Exploring Voltage Sensitive Dyes: Mechanisms and Applications
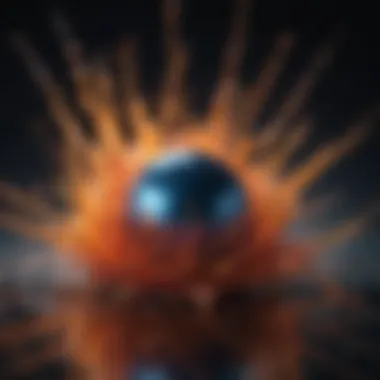
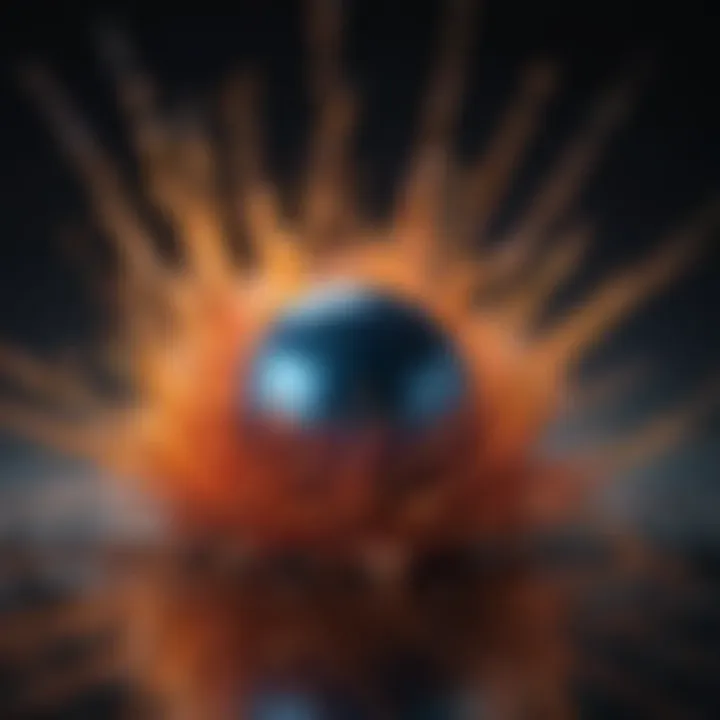
Intro
Voltage-sensitive dyes (VSDs) are innovative tools used predominantly in the fields of neuroscience, cardiology, and cellular biology. These dyes are capable of detecting changes in membrane potential, providing researchers with insights into the electrical activities of cells. Their unique ability to respond to voltage fluctuations opens new avenues for understanding cellular processes and mechanisms, making them a vital topic of study within scientific and medical communities.
As researchers continue to explore the principles behind these dyes and their applications, it becomes increasingly essential to grasp the underlying theories and techniques that contribute to their functionality. In this section, we will summarize the critical findings related to voltage-sensitive dyes, followed by an exploration of their significance in contemporary research and clinical practices.
Research Overview
Voltage-sensitive dyes, primarily based on molecular frameworks that shift their absorption or emission properties in response to voltage changes, have emerged as pivotal resources for studying dynamic electrical events in living tissues.
Summary of key findings
Recent studies have unveiled several important characteristics of these dyes:
- Mechanism of Action: The fundamental principle revolves around the interaction of the dye's molecular structure with electric fields across cell membranes, leading to measurable optical changes.
- Performance Enhancements: Continuous advancements in dye formulations have improved their sensitivity and selectivity, enabling higher resolution imaging of cellular voltage dynamics.
- Application Diversity: Voltage-sensitive dyes are utilized across various disciplines, including examining neuronal action potentials, cardiac rhythm abnormalities, and the behavior of other excitable cells.
Importance of the research in its respective field
Understanding the mechanisms and applications of voltage-sensitive dyes is crucial for several reasons. First, it allows researchers to visualize cellular activities in real time, offering unprecedented ways to study the intricacies of cell communication and function. The insights gained contribute significantly to the understanding of neural networks, cardiac function, and the behavior of other electrically active tissues. As such, the implications of this research extend into the realms of medical diagnostics and treatment strategies, enabling researchers to devise potential therapies for a range of voltage-dependent disorders.
Methodology
To explore voltage-sensitive dyes effectively, rigorous methodologies must be employed. Various experimental and analytical techniques help to uncover the nuances of how these dyes operate and contribute to scientific knowledge.
Description of the experimental or analytical methods used
Common methods in the study of voltage-sensitive dyes include:
- Fluorescence Imaging: This technique employs lasers to excite the dye molecules, observing the emitted light to determine voltage changes.
- Electrophysiological Recordings: Combining VSDs with traditional electrophysiology allows for a comprehensive analysis of cell behaviors.
- Computational Modeling: Simulations help predict dye behavior and optimize experimental conditions.
Sampling criteria and data collection techniques
Researchers typically select specific cell types based on the research focus, such as neurons in the case of neurophysiology studies. For data collection, optical signals from VSDs can be quantified using specialized imaging software and custom-designed setups to capture the dynamics of voltage changes during experiments. This combination of sampling and analysis fosters a thorough understanding of the role of voltage-sensitive dyes in living systems.
The understanding and application of voltage-sensitive dyes push the boundaries of cellular imaging and contribute significantly to advancements in both research and clinical diagnostics.
Foreword to Voltage Sensitive Dyes
Voltage sensitive dyes (VSDs) are innovative tools that enable scientists to visualize and measure electrical activity in living cells. They have gained prominence in various research fields, particularly neuroscience and cardiology. The ability to monitor voltage changes in real-time is crucial for understanding complex biological systems. VSDs offer insights into cellular dynamics that traditional methods may overlook.
Definition and Importance
Voltage sensitive dyes are fluorescent compounds that can indicate changes in membrane potential. When a cell's electrical state changes, the dye's fluorescence properties also change. This characteristic allows researchers to map out electrical activity in cells with high spatial and temporal resolution.
The importance of VSDs lies in their capability to provide dynamic information about how cells communicate and react to stimuli. For instance, in neuroscience, these dyes can reveal how neurons transmit signals. In cardiology, they can help analyze how hearts respond to electrical impulses. This information is invaluable for advancing our understanding of various physiological processes, as well as developing therapeutic interventions for diseases.
Historical Context
The development of voltage sensitive dyes dates back to the mid-20th century when scientists began exploring the properties of fluorescent markers. Initial research was driven by the need to monitor neuronal activity, which is fundamental to understanding brain function. Early VSDs had limitations, including poor signal-to-noise ratios and inadequate stability.
Advancements in chemical synthesis and imaging technologies have transformed VSDs over the decades. The introduction of modern dyes in the 1990s marked a significant shift. Dyes like Di-4-ANEPPS and others have dramatically improved the performance characteristics of these compounds. As a result, VSDs have become a staple in both basic research and applied sciences.
Chemical Basis of Voltage Sensitive Dyes
Understanding the chemical basis of voltage sensitive dyes is crucial for comprehending their role in biological imaging and potential applications. The performance of these dyes greatly relies on their molecular structure and how they interact with voltage changes in membrane potentials. Their importance in both research and clinical practices stems from the ability to visualize electrical activities in cells, making it an invaluable tool in neuroscience and cardiology.
Molecular Structure
Voltage sensitive dyes often have a distinct molecular structure allowing them to respond to changes in electrical potential across membranes. These dyes typically consist of conjugated systems that impart polarity sensitivity. The key attributes of their molecular structure include:
- Conjugated Bonds: The presence of alternating single and double bonds facilitates the delocalization of electrons. This property is essential for the dye's ability to change absorbance or fluorescence in response to voltage changes.
- Charge Dynamics: Certain voltage sensitive dyes are designed to include charged groups, which contribute to their affinity for cellular membranes. The interaction between the dye and the membrane can lead to significant changes in the dye's optical properties, correlating with the membrane's voltage state.
- Stability: The chemical stability of these dyes impacts their usability in live cell imaging. Structures that resist photobleaching ensure that the fluorescent signals remain strong during extended imaging periods.
The molecular structure not only defines how the dye behaves under different conditions but also influences its effectiveness in specific applications, such as imaging neuronal activity.
Mechanism of Action
The mechanism of action of voltage sensitive dyes involves a transition between different electronic states in response to voltage changes, resulting in measurable optical signals. This action can be understood in the following steps:
- Voltage Sensing: When a dye is introduced into a cell, it incorporates into or near the membrane. A change in membrane potential alters the local electrical field, affecting the dye's properties.
- Fluorescence Changes: As the membrane potential varies, the dye undergoes a shift in its electronic state. This transition usually leads to a change in fluorescence intensity or wavelength. For instance, certain dyes may emit more light when the membrane potential is depolarized.
- Signal Acquisition: The emitted signal is captured using specialized imaging techniques. The intensity and wavelength of this light provide insights into the electrical state of the cell.
A clear understanding of this mechanism is imperative. It helps researchers choose the appropriate dye for their specific imaging needs and informs strategies to mitigate potential challenges in signal clarity.
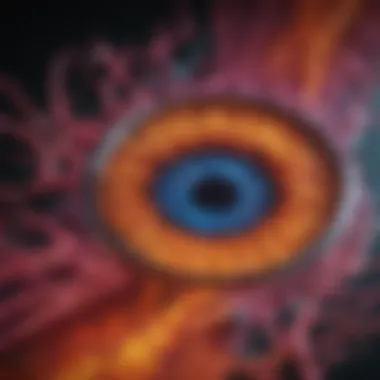
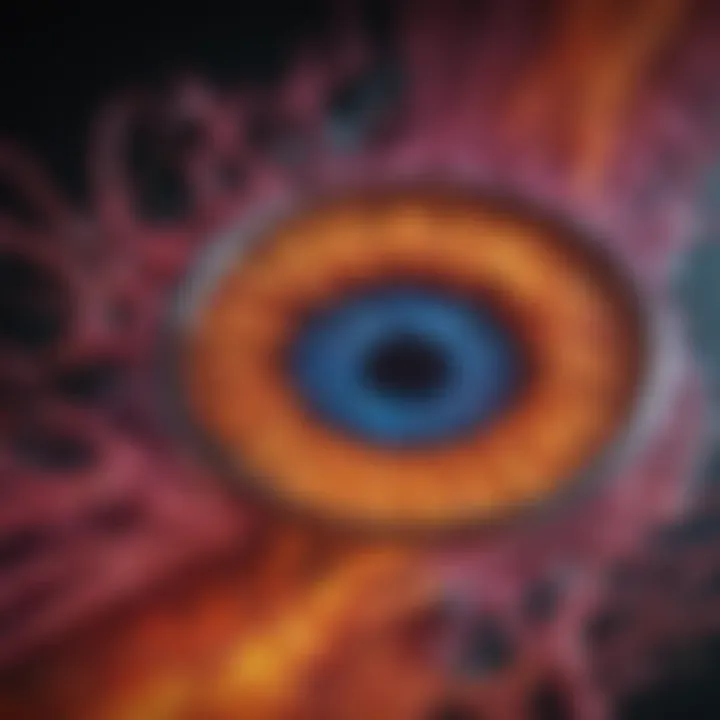
"The capacity of voltage sensitive dyes to concurrently monitor multiple sites makes them instrumental in mapping electrical activities across complex tissues."
In summary, the chemical basis for voltage sensitive dyes encompases the molecular structure and mechanism of action, which highlights their utility in scientific research and medical diagnostics. Knowledge of how these components work together is essential for exploiting their full potential in understanding cell dynamics.
Types of Voltage Sensitive Dyes
Understanding the diversity of voltage sensitive dyes is essential for grasping their applications across various scientific domains. Each type serves unique purposes, reflecting its underlying principles of operation. This section will examine traditional dyes, modern innovations, and conclude with a comparative analysis of the two families of dyes. By elucidating the essential aspects of these dyes, we aim to provide clarity on their significance in research.
Traditional Dyes
Traditional voltage sensitive dyes have long been instrumental in experimental setups. Among them, Di-4-ANEPPS and Oxonol dyes are notable examples. These dyes have been extensively utilized in both cardiac and neuronal imaging. Their chemical properties facilitate the measurement of membrane potentials by altering fluorescence in response to voltage changes.
Typically, they are characterized by a relatively straightforward design, generally consisting of a hydrophobic region for membrane integration and a polar head for interaction with the intracellular environment. This design contributes to their capability to navigate through lipid membranes, where they exert their voltage sensitivity.
Some significant advantages of traditional dyes include:
- Established use in various studies, allowing for comparability across research.
- Well-studied mechanisms of action, providing reliable performance.
- Relatively cost-effective options.
However, they encounter limitations such as potential phototoxicity and sensitivity to environmental factors. These drawbacks highlight the necessity for continued innovation in dye technology to enhance efficacy and safety.
Modern Dyes and Innovations
Modern voltage sensitive dyes introduce advanced features that overcome shortcomings of their traditional counterparts. One prominent example is the use of genetically encoded voltage indicators (GEVIs), such as Arch and QuasAr. These innovative tools are engineered to offer high sensitivity and specificity towards voltage changes in neurons.
Modern dyes often exhibit:
- Higher Signal-to-Noise Ratios: This improves the quality of imaging data, allowing for more accurate interpretation of cellular dynamics.
- Reduced Phototoxicity: Newer formulations tend to mitigate damaging effects on living tissue, making them more suitable for long-term studies.
- Broader Spectral Range: Innovations allow for multiplexing wherein multiple voltage indicators can be imaged simultaneously.
These dyes push the boundaries of what's possible in cellular imaging. They enable finer granularity in assessing electrical activities, significantly benefiting the study of neuronal processes and cardiac function.
Comparison of Dye Families
In comparing traditional and modern voltage sensitive dyes, several factors merit attention:
- Sensitivity and Precision: Modern dyes typically show enhanced voltage resolution. Traditional dyes can often miss rapid, transient voltage fluctuations.
- Ease of Use: Traditional dyes may require less sophisticated equipment, whereas modern innovations could necessitate advanced imaging systems.
- Application Scope: Modern dyes are increasingly adaptable across various fields, while traditional dyes are often limited to specific applications.
Applications in Neuroscience
Voltage sensitive dyes have transformed the field of neuroscience significantly. Their ability to provide real-time insights into neuronal activity serves as a critical tool for researchers aiming to understand the complexities of brain function. By enabling visualization of changes in membrane potential, these dyes allow scientists to monitor the electrical activity of neurons non-invasively. The impact of such techniques can be profound, offering a window into dynamic processes such as synaptic transmission and network interactions.
The benefits of voltage sensitive dyes extend to various applications. They facilitate the study of neuronal firing patterns, enabling a more nuanced understanding of how information is processed in the brain. This ability to visualize neuronal activity in real time has made voltage imaging a popular choice for electrophysiological studies, complementing traditional methods like patch clamping. Furthermore, because voltage sensitive dyes can be used in live tissue, they provide a unique perspective on how neurons behave within their native environments.
In this article, we will delve into two key aspects of voltage sensitive dyes in neuroscience: imaging neuronal activity and studying network dynamics and plasticity. Each subsections will highlight the ways in which these technologies have advanced our understanding of brain function and their continued relevance in current research.
Imaging Neuronal Activity
Imaging neuronal activity with voltage sensitive dyes has opened new avenues for exploring brain physiology. By using these dyes, researchers can capture rapid changes in voltage when neurons are activated. The fluorescence emitted by the dyes correlates with the changes in membrane potential. This effect allows for the visualization of action potentials and other rapid electrical signals.
The application of voltage sensitive dyes in imaging has proven essential in understanding how neuronal circuits function. For example, in cortical slice preparations and whole-brain imaging, these dyes enable visualization of neuronal behavior across complex networks. Importantly, with advances in imaging techniques, such as multi-photon microscopy, it is now possible to gather data from multiple neurons simultaneously. This capacity enriches our understanding of network-level interactions, highlighting how neurons cooperate to process information.
Moreover, real-time imaging with these dyes allows neuroscientists to observe how drug interventions, genetic modifications, or disease processes affect neuronal function. This has significant implications not only for basic neuroscience but also for translational research aimed at finding new treatments for neurological disorders.
Network Dynamics and Plasticity
Voltage sensitive dyes are crucial in studying network dynamics and plasticity, which refer to the brain's capability to change and adapt. Plasticity is a fundamental property that underlies learning and memory. The ability to visualize and quantify network activity allows researchers to assess how synaptic connections strengthen or weaken over time.
Through the use of voltage sensitive dyes, researchers have investigated how patterns of activity can lead to changes in synaptic efficacy. For instance, during long-term potentiation (LTP) or long-term depression (LTD), these dyes can map the spatial and temporal dynamics of neuronal excitation within networks. This provides insight into the molecular frameworks underlying neuronal plasticity. In addition, voltage imaging can reveal how disruptions in these processes may relate to cognitive deficits or neurological conditions.
Applications in Cardiology
The use of voltage-sensitive dyes in cardiology allows for detailed studies of cardiac function. Analyzing cardiac action potentials and understanding arrhythmias are crucial to the field. With advancements in imaging technologies, researchers can visualize cardiac activity with great accuracy. This contributes significantly to both diagnostic and therapeutic approaches in cardiology.
Cardiac Action Potentials Imaging
Voltage-sensitive dyes are instrumental in imaging cardiac action potentials. These dyes can indicate changes in membrane potential, which is essential in understanding how heart cells communicate and function. By applying these dyes, researchers can capture high-resolution images of electrical activity in the heart. This technique allows for the assessment of normal cardiac rhythms as well as identifying deviations, such as those seen in heart disease.
The ability to monitor rapid electrical changes in real-time has led to breakthroughs in understanding cardiac physiology. It provides insights into conduction pathways and helps map out the heart's electrical system. Such imaging is vital for developing better treatment protocols for various cardiac conditions.
Arrhythmia Research
Arrhythmias present a significant challenge in cardiology, affecting millions of patients worldwide. Voltage-sensitive dyes have a pivotal role in arrhythmia research by enabling scientists to visualize abnormal electrical activities with high precision. Understanding the mechanisms behind arrhythmias can lead to more effective interventions and treatment plans.
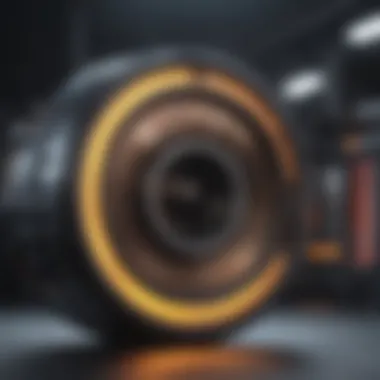
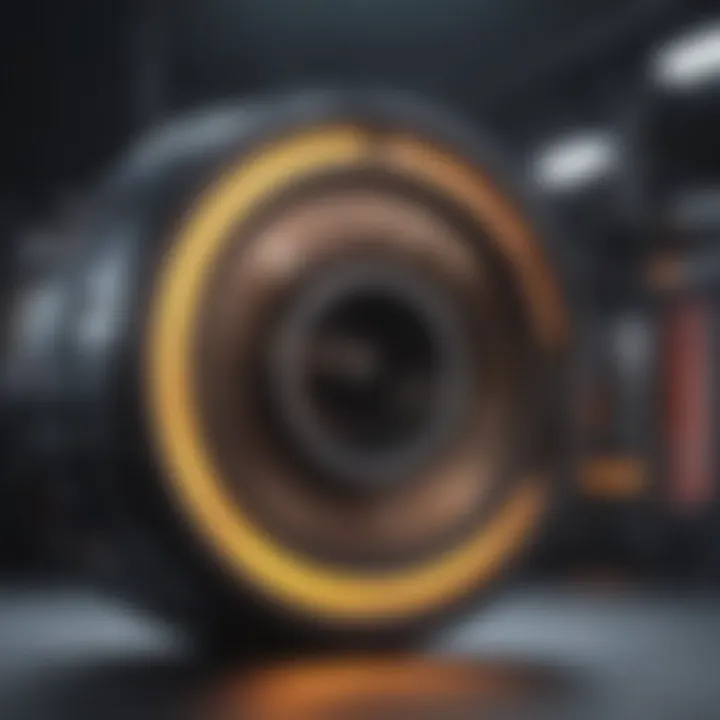
Using voltage dyes, researchers can study the initiation and propagation of arrhythmias under different conditions. This knowledge can aid in identifying potential therapeutic targets. Utilization of these imaging techniques provides essential data that can help differentiate between various forms of arrhythmia, offering a tailored approach to patient care.
In summary, voltage-sensitive dyes prove to be essential tools in cardiology. They enable detailed imaging of cardiac action potentials, leading to improved understanding of heart physiology and the management of arrhythmias. \n
"The integration of voltage-sensitive dyes in cardiology is transforming our approach to cardiac rhythm disorders and offers hope for more personalized treatments."
The ongoing research is crucial for advancing our understanding of cardiovascular health and enhancing the quality of care for patients.
Challenges in Using Voltage Sensitive Dyes
The utilization of voltage-sensitive dyes in scientific research brings significant advantages, yet it also presents various challenges that affect their adoption and effectiveness. Understanding these challenges is crucial for researchers who aim to leverage these tools for studying cellular activities. This section will delve into the specific issues associated with the use of these dyes, including stability and phototoxicity as well as signal-to-noise ratio concerns.
Stability and Phototoxicity
Stability is a fundamental concern when working with voltage-sensitive dyes. Many of these dyes can degrade over time, leading to unreliable results during experiments. Environmental factors, such as light exposure and temperature fluctuations, can exacerbate the degradation process. Ensuring the longevity of the dye is necessary for acquiring consistent and reproducible data.
Phototoxicity is another critical aspect linked to the use of these dyes. When voltage-sensitive dyes are exposed to light, they can induce damage to cellular structures and influence biological processes. This phenomenon can affect the viability of the cells being studied and may alter the physiological responses that researchers intend to test. Minimizing phototoxicity is essential, particularly in live-cell imaging applications. Researchers may need to optimize light exposure duration and intensity to mitigate these adverse effects while still obtaining clear imaging results.
Practices to manage stability and phototoxicity effectively include:
- Keeping dyes in dark, temperature-controlled environments when not in use.
- Utilizing low-power light sources whenever possible.
- Testing the effects of different concentrations of the dye to find a balance between visibility and cellular impact.
Signal-to-Noise Ratio Issues
The signal-to-noise ratio (SNR) is a pivotal element in the performance of voltage-sensitive dyes, as it directly influences the quality of acquired images. A favorable SNR enables clearer detection of voltage changes, whereas a low SNR can obscure true signals due to background noise.
Factors contributing to SNR challenges include dye concentration, imaging equipment, and the specific biological environment. High concentrations of voltage-sensitive dyes can enhance the signal but may also lead to increased background fluorescence, thereby diminishing SNR. On the other hand, lower dye concentrations may reduce background noise but compromise signal visibility. Achieving the right balance is crucial to optimize observational clarity.
Furthermore, the imaging system's inherent characteristics play a role in determining SNR. Cameras and microscopy techniques vary in sensitivity and resolution. Selecting the appropriate tools for imaging and utilizing effective computational methods to filter out noise can help ameliorate this issue.
In summary, challenges like stability, phototoxicity, and signal-to-noise ratio are vital considerations in the use of voltage-sensitive dyes. Addressing these issues is essential to fully exploit the potential of these dyes in research settings, ensuring that data obtained is both reliable and impactful.
Advancements in Imaging Techniques
The realm of imaging techniques has seen substantial evolutions, particularly in the context of voltages sensitive dyes. Advancements in this field not only enhance the resolution of imaging but also broaden the scope of applications. As researchers aim to investigate complex biological systems, innovative imaging methods capable of capturing rapid changes in voltage across membranes become essential.
Several benefits arise from these advancements. Increased temporal resolution allows for capturing fast physiological processes, facilitating insights into dynamic cellular behavior. Moreover, better spatial resolution provides clarity in understanding cell structures and their voltage dynamics. These enhancements also contribute to more accurate diagnostics and improved therapeutic strategies, especially in fields like neuroscience and cardiology.
Time-Resolved Imaging
Time-resolved imaging represents a critical advancement in the use of voltage-sensitive dyes. This technique enables scientists to capture images at specific intervals, creating a dynamic representation of cellular processes over time. By adjusting the imaging speed, researchers can observe how voltage dynamics unfold during significant events such as action potentials in neurons.
This imaging method utilizes sophisticated timing mechanisms to synchronize the capture of images with physiological events. It provides data that reveal patterns and anomalies in voltage behavior that might be missed with conventional imaging techniques. Time-resolved imaging is particularly important in understanding transient phenomena, such as synaptic transmission in neurons or rapid electrical changes in cardiomyocytes.
- Benefits of Time-Resolved Imaging:
- Enables observation of rapid voltage changes.
- Offers insights into temporal dynamics of cellular activities.
- Critical for studying fast signaling processes across membranes.
Multi-Photon Microscopy
Multi-photon microscopy is another leap forward in imaging technologies applied to voltage-sensitive dyes. This technique allows for deep tissue imaging with minimal photodamage and high spatial resolution. The advantages of using multi-photon microscopy include the capability to monitor events in three dimensions and the ability to visualize activity in live tissues over extended periods.
The fundamental working principle involves the simultaneous absorption of two or more photons of lower energy, which compensates for the higher energy required for electron excitation. This results in less scattering and absorption, enabling imaging of denser biological tissues. The resolution and depth capability rendered by this technology are unparalleled, allowing for detailed exploration of complex networks in brain tissues or cardiac structures.
- Advantages of Multi-Photon Microscopy:
- Minimizes photodamage during imaging.
- Allows for high-resolution imaging deep within tissues.
- Facilitates the study of complex cellular interactions in real time.
Future Directions in Voltage Sensitive Dyes Research
The future of voltage sensitive dyes (VSDs) is quite promising, as ongoing research continues to refine and enhance their utility across various domains, particularly in neuroscience and cardiology. The significance of exploring new avenues in VSD technology lies in the continual push towards more precise, efficient, and effective methods for imaging cellular activity. With advancements in synthesis methodologies of next-generation VSDs, researchers aim to overcome current limitations such as signal-to-noise ratio issues and stability challenges.
This section will highlight two specific elements critical to the future developments of VSDs: next-generation dyes and integration with other technologies. Each of these elements carries unique benefits that can potentially redefine the landscape of cellular imaging.
Next-Generation Dyes
New materials and innovative approaches to dye formulation are paving the way for next-generation voltage sensitive dyes. These next-generation products aim to address key shortcomings seen in traditional dyes. For instance, improvements in molecular structure can lead to better performance under specific imaging conditions.
A few benefits of next-generation dyes include:
- Higher sensitivity to voltage changes: Enhanced sensitivity ensures that even minute changes in membrane potentials can be detected accurately.
- Reduced phototoxicity: Innovations aimed at minimizing phototoxic effects are vital for long-term imaging, particularly in live cell studies.
- Broader spectral range: Next-generation dyes may offer flexibility in application by emitting light at various wavelengths. This would allow their use in multiplexing applications, a technique important for studying multiple types of cellular activities simultaneously.
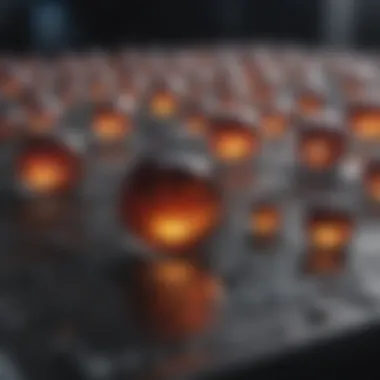
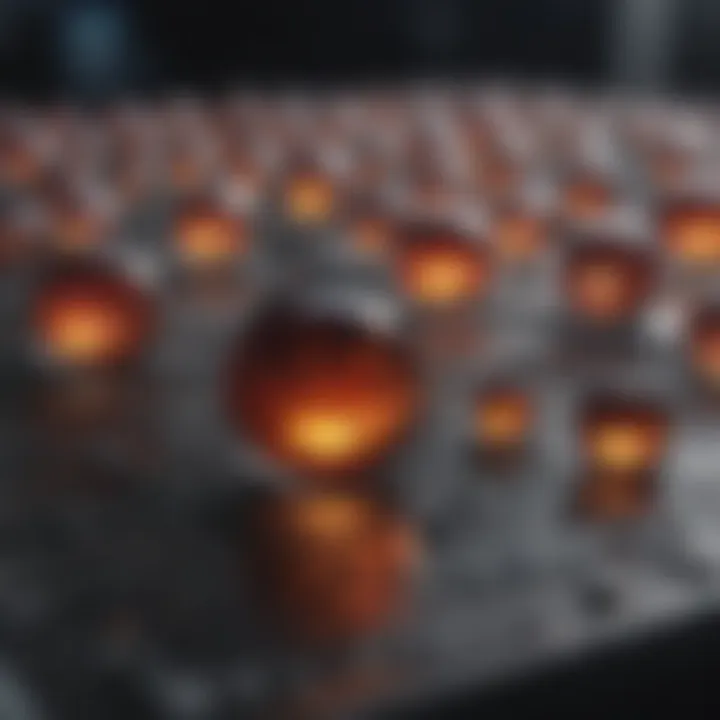
The development of such dyes is not merely an improvement upon existing technology; it significantly broadens the scope of experiments that can be conducted and interpreted in vivo.
Integration with Other Technologies
The integration of voltage sensitive dyes with other advanced imaging technologies holds substantial promise to enhance the capacity of researchers to analyze cellular dynamics. This synergy can unlock new insights into cellular mechanisms and intercellular communications.
Some noteworthy points regarding this integration include:
- Coupling with optogenetics: Combining VSDs with optogenetic tools allows for precise control over the timing and level of stimulation in neuronal circuits, making it possible to elucidate the relationship between electrical activity and cellular behavior.
- Application in machine learning: Employing machine learning algorithms on VSD data can help identify complex patterns in neuronal firing or cardiac rhythms that would not be evident through standard analysis methods.
- Utilizing hybrid imaging techniques: Integrating VSDs with technologies like PET (Positron Emission Tomography) and MRI enhances both spatial and temporal resolution of cellular events. Such hybrid methods provide a comprehensive view of how voltage changes relate to structural and functional aspects of cells.
The future of voltage sensitive dyes is not just about incremental improvements but rather about embracing multidisciplinary approaches that can transform our understanding of cellular activities.
In summary, the future directions in VSD research reflect a critical interface between innovative dye development and the integration of advanced technologies. These elements not only push the boundaries of current capabilities but also hold considerable implications for translational research and clinical applications.
Impact on Scientific Understanding
Understanding voltage-sensitive dyes plays a vital role in advancing several scientific fields. Their ability to provide real-time imaging of electrical activity in cells contributes significantly to our knowledge of biological systems. Notably, these dyes are crucial for elucidating events such as neuronal firing and cardiac function. By visualizing voltage changes, we can correlate physiological processes with cellular behavior.
Contributions to System Biology
In system biology, voltage-sensitive dyes enhance our grasp of complex interactions within biological systems. This is achieved through the study of electrical activities of cells in various tissues. For example, imaging neuronal circuits with these dyes enables a more profound understanding of synaptic transmission and neural network dynamics. Researchers can observe how neurons communicate during various tasks and behaviors.
Moreover, voltage-sensitive dyes facilitate the exploration of cellular responses in real time. As a result, scientists can detect shifts in membrane potential associated with signaling pathways or metabolic changes. Such insights refine our understanding of how cells adapt to internal and external stimuli. By mapping these patterns, researchers can identify potential biomarkers for diseases and conditions that disrupt normal electrical signaling in the body.
Translational Research Potential
The translational research potential of voltage-sensitive dyes is significant. They can be applied beyond basic research into clinical settings, where understanding electrical activity can improve diagnosis and treatment. For instance, these dyes are useful in identifying arrhythmias and other cardiac conditions. Real-time voltage imaging can aid in pinpointing the exact locations of electrical disturbances in heart tissues, guiding therapeutic interventions.
Furthermore, their application in neuroscience can lead to advancements in treating neurological disorders such as epilepsy. Observing the propagation of electrical signals can help recognize abnormal patterns leading to seizures. Such knowledge has the potential to inform targeted therapies that restore normal function.
"The evolution of voltage-sensitive dye technologies signifies a remarkable stride towards unraveling the complexities of living systems and enhancing clinical outcomes."
In summary, the impact of voltage-sensitive dyes on scientific understanding is multifaceted. These dyes bridge core concepts of biology with practical applications, fostering innovation in various research arenas. As the technologies continue to develop, we can expect even greater contributions to the biological and medical sciences.
Ethical Considerations
Ethical considerations play a crucial role in the application and development of voltage-sensitive dyes (VSDs). As researchers continue to utilize these dyes in various studies, the implications for both human and animal subjects become increasingly significant. The ethical landscape surrounding this technology necessitates a thoughtful examination of how these tools are implemented, providing insight into the responsibilities of scientists. In this section, we will discuss the ethical implications, focusing specifically on in vivo applications and the regulatory frameworks that guide their use.
Ethics of In Vivo Applications
In vivo applications of voltage-sensitive dyes raise numerous ethical concerns. Firstly, one must consider the welfare of animal models in which these dyes are tested. The application of VSDs during research can cause stress or suffering to the animals involved. It is essential to ensure that such studies comply with ethical standards designed to minimize discomfort. Researchers have to justify the benefits of their findings against the potential harms inflicted on animal models. This balance is critical to uphold the integrity of scientific research.
Secondly, there are questions related to the long-term effects of VSDs when used in living organisms. The impact of these dyes on biological systems over time has not been thoroughly explored, raising concerns about unintended consequences. Researchers must engage in thorough risk assessments and follow ethical guidelines that emphasize the need for careful monitoring of any adverse effects that may arise from these applications.
Finally, transparency in reporting results and methodologies is a key ethical consideration. Researchers need to communicate findings regarding the efficacy and safety of VSDs to the public in a clear and honest manner. This transparency helps maintain public trust in scientific research and ensures that ethical standards are upheld throughout the research process.
Regulatory Framework
The regulatory framework surrounding voltage-sensitive dyes is vital for ensuring ethical standards are met in their application. Various institutions and governing bodies have established guidelines that researchers must adhere to when conducting studies involving these dyes. The aim of such regulations is to protect both the subjects involved and the integrity of the research itself.
In many countries, researchers are required to obtain approval from institutional review boards (IRBs) before conducting any studies involving in vivo applications of VSDs. These boards review proposed research projects for ethical compliance and provide oversight to ensure that the potential benefits justify the risks involved. It is pivotal that researchers familiarize themselves with these regulations, as failing to comply may result in significant repercussions, including loss of funding or retraction of published studies.
Furthermore, researchers must stay updated about evolving regulatory requirements as new information emerges regarding the safety and effectiveness of voltage-sensitive dyes. The dynamic nature of both scientific advancement and regulatory policies illustrates the importance of adaptability and continuous education within the scientific community.
"An ethical approach to research fosters trust and integrity in scientific exploration, paving the way for innovative breakthroughs while ensuring accountability."
The combination of ethical considerations and robust regulatory frameworks ensures that the use of voltage-sensitive dyes aligns with societal values and scientific rigor. Researchers in the field must remain acutely aware of their ethical responsibilities as they navigate complex challenges posed by the applications of VSDs.
The End
The conclusion of this article underscores the significance of voltage-sensitive dyes in modern scientific research and medical applications. The exploration of this topic reveals a complex interplay between molecular biology, imaging techniques, and technological advancements. This synthesis not only highlights the considerable progress made with voltage-sensitive dyes but also points to relevant considerations regarding their future development and ethical usage.
Summary of Key Points
- Voltage-sensitive dyes are crucial tools for studying electrical activities in cells, especially in neurons and cardiac tissues.
- Their chemical action depends on the molecular structure, allowing real-time imaging of voltage changes.
- Applications extend to neuroscience and cardiology, offering insights into network dynamics and arrhythmia.
- Despite their advantages, challenges such as phototoxicity and signal-to-noise ratio persist.
Research advances, such as time-resolved imaging and multi-photon microscopy, have further enhanced their utility.
- Ethical considerations, particularly in vivo applications, warrant careful attention to ensure responsible research practices.
Implications for Future Research
Looking ahead, the trajectory of voltage-sensitive dyes appears promising, with numerous opportunities for innovation. Key areas for future research include:
- Next-generation dyes: Development of dyes with improved stability and sensitivity will likely facilitate more accurate measurements of cellular activity.
- Integration with technologies: Combining voltage-sensitive dyes with advanced imaging techniques can unveil more comprehensive insights into biological systems.
- Translational research: The potential for applying findings from voltage imaging to clinical settings can influence patient care strategies profoundly.
The importance of continual evaluation of ethical frameworks in tandem with scientific progress cannot be overstated. Ensuring clear guidelines protects both research subjects and the integrity of the scientific community, fostering trust and safety.
In summary, voltage-sensitive dyes represent a critical component in the advancement of biological research, revealing complex cellular mechanisms and holding promise for future innovations that could reshape our understanding of physiology.