Exploring Ultrasonic Arrays: Mechanisms and Applications
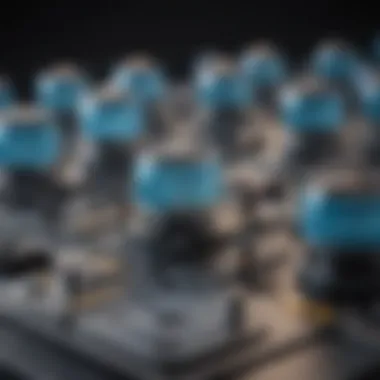
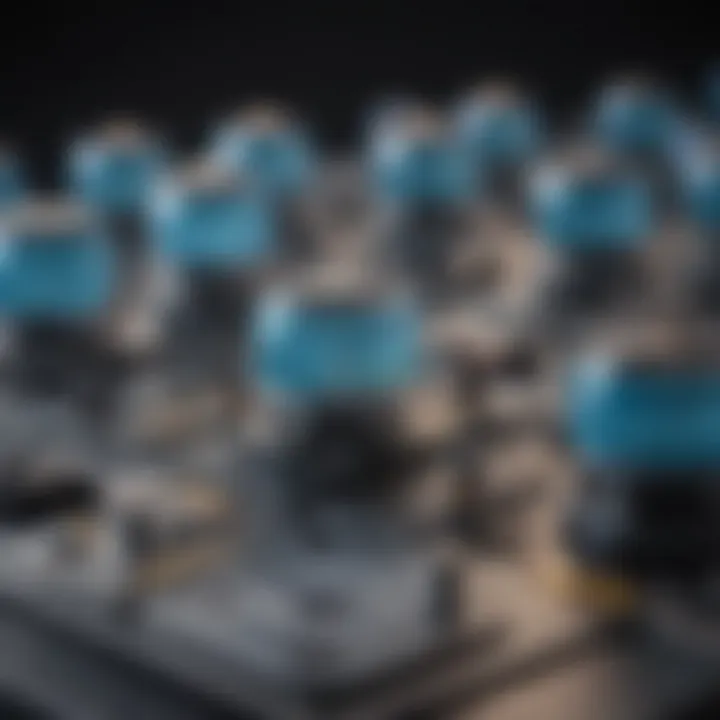
Intro
Ultrasonic arrays have become a pivotal technology with vast applications ranging from medical imaging to industrial inspection. By harnessing the power of sound waves, these arrays can gather information that would otherwise be hidden from the naked eye. In understanding their dynamics, we uncover not only the principles of design and operation but also the stories behind their diverse uses. This exploration reveals how ultrasonic arrays act as both tools and catalysts, enhancing our ability to analyze materials, diagnose medical conditions, and ensure quality in manufacturing processes.
With this backdrop, let's embark on a detailed journey into the intricacies of ultrasonic arrays, examining their foundational concepts and the implications they hold for various fields.
Research Overview
Summary of key findings
In recent studies, ultrasonic arrays have demonstrated remarkable capabilities. The ability to finely tune operational parameters has led to advancements in image resolution in the medical field. For instance, improvements in spatial resolution help clinicians make more accurate diagnoses. In industrial settings, they can detect flaws in materials with exceptional sensitivity, allowing for earlier interventions that can prevent costly failures.
Additionally, the emergence of new materials, like piezoelectric composites, has enhanced energy efficiency, making ultrasonic arrays more effective than ever. Researchers are now looking into machine learning techniques to analyze the data collected by these arrays, potentially unlocking new levels of understanding in both medical and non-medical fields.
Importance of the research in its respective field
The research into ultrasonic arrays is critical for several reasons:
- Medical Imaging: Enhanced resolution directly impacts patient care, enabling quicker and more accurate diagnoses.
- Industrial Inspection: Early detection of material defects can save companies millions and prevent disasters.
- Material Characterization: Understanding material properties on a deeper level opens avenues for innovation and technology advancements.
Methodology
Description of the experimental or analytical methods used
The study of ultrasonic arrays typically employs various experimental techniques. For image acquisition, phased array techniques are commonly utilized, where multiple transducers are strategically arranged to direct ultrasound beams at specific angles. This method enhances the field of view and allows for intricate imaging. The collected data is often processed using algorithms that enhance signal processing, improving the clarity of the images obtained.
In addition to experimental approaches, analytical models play a vital role. These models help in predicting how ultrasonic waves interact with different materials, assisting in fine-tuning the design of the arrays.
Sampling criteria and data collection techniques
Data collection often involves testing different materials using rigorous criteria, depending on the research goals. For medical applications, samples may include biological tissues, while industrial inspections might focus on metals or composites.
The use of both quantitative and qualitative data collection techniques ensures a comprehensive understanding of ultrasonic dynamics. Often, researchers utilize software tools to visualize and analyze the data collected from various arrays.
Through meticulous methodology, the study of ultrasonic arrays stands as a fine example of the intersection between technology and scientific inquiry. By delving deeper into the operation and application of these tools, we unlock exciting prospects for future advancements.
Prolusion to Ultrasonic Arrays
Ultrasonic arrays play a vital role in a variety of modern technologies, particularly across fields like medical imaging and industrial inspections. This system relies on the principles of acoustic waves, displaying an impressive capability to gather data and create images in high resolution. The versatility of these arrays can't be overstated; they are not just limited to one specific application but span a broad spectrum of use cases that range from non-destructive testing of materials to advanced diagnostic imaging systems.
Definition and Composition
To grasp the importance of ultrasonic arrays, one must start with a firm definition. Ultrasonic arrays comprise multiple transducer elements arranged in a specific configuration to transmit and receive ultrasound waves. These elements typically function at frequencies above the audible range, allowing them to capture detailed images and precise measurements.
The composition of these arrays generally involves piezoelectric materials that convert electrical signals into mechanical vibrations. Some common materials used include lead zirconate titanate (PZT) and polyvinylidene fluoride (PVDF). The careful arrangement and spacing of these elements in relation to one another significantly influence the array's performance and capabilities.
Moreover, there are configurations, such as linear and phased arrays, which serve distinct functions based on the application. Each design intricately ties to the intended use; for example, a phased array can electronically steer the ultrasound beam, resulting in the ability to scan complex geometries without physical movement of the device.
Historical Background
The roots of ultrasonic technology dip well into the 20th century. Perhaps most prominently, the introduction of sonar during World War I paved the way for advancements in ultrasonic applications. While sonar primarily focused on military uses, the principles carried over into civilian domains. The incorporation of ultrasonics into medical imaging began to gain traction in the late 1950s and early 1960s with the advent of the first ultrasound machines. These machines utilized single-element transducers which obstinately laid the groundwork for the development of more sophisticated array systems.
By the 1970s, researchers began to recognize the limitations of traditional single-wavelength systems. This led to the growth and refinement of ultrasonic arrays, facilitating greater resolution and wider scanning capabilities. The developments over decades have been staggering. From bulky, expensive machines to the sleek, portable devices we see today, the evolution of ultrasonic arrays reflects technological progress in every sense.
Relevance in Modern Science
In todayās rapidly evolving scientific landscape, the impact of ultrasonic arrays is profound. First and foremost, they have transformed medical imaging. Clinicians rely on high-resolution ultrasound scans for diagnostics, making it an indispensable tool in prenatal appointments, cardiac examinations, and more. The non-invasive nature of these scans allows for safe and effective monitoring of health conditions.
Outside of healthcare, industries utilize ultrasonic arrays for non-destructive testing to ensure material integrity. This significance only grows as industries pivot towards more automated processes. Consider the automotive and aerospace sectors, where a missed flaw can lead to catastrophic failures. Moreover, the rise in interest for smart manufacturing has integrated ultrasonic arrays into quality control workflows that monitor products in real-time.
"Ultrasonic arrays not only improve accuracy in diagnostics but are also central to ensuring safety in manufacturing processes."
In summary, the dynamics and applications of ultrasonic arrays are not just technical curiosities but are pivotal to both scientific advancement and real-world applications. As the technology continues to evolve, both challenges and innovations will shape the future of ultrasonic studies, making it a crucial element for various fields.
Fundamental Principles of Ultrasonics
Understanding the fundamental principles of ultrasonics is crucial in grasping how ultrasonic arrays function effectively across diverse applications. Unlike traditional sound waves, ultrasonic waves operate at frequencies beyond the human audible range, making them valuable for a variety of technical and medical uses. By diving deeper into the specifics of acoustic waves, transducer mechanisms, and wave propagation, we can appreciate the intricacies and benefits that these principles afford.
Acoustic Waves and Their Properties
Acoustic waves are the building blocks of the ultrasonic realm. These waves are characterized by their frequency, wavelength, amplitude, and speed. The frequency dictates how often the wave oscillates per second, which can range from 20 kHz to several hundred megahertz in ultrasound applications. Higher frequencies generally result in better resolution but less penetration in dense materials.
Key Properties of Acoustic Waves:
- Frequency: Determines the clarity and penetration of the wave.
- Wavelength: Influences how the wave interacts with different materials.
- Amplitude: Affects the strength of the wave and, in turn, the intensity of the received signal.
- Speed: Varies based on the medium through which the wave travels.
In practical applications, understanding these properties allows for fine-tuning of ultrasonic systems to achieve desired outcomes, whether in imaging or material analysis. By manipulating frequency and amplitude, technicians can enhance image resolution in medical ultrasound or improve defect detection in industrial testing.
Transducer Mechanisms
Transducers serve as the heart of ultrasonic arrays, converting electrical energy into mechanical vibrations that produce acoustic waves, and vice versa. The most common types of transducers include piezoelectric crystals which expand and contract when an electrical signal is applied, thus generating ultrasound.
Mechanisms of Transducers:
- Piezoelectric Effect: The foundational principle where materials generate an electric charge when mechanically stressed.
- Magnetostrictive Effect: Involves materials that change shape in the presence of a magnetic field, thus producing ultrasonic waves.
- Surface Acoustic Wave (SAW) Devices: These devices use acoustic waves on the surface of materials, allowing for applications in sensors and acoustic signal processing.
Understanding these mechanisms is essential for developments in ultrasonic technology. The precise functioning of these transducers impacts the quality of the signals received and the acoustic images produced, which is especially important in medical imaging, where clarity can be a matter of critical importance.
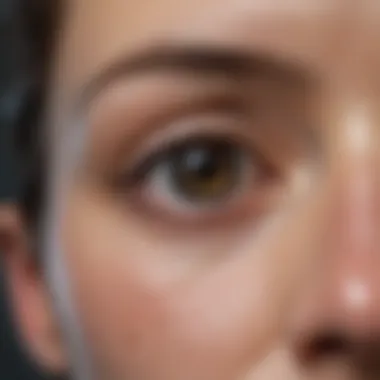
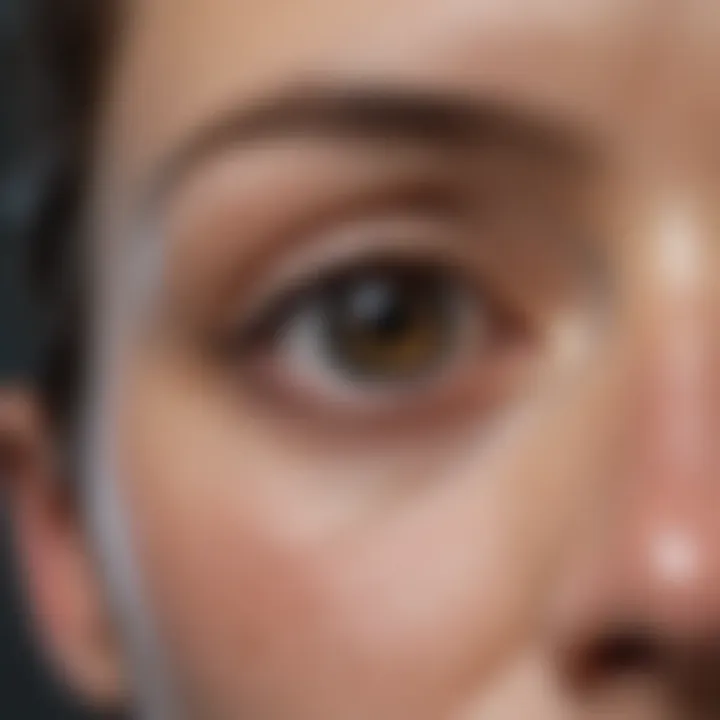
Wave Propagation in Different Media
The propagation of ultrasonic waves through various media is another critical principle that affects their application. Different materials possess distinct acoustic impedances, which influence how ultrasonic waves travel through them. Factors such as density, elasticity, and temperature play significant roles.
- Solid Materials: Ultrasonic waves typically travel faster in solids due to tightly packed molecules that facilitate efficient vibrational transfer.
- Liquids: The speed of sound in liquids is substantially slower than in solids, but ultrasonic applications in medical settings take advantage of this property to generate images of soft tissues.
- Gases: In gases, meanwhile, acoustic waves travel even slower and are less effective for detailed imaging but can serve purposes in detecting leaks or monitoring industrial processes.
Understanding how ultrasonic waves propagateāand how this propagation can be adjusted or manipulatedāis vital for engineers and researchers working to harness these waves in innovative ways. The ability to adapt to the specific challenges posed by different media enhances the effectiveness of ultrasonic applications across industries.
"Knowledge of the fundamental principles of ultrasonics not just enriches technical skills but expands the horizons of innovation in fields that range from healthcare to industrial applications."
These core principles serve as the foundation on which the functionalities of ultrasonic arrays are built. Mastering these elements will pave the way for future developments and improvements within this vibrant field.
Design Aspects of Ultrasonic Arrays
In the realm of ultrasonic technology, the design aspects of ultrasonic arrays hold immense significance. These arrays are pivotal in shaping their functionality and performance in various applications, from medical imaging to industrial testing. Understanding the design nuancesāright from configuration types to element spacing and phased technologyāenables practitioners to optimize the effectiveness of ultrasonic arrays for specific purposes. This section delves deep into the components that influence the efficacy of these sophisticated systems.
Array Configuration Types
When it comes to ultrasonic arrays, the arrangement of transducer elements can vary significantly, and each configuration has its own idiosyncrasies that cater to different applications. Commonly utilized configurations include:
- Linear Arrays: Ideal for surface imaging in medical diagnostics or material inspection. They provide a straightforward approach where elements are lined up along a straight path, ensuring uniform imaging across a designated area.
- Curved Arrays: These are notably advantageous for imaging purposes as they wrap around the target area. In echocardiography, for example, curved arrays help visualize the heart structure from multiple angles, enhancing the diagnostic capability.
- Matrix Arrays: The most complex, these arrays consist of a two-dimensional arrangement. They enable real-time 3D imaging, allowing for advanced procedures like abdominal scans or other detailed examinations. This versatility comes at a cost, requiring meticulous calibration and sophisticated signal processing algorithms.
Each of these configurations offers different advantages and limitations, where choices largely depend on the application demands, required resolution, and depth of field.
Element Spacing and Geometry
Another crucial factor lies in the spacing and geometry of the transducer elements. The distance between those elements, termed as "pitch," directly affects the resolution and beam width. Itās a balancing act.
- Narrow Spacing: Closer arrangement yields higher resolution but can lead to difficulties in isolating signals from closely situated points. This denseness might also result in increased cross-talk between channels, where signals interfere with each other.
- Wider Spacing: While it helps in reducing interference for clearer imaging, it may compromise spatial resolution. Practitioners need to consider the medium and the intended application when determining the optimal pitch.
A well-calibrated spacing can significantly enhance image clarity, but without proper setup, the results can be more akin to staring into a muddy pond rather than a clear window.
The geometry of the elementsāwhether circular, rectangular, or even custom shapesācan also influence how effectively ultrasonic waves are generated and received. A round design might be more appropriate for focused applications, whereas rectangular elements might spread energy more uniformly across a broader area.
Advent of Phased Array Technology
Phased array technology has revolutionized how ultrasonic arrays operate. Unlike traditional fixed arrays, phased arrays can electronically steer the beam direction and focus by controlling individual transducer element timing. This unique ability allows for:
- Real-time Imaging Adjustments: Operators can modify imaging without physically moving the transducer. This adaptability is of great benefit in dynamic situations, like during surgery or intricate inspections.
- Enhanced Resolution: With electronic steering, phased arrays can achieve finer detail by focusing energy precisely at varying depths or angles, making them exceptional for complex medical imaging tasks.
- Versatility Across Applications: Beyond healthcare, industries utilize phased arrays for checking weld integrity or finding flaws in materials, highlighting their multiple functionalities.
Operational Mechanics of Ultrasonic Arrays
The operational mechanics of ultrasonic arrays is pivotal in understanding how these intricate systems function and deliver results across various applications. From the subtle nuances of signal processing to the rigor of synchronization, each aspect weaves together to ensure the efficient operation of the arrays. Appreciating these dynamics fosters a deeper comprehension of not just the technology itself but also its implications in fields like medical imaging and industrial inspection.
Signal Processing Techniques
Signal processing is the backbone of any ultrasonic array, allowing it to convert raw acoustic data into meaningful information. These techniques include filtering, amplification, and sampling, each serving a specific purpose in enhancing the signal's clarity and relevance.
- Filtering is employed to eliminate unwanted noise, ensuring that the detected signals are as clean as a whistle. This is particularly critical when operating in noisy environments like factories, where background sound can confound the readings.
- Amplification increases the strength of the signals received. Without proper amplification, weak signals may be lost amidst the background hum of machinery or the body's own tissues, especially in medical ultrasound applications.
- Sampling rates need to be high enough to accurately capture the signal's characteristics but low enough to manage system load efficiently. A balance here is analogous to a tightrope walk; too low could mean critical data loss, while too high could strain processing capabilities.
"Signal processing not only dictates the performance but also determines the resolution and accuracy of ultrasonic systems."
Overall, mastering signal processing techniques ensures that the data derived from ultrasonic arrays is reliable and useful, ultimately influencing diagnosis, inspection, and material analysis outcomes.
Synchronization and Control Systems
Synchronization in ultrasonic arrays orchestrates the various components, ensuring they work in tandem. Just like a well-rehearsed orchestra, each transducer must fire at the correct time to produce coherent signals. This is essential for the array to accurately capture a comprehensive view of the subject being examined.
Control systems play a crucial role in this synchronization. They oversee the timing of pulse emissions and respond to feedback from received signals, effectively adjusting parameters in real-time. Some considerations within this realm include:
- Timing accuracy: Any timing errors can lead to distorted images or inaccurate measurements in medical diagnostics. A lag of mere milliseconds can skew results.
- Dynamic adjustment: Real-time changes in the operating environment can affect signal interpretation. Advanced control systems can react to these fluctuations, ensuring stable output.
- Integration with software algorithms: Modern systems often utilize sophisticated algorithms that not only aid in syncing but also previously discussed signal processing.
Having robust synchronization and control systems means data is collected in a systematic manner, maximizing efficiency and efficacy in all applications.
Energy Management in Array Operation
Managing energy is paramount when operating ultrasonic arrays, primarily because the array needs to sustain prolonged use without significant power drain. Energy management involves various strategies to optimize performance while ensuring reliability. Here are key points to consider:
- Power consumption: Understanding how much energy your array pulls is critical. Low power consumption ensures longer operation times without the need for frequent recharges or replacements.
- Battery technologies: Utilization of advanced battery solutions like lithium-ion or newer alternatives such as solid-state batteries can facilitate longer operational durations and quick recharge cycles, making devices incredibly portable.
- Heat management: Excessive heat can compromise the arrayās performance and longevity. Techniques such as passive or active cooling systems can be crucial to maintaining an optimal operating temperature.
In sum, effective energy management is not just about maintaining function; itās about creating robust systems that can be counted on in critical situations, whether thatās an emergency medical diagnosis or an inspection in a production line.
Applications in Medical Imaging
The domain of medical imaging has unarguably benefitted from the advancements in ultrasonic arrays. These devices harness sound waves to create images of internal body structures, offering non-invasive insights into patient health. With rising healthcare demands, ultrasound technology stands out for its effectiveness, safety, and cost-effectiveness when compared to alternatives like MRI or CT scans. This section will delve into distinct facets of ultrasonic technology in medical settings, laying out benefits and considerations for practitioners.
Ultrasound in Diagnostic Procedures
Ultrasound has made its mark in various diagnostic procedures, providing real-time imaging that is crucial for accurate assessments. One area of particular importance is obstetrics, where ultrasound scans are routine in monitoring fetal development.
- Real-time imaging: The dynamic real-time capabilities allow healthcare professionals to observe movements, abnormalities, or issues as they occur, enabling prompt intervention when necessary.
- Accessibility: Unlike other imaging techniques, ultrasound machines are often portable and more affordable, making them accessible in diverse settings, from bustling emergency rooms to remote clinics.
- Safety: There are no harmful radiation effects, which makes it safer, especially for pregnant women and developing fetuses.
The simplicity of using ultrasonic arrays makes them invaluable in various diagnostic contexts. They not only facilitate everyday exams but also extend into specialized domains, such as cardiology, where the heartās functioning can be closely monitored through echocardiograms. The feedback from these procedures ultimately guides treatment decisions, significantly impacting patient outcomes.
Therapeutic Uses of Ultrasonic Arrays
Besides diagnostic capabilities, ultrasonic arrays find utility in therapeutic contexts as well. This dual functionality enhances their overall significance in healthcare, as seen in various treatment methodologies:
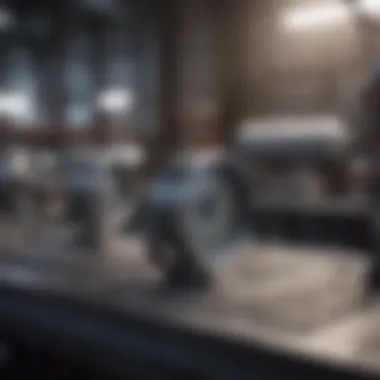
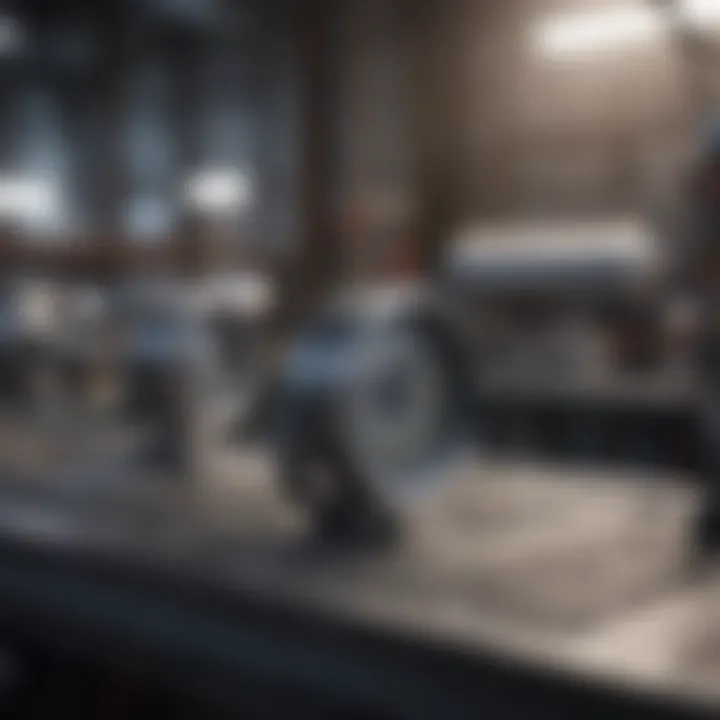
- Ultrasound-Guided Procedures: These include therapeutic interventions like biopsies and ablations, where precise targeting is essential for minimizing damage to surrounding tissues. By directing high-frequency sound waves, practitioners can ensure a higher success rate in these procedures.
- Physical Therapy: Ultrsound is commonly utilized in physiotherapy to aid muscle recovery, reduce pain, and improve blood circulation via deep tissue heating. The therapeutic effects promote more effective rehabilitative programs.
- Healing Mechanisms: Recent research suggests that ultrasound can stimulate cellular repair and regeneration processes. This could open up new avenues for treatment in various conditions.
As these applications grow, the considerations for ultrasound therapy must not be overlooked. Practitioners must carefully monitor settings and techniques to ensure safety and efficacy at all times.
Advancements in Imaging Technology
The swift pace of technological advancement has propelled ultrasonic arrays to new heights. The evolution of image clarity, resolution, and processing speed has transformed practices:
- 3D and 4D Imaging: Modern ultrasonic systems now provide comprehensive three-dimensional imaging capabilities, enhancing diagnostics in prenatal assessment and significantly improving anatomical visualization. The leap to 4D imagingāmonitoring live movementāhas also revolutionized fields like obstetrics.
- Portable Devices: The miniaturization of ultrasonic technologies allows for portable options that can be used outside traditional imaging facilities. This flexibility serves particularly well in emergency situations or underserved areas.
- Integration with AI: The advent of machine learning algorithms has bolstered image analysis, assisting in identifying patterns that might go unnoticed by the human eye. This can enhance diagnostic accuracy and efficiency.
"The marriage of advanced imaging technology with ultrasonic arrays can lead to groundbreaking improvements in patient care and clinical outcomes."
Industrial Applications of Ultrasonic Arrays
The significance of ultrasonic arrays in industrial settings cannot be overstated. They stand as a cornerstone in modern techniques for both quality assurance and product life cycle management. The following sections delve into specific applications of ultrasonic technology, reflecting its myriad benefits and considerations.
Non-Destructive Testing Methods
One of the most compelling uses of ultrasonic arrays lies in non-destructive testing (NDT). This technique allows for the evaluation of materials without causing any damage. In industries like aerospace and automotive, where safety is paramount, ultrasonic testing helps identify flaws such as cracks or voids in a material.
For instance, consider an aircraft wing. The implications of hidden defects are dire; undetected imperfections could lead to catastrophic failures. Therefore, ultrasonic testing offers a meticulous approach, enabling inspectors to customize frequency and intensity to gather precise readings about material integrity. It's a dance with sound waves, subtly revealing secrets hidden within solid structures.
"Non-destructive testing affords peace of mindāwithout compromising the integrity of the structure being tested."
Some key benefits of NDT using ultrasonic arrays include:
- High Sensitivity: Capable of detecting minute defects.
- Versatility: Applicable to various material types.
- Rapid Scanning: Time-efficient in both inspection and analysis.
In sum, ultrasonic arrays are indispensable for maintaining safety standards across numerous industries, providing thorough evaluations that are as informative as they are gentle on the materials they assess.
Material Characterization Techniques
Material characterization is another critical aspect where ultrasonic arrays shine. The identification of material propertiesālike elasticity, density, and internal structureāis essential in quality control and development. Ultrasonic techniques can yield insights into a material's behavioral patterns under different conditions, which in turn informs both design and manufacturing processes.
Using ultrasonic arrays, manufacturers can:
- Determine thickness variations, which helps in assessing wear and tear.
- Analyze material homogeneity, ensuring consistency across production batches.
- Conduct failure analysis by studying the physical responses of materials under stress.
The precision of ultrasonic testing means it allows for repetitive tests, crucial for monitoring changes over time. Firms that utilize these advanced techniques often find themselves on the cutting edge of material science.
Automation and Quality Control
As industries move toward faster, more efficient operations, the integration of ultrasonic arrays into automation workflows is becoming increasingly prevalent. These systems enable continuous monitoring in real-time, providing immediate feedback on material integrity and compliance. Imagine a production line where every item is inspected automatically, catching defects before they reach the customer.
Such automation leads to:
- Increased throughput: More items can be inspected in less time.
- Enhanced accuracy: Human error is minimized as machines perform tasks more consistently.
- Cost savings: Reducing the need for re-works and recalls.
Moreover, with ultrasonic technology being coupled with machine learning and AI, there's potential for even greater advancements. The systems can adapt and learn from historical data, refining processes and improving outputs steadily.
In summary, the application of ultrasonic arrays across industrial landscapes reveals an avenue ripe with possibilities. These technologies not only enhance safety and efficiency but also push the boundaries of whatās feasible in manufacturing and material sciencesāa true testament to innovation in action.
Emerging Technologies and Innovations
Emerging technologies are reshaping the landscape of ultrasonic arrays, offering innovative solutions that enhance performance and expand applicability. These advancements are crucial not only for the evolution of the technology itself but also for how it influences various industries, ranging from medical to manufacturing. Understanding these innovative trends is key for stakeholders looking to harness the full potential of ultrasonic systems, making this section vital for comprehending the modern framework of ultrasonic applications.
Integration with AI and Machine Learning
In recent years, the intersection of ultrasonic arrays with artificial intelligence and machine learning has sparked a transformative wave. Implementing AI algorithms alongside these technologies allows for better data analysis, improved image processing, and enhanced defect detection in industrial applications. For instance, deploying machine learning can facilitate real-time interpretation of ultrasound data, providing predictive analyses that can preemptively identify potential failures in materials.
Consider these benefits:
- Improved Accuracy: Machine learning can reduce human error by automating data interpretation.
- Efficiency: AI models can quickly analyze vast sets of data, offering insights almost instantaneously.
- Customization: Algorithms can be tailored for specific applications, enhancing performance based on unique operational conditions.
Thus, the implementation of AI in ultrasonic arrays doesn't just enhance existing applications; it introduces a paradigm shift that enables entirely new possibilities.
Miniaturization and Portable Devices
Another significant trend is the miniaturization of ultrasonic technology. As industries demand more portable and user-friendly solutions, manufacturers are rising to the occasion. This trend not only makes ultrasonic devices more accessible but also enhances their usability in field environments that require mobility and flexibility.
The miniaturization of ultrasonic arrays can bring about:
- Increased Accessibility: Smaller units are easier to transport and can be utilized in a variety of settings, such as remote medical clinics or on-site inspections in construction.
- Enhanced Functionality: Compact devices can integrate multiple functionsālike imaging, measurement, and diagnosticsāinto one unit, streamlining processes.
- Affordability: Reducing size often leads to lower material costs, making advanced technologies available to more users.
Portable ultrasonic devices are breaking barriers, allowing professionals to conduct accurate analyses without the limitations often posed by larger machinery.
Future Directions in Ultrasonic Array Research
Research in the field of ultrasonic arrays sees a promising horizon. Future developments are likely to focus on improving the resolution, sensitivity, and versatility of these systems. As the technology evolves, so too does the need for interdisciplinary collaboration, combining insights from various fields like material science, computer science, and signal processing.
Potential directions of this research may include:
- Enhanced Imaging Techniques: Advancements such as higher frequency transducers could lead to improved imaging capabilities, particularly useful in medical diagnostics.
- Adaptive Arrays: The development of arrays that can adjust their configurations dynamically in response to environmental changes or specific operational needs holds great potential.
- Sustainability Considerations: Focusing on environmentally friendly materials and processes can align ultrasonic technologies with sustainable development goals.
The overall expectation is a more integrated approach to ultrasonic research, focusing on collaboration across scientific disciplines and real-world applicability.
"With every leap in technology, we touch the future, and in ultrasonics, that future is palpably bright with possibilities."
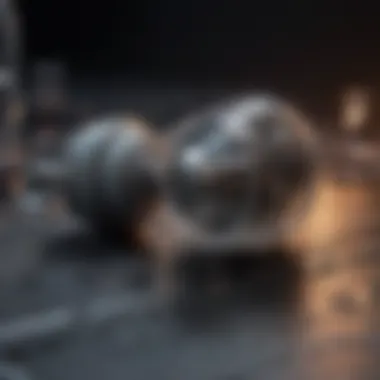
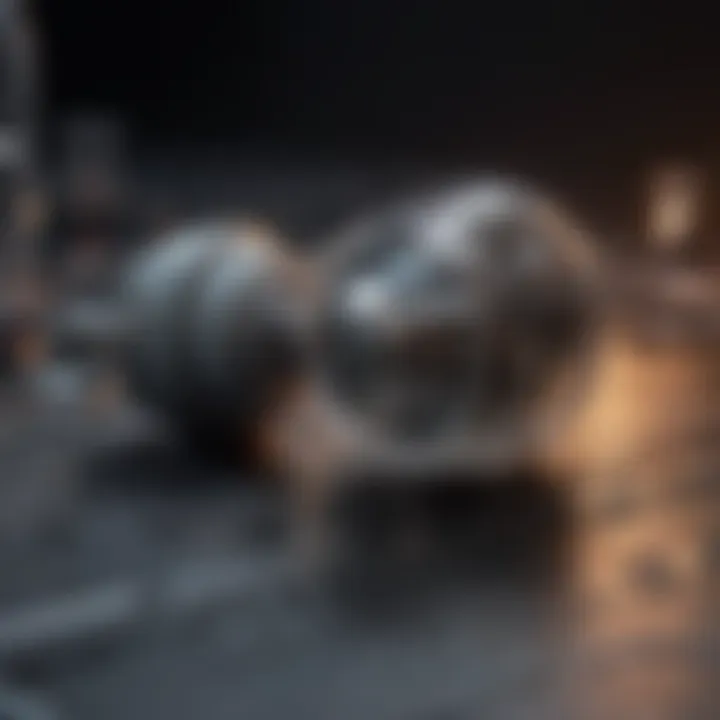
Through these evolving technologies and innovative directions, ultrasonic arrays stand on the brink of a revolutionāpoised to redefine the boundaries of their applications in the coming years.
Challenges and Limitations
Understanding the challenges and limitations of ultrasonic arrays is critical for advancing this technology. The insights gained from analyzing these elements not only help in identifying the existing gaps but also pave the way for innovations that can enhance performance, reliability, and safety across various applications. From technical constraints to economic considerations, each aspect plays a pivotal role in shaping the future of ultrasonic applications.
Technical Barriers
One of the primary obstacles in utilizing ultrasonic arrays effectively lies in technical barriers which can hinder their performance. Some of these barriers include:
- Complex Design Requirements: Designing an ultrasonic array is no walk in the park. Each component must be meticulously crafted to ensure optimal signal clarity and effective engagement with various media. A poorly designed system may lead to poor signal resolution, which could compromise the results of critical procedures, especially in medical diagnostics.
- Environmental Influences: Temperature fluctuations, noise interference, and the physical properties of the medium through which ultrasound travels can greatly impact the performance of an array. For example, variations in density or composition may distort wave propagation, leading to inaccurate readings.
"A small ripple in the conditions can create a wave of issues down the lineāespecially in high-stakes environments like healthcare."
- Technical Expertise: The operation and maintenance of ultrasonic systems require a level of expertise that can often be in short supply. Training staff to properly handle these sophisticated devices involves time and resources, which can be a stumbling block for many organizations.
Regulatory and Safety Issues
As with any technology utilized in sensitive fields, regulatory and safety issues often cast a long shadow over the deployment of ultrasonic arrays. Organizations must navigate a maze of regulations affecting their operation. Considerations include:
- Compliance with Standards: Different industries have varying regulations to ensure safety and effectiveness. For instance, healthcare providers need to adhere to strict guidelines set by bodies such as the Food and Drug Administration (FDA) when employing ultrasonic technology for diagnostics or treatment. This compliance can be a complex, resource-consuming task.
- Risk of Misdiagnosis: The stakes are particularly high in medical imaging; if an ultrasound fails to produce accurate results, the ramifications can be dire. Misdiagnoses or delayed treatments can lead to irreversible damage to patient health. Therefore, ensuring the reliability and accuracy of the equipment is paramount.
- Potential Hazards: While ultrasonic arrays are generally considered safe, the risk of exposure to high-intensity sound waves leads to concerns. Structures and safety protocols must be established to protect both medical personnel and patients from potential hazards.
Cost-Effectiveness Analysis
When it comes to implementing ultrasonic technology, a keen eye on cost-effectiveness can make or break a project. Here are some factors to consider:
- Initial Investment vs Long-Term Benefits: Ultrasonic arrays often require a significant upfront investment, which can serve as a barrier for many smaller organizations. However, understanding that a well-functioning array can lead to increased efficiency and reduced costs in the long run is crucial for decision-makers. The ability to carry out non-destructive testing or high-fidelity imaging can save considerable costs in alternative methods.
- Maintenance and Training Costs: The total cost of ownership extends beyond just the purchase price. Regular maintenance and training for staff add to the overhead, and these ongoing expenses must be factored into any cost-benefit analysis.
- Market Variability: Prices for ultrasonic devices can fluctuated based on technological advancements and competition. Decisions made today might lead to obsolescence sooner than anticipated, prompting additional investments in newer technology or upgrades.
In summary, addressing the challenges and limitations of ultrasonic arrays is vital for enhancing their effectiveness and reliability. Understanding these factors can help guide future research efforts, regulatory decisions, and innovations that continue to push the boundaries of what is possible with this technology.
Comparative Analysis with Other Technologies
Understanding the comparative dynamics of ultrasonic arrays versus other technological methodologies is crucial for grasping their unique advantages and potential limitations. This section looks at various aspects of ultrasonic arrays in contrast to electromagnetic methods, highlights their strengths and weaknesses, and provides an application-specific overview of their effectiveness and efficiency.
Ultrasonics versus Electromagnetic Methods
Ultrasonic technology and electromagnetic methods serve diverse roles in industry and research, yet their operational principles set them apart significantly.
- Principle of Operation: Ultrasonics rely on high-frequency sound waves to interact with materials, while electromagnetic systems function by utilizing electric and magnetic fields to gather information about objects.
- Depth of Penetration: Ultrasonic waves can penetrate deeper into materials compared to electromagnetic waves, which often face challenges in complex mediums, especially conductive materials.
- Sensitivity to Material Properties: Different materials respond uniquely to ultrasonic waves, allowing for nuanced assessments, particularly in non-destructive testing. Electromagnetic methods may lack this level of adaptability, being more reliant on surface conditions.
"In scenarios where depth and material integrity are paramount, ultrasonic approaches often reign supreme."
Strengths and Weaknesses
Each method possesses distinct characteristics influencing their application scope:
Strengths of Ultrasonic Arrays:
- Non-Destructive: They can evaluate material integrity without causing damage.
- Versatility: Function efficiently across various mediums, including liquids and solids.
- Cost-Effective in the Long Run: Although initial setup may require investment, the long-term savings on material wastage are significant.
Weaknesses of Ultrasonic Arrays:
- Limited External Conditions: Often not suited for very high-temperature environments or extreme pressures.
- Dependence on Skill Level: Results can be highly operator-dependent, requiring trained professionals for accurate interpretations.
Strengths of Electromagnetic Methods:
- Speed of Operation: Generally faster in data acquisition and processing.
- Surface Analysis: More effective for evaluating surface conditions and features.
Weaknesses of Electromagnetic Methods:
- Limited Depth: Struggles to penetrate dense materials.
- Complexity in Processing: Requires sophisticated algorithms for data interpretation, increasing potential for errors.
Application-Specific Comparisons
When evaluating specific applications, the choice between ultrasonic and electromagnetic technologies may hinge on the unique requirements of the task:
- Non-Destructive Testing in Aerospace: Ultrasonic methods dominate here due to their ability to analyze complex geometries without compromising integrity.
- Medical Imaging: Ultrasound plays a pivotal role in diagnostic imaging, providing real-time insights that electromagnetic tools like MRI might not deliver as effectively.
- Quality Control in Manufacturing: Both methods find their places, but ultrasonic testing is often preferred for structural integrity testing, particularly with welds and joints.
Incorporating these insights facilitates a deeper understanding of the complex interplay between ultrasonic arrays and other technologies, aiding in advancing research and practical applications.
Ending
The conclusion of the article serves as a pivotal point to synthesize the intricate themes explored throughout the various sections. It brings closure to the discussion on ultrasonic arrays by summarizing essential findings and outcomes, highlighting why these arrays are indispensable across several fields such as medicine and industrial inspection.
Key insights culled from this exploration reveal that ultrasonic arrays not only enhance diagnostic capabilities but also bolster non-destructive techniques, thereby ensuring safety and quality in material evaluations. The melding of advanced technology with practical applications underscores the remarkable flexibility and potential of these systems. We see their true value in how they aid in image clarity during medical procedures, streamline industrial audits, and even play a role in research settings for complementary technologies.
Summary of Key Insights
- Versatility in Use: Ultrasonic arrays are adaptable across sectors, making them suitable for diverse applications like medical diagnostics and industrial inspections.
- Technological Innovation: Continuous integration of AI and machine learning enhances their precision and utility, opening the door to smarter systems in the future.
- Cost-Effectiveness: While initial investments may seem steep, the long-term benefits and efficiency justify the outlay, making them attractive in both medical and industrial contexts.
"Innovation paired with robust applications in ultrasonic arrays ensures they are not just a niche technology but a mainstream solution with vast possibilities."
Implications for Future Research
Looking ahead, several opportunities are ripe for exploration that can significantly augur the trajectory of ultrasonic arrays:
- Enhanced Signal Processing: Research into more sophisticated algorithms for signal interpretation can lead to clearer imaging and more accurate data collection.
- Greater Integration with Smart Tech: Investigating how ultrasonic arrays can work synergistically with wearable tech and smart devices poses a vast frontier for studies.
- Environmental Impact Assessments: Understanding how to optimize these arrays for ecological monitoring can pave the way for more sustainable practices in construction and infrastructure assessment.
Call to Action for Advancements
The findings presented in this article should incite a robust push among researchers, developers, and practitioners to further investigate and refine the technology behind ultrasonic arrays. Collaborations among academia, industry, and regulatory bodies can foster the following actions:
- Investment in Cutting-Edge Research: Funding for innovative projects focused on the integration of AI in ultrasonic systems.
- Standardization of Practices: Establishing guidelines and standards for the implementation and use of ultrasonic arrays in various fields.
- Educational Programs: Developing programs to train professionals in understanding and utilizing these technologies effectively.
By committing to these advancements, stakeholders can not only harness the existing capabilities of ultrasonic arrays but also shape a future where they lead to groundbreaking solutions across numerous disciplines.