunnel assays are not confined to any single field; they find applications across a broad spectrum of scientific disciplines. From pharmacology to material science, their flexibility makes them a go-to choice for researchers tackling diverse scientific questions. In biological contexts, they can be employed for cell-based assays, where they help in understanding cellular responses to various stimuli. For instance, studying how cancer cells react to potential new drugs provides crucial insights into treatment efficacy.
On the chemical side, tunnel assays may be used to investigate reaction kinetics and mechanisms. This versatility is further exemplified in material science, where they help explore properties of new materials or surface interactions relevant to engineering.
"The inherently adaptable nature of tunnel assays facilitates a multidisciplinary approach to research, bridging gaps across fields and promoting collaborative discoveries."
Challenges and Limitations
Tunnel assays have garnered significant attention for their ability to probe complex interactions within biological, chemical, and material systems. However, like any scientific methodology, they are not without their hurdles. Understanding the challenges and limitations associated with tunnel assays is essential for researchers aiming to fully leverage their potential while avoiding pitfalls.
Technical Difficulties
One of the foremost challenges in tunnel assays pertains to the technical complexities involved in their design and execution. The precision required to establish accurate measurements can be quite demanding. Factors such as equipment calibration, environmental control, and sample preparation techniques can significantly impact the reproducibility of results. For instance, variability in the viscosity of samples could affect the movement of reactants through the tunnel, leading to inaccurate data. Researchers must exercise great care to maintain consistency in these elements. Without proper attention to such details, results can become skewed, rendering findings questionable or leading to false conclusions.
Moreover, some tunnel assay techniques require specialized knowledge in programming or data analysis, which can present an unnecessary barrier for many researchers. Navigating sophisticated software or adapting intricate experimental setups may not be feasible for all lab environments, especially those with limited resources.
Interpretation of Results
Interpreting the outcomes of tunnel assays adds another layer of complexity. The nature of the data generated is often multifaceted, requiring a nuanced understanding of both the methodologies employed and the underlying biological or chemical principles at play. For example, a researcher unversed in the nuances of ligand-receptor interactions might misinterpret kinetic data from a biological tunnel assay, leading to potentially erroneous scientific claims.
Also, several factors can affect the final results, including background noise, non-specific interactions, and the stability of the assay components over time. This can muddy the waters, making it increasingly difficult to draw clear conclusions. Thus, researchers must remain vigilant against overinterpreting data, which can be a tempting trap for those eager to publish novel findings.
In summary, while tunnel assays hold tremendous potential across various fields, navigating the technical difficulties and interpretative challenges is crucial for those involved in their application. By acknowledging these limitations and adopting a careful approach, researchers can work more effectively within the unique framework that tunnel assays offer.
Future Directions in Tunnel Assays
The trajectory of tunnel assays is promising, driving forward not merely scientific inquiry but also practical applications across varied fields. As this methodology gains traction, understanding the future directions becomes paramount, particularly for researchers and professionals. Key elements include the potential perks, considerations around innovative methods, and the deep integration with modern technologies.
Innovative Techniques
Innovation is the lifeblood of scientific progress, and tunnel assays are no exception. With the advent of microfluidics, the precision in these assays is reaching new heights. By integrating micro-scale systems into tunnel assays, researchers can now conduct experiments with minute volumes of reagents and samples. This refinement not only reduces wastage but also enhances sensitivity and accuracy of the results.
Moreover, the application of affinity-based techniques in tunnel assays is noteworthy. This method leverages the specific interactions between molecules—like antibodies and antigens—to improve the detection limits significantly. Envision a scenario where drug discovery relies on pinpoint accuracy, and these innovative techniques provide just that.
Here are a few more groundbreaking techniques anticipated in the next strides:
- Real-time monitoring: This will facilitate instant feedback on reaction dynamics.
- Combination assays: Merging biological and chemical analysis will provide a holistic view of interactions.
- Artificial Intelligence (AI): Employing algorithms to analyze and predict assay outcomes can streamline the research process immensely.
Integration with Emerging Technologies
The importance of integrating tunnel assays with emerging technologies cannot be overstated. Artificial intelligence stands as a monumental tool that is reshaping how data from tunnel assays are interpreted. Machine learning algorithms can sift through vast datasets faster than the blink of an eye, uncovering patterns and correlations that the human eye may miss. This translates to quicker, more reliable conclusions in research settings.
Another revolutionary technology making waves is CRISPR. As this gene-editing marvel continues to evolve, its synergy with tunnel assays opens up exciting avenues for genetic research and therapeutic developments. Imagine using tunnel assays to assess the efficacy of CRISPR interventions, pushing the envelope on precision medicine.
The interconnections are promising:
- Nanotechnology: Enhancing sensitivity and operational efficiency in assays.
- Biomarkers: Advanced detection methods for identifying specific biological markers linked to diseases.
- Cloud Computing: Facilitating enhanced collaboration and data sharing among researchers worldwide.
"The future of tunnel assays lies in the union of precision techniques and inventive technologies, creating a more interconnected landscape for scientific breakthroughs."
In summary, the future of tunnel assays is not just an evolution of methods but a revolution in capabilities. With innovative techniques and the integration of emerging technologies, tunnel assays are set to elevate research across fields, leading to groundbreaking discoveries and applications.
The End
The discussion surrounding tunnel assays culminates in the recognition of their significance within both the scientific community and various applied fields. As a methodological tool, tunnel assays offer unique insights into complex interactions — whether they be biological, chemical, or material-based. This article has traversed a myriad of topics related to tunnel assays, illuminating not only their foundational principles but also their numerous practical applications.
Summary of Findings
After an extensive analysis, several key points emerge from our exploration of tunnel assays:
- Diverse Applications: Tunnel assays span across drug discovery, environmental monitoring, and material science, demonstrating their versatility.
- High Sensitivity: These assays allow for precise measurements, making them invaluable in experimental contexts.
- Innovation and Evolution: With advancing technology, the mechanisms and techniques involved in tunnel assays continue to evolve, presenting exciting future possibilities.
It’s clear that the advantages offered by tunnel assays — notably their sensitivity, applicability, and robustness — highlight their essential role in furthering research and practical endeavors across various domains.
Implications for Research and Practice
The impact of tunnel assays stretches far beyond their technical applications. For researchers, they are a gateway to deeper understanding of biological phenomena and the chemical mechanisms driving interactions in nature. The implications for practice can be summarized as follows:
- Informed Decision-Making: Data obtained from tunnel assays can significantly influence experimental design, leading to more effective methodologies and outcomes in various fields.
- Interdisciplinary Collaboration: The versatility of tunnel assays encourages collaboration across disciplines. Researchers from biology, chemistry, and materials science can leverage each other’s findings, broadening their scope of inquiry.
- Future Innovations: As technology continues to advance, expectations can be set on what tunnel assays may enable in terms of novel discoveries and innovations — it could potentially alter the landscape of research, leading to new methodologies and breakthroughs.