High Resolution Melting Analysis in Molecular Biology
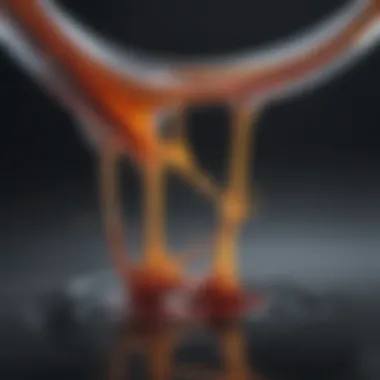
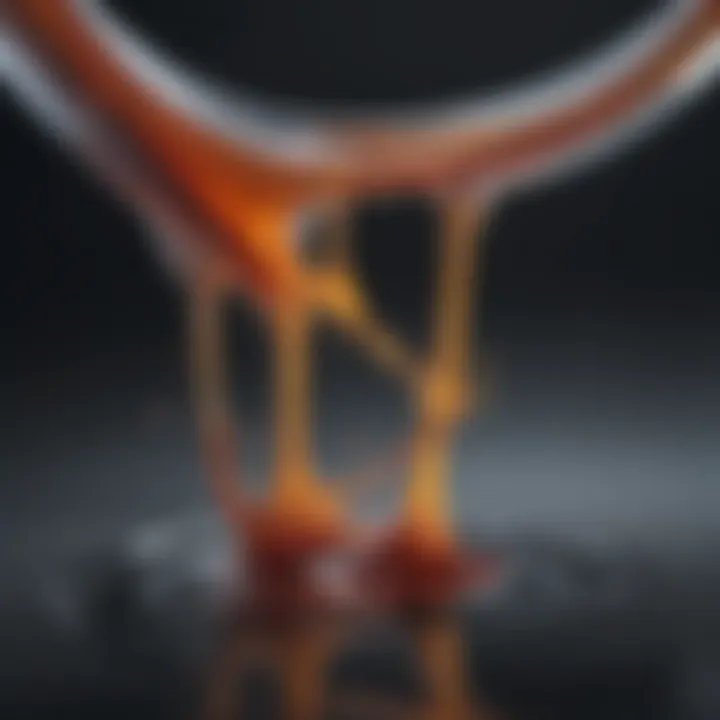
Intro
High-resolution melting (HRM) analysis has rapidly gained traction as a vital tool in molecular biology due to its unique ability to identify genetic variations. At its core, HRM is a derivative of traditional melting curve analysis but brings the precision and accuracy necessary for detecting subtle differences in DNA sequences. This technique tracks the changes in fluorescence as DNA duplex disassociates into its single-stranded forms when subjected to increasing temperatures. Essentially, it nails down even the most minute polymorphisms, making it indispensable for researchers studying genetic diversity, disease markers, and evolutionary biology.
The technique's charm lies in its simplicity and efficiency. HRM facilitates a straightforward experiment wherein accurate results emerge without the need for extensive post-PCR processing. This has broad implications, especially for faster diagnostics in clinical and research settings. As we delve deeper, itās essential to grasp not only the mechanisms at play but also the methodologies and applications that make HRM a frontrunner in the field of genetics.
Preface to High Resolution Melting Analysis
High Resolution Melting (HRM) analysis has emerged as a significant tool in molecular biology, offering precise and efficient means of examining DNA variations. Understanding HRM is essential for students, researchers, and professionals aiming to harness its dimensions in genetic research and diagnostics. This section serves as a gateway to comprehending the foundation of HRM. It not only underscores the importance of this method but also sets the stage for exploring its principles and applications in detail.
Definition and Importance
HRM analysis is a technique that focuses on the melting behavior of DNA. Essentially, it allows scientists to detect variations in the genetic material after PCR amplification. During the melting process, double-stranded DNA separates into single strands, and the temperature at which this occurs is influenced by the sequence and structure of the DNA. Thus, the precise monitoring of these melting curves using specialized instruments reveals subtle differences, making it invaluable for identifying genetic polymorphisms.
The importance of HRM lies in several key aspects:
- Precision: It enables a high degree of accuracy in detecting even minor genetic changes that could have significant biological implications.
- Efficiency: Compared to traditional methods, HRM is quicker, allowing for a faster turnaround in research and clinical settings.
- Cost-effectiveness: Once the infrastructure is in place, analyzing samples through HRM is often more economical than other rigorous techniques such as Sanger sequencing.
"HRM analysis has the potential to revolutionize how we identify and understand genetic variations, bridging gaps in diagnostics and research."
Understanding these facets helps underline HRM's pivotal role in not just academic settings but also in practical applications such as clinical diagnostics, thus solidifying its place in the future of genetics.
Historical Context
The journey of High Resolution Melting analysis began in the late 1990s, evolving alongside advancements in molecular biology. Initially, DNA melting analysis was a rudimentary process relying heavily on observational methods. Scientists faced limitations in resolution and sensitivity, making in-depth analysis challenging.
The introduction of real-time PCR changed the game. This breakthrough allowed for the simultaneous amplification and analysis of DNA, laying the groundwork for what would become HRM. In the early 2000s, researchers began to appreciate how improved thermodynamic models could enhance melting curve analysis, leading to more streamlined processes.
Over time, technological advancements have only broadened the scope of HRM. Sophisticated instruments capable of high-resolution real-time data acquisition were developed, enabling researchers to visualize melting curves with unprecedented clarity. This shift has broadened HRM's applicability in various fields, including genetics, forensics, and pathogen detection.
The evolution and growing significance of HRM highlight the field's dynamism and its continuing relevance in addressing complex biological questions. As methodologies improve, HRM stands as a fruitful area of exploration, melding traditional concepts with groundbreaking technologies.
Principle of High Resolution Melting
Understanding the principles behind high resolution melting (HRM) is key to appreciating its role in the assessment of genetic variations. HRM takes advantage of the unique melting properties of DNA to identify even the most subtle differences between genetic sequences. This principle not only allows biologists to detect mutations but also to monitor changes that could have significant implications in genetic research, diagnostics, and even personalized medicine. In order to harness the full potential of HRM, one must grasp the interplay between DNA melting and thermodynamic principles.
DNA Melting and Thermodynamics
When discussing DNA, people often refer to the melting temperature, known as Tm. This is the temperature at which half of the DNA strands are in the double helix state and the other half are in the "melted" single-stranded state. The melting curve, obtained during HRM analysis, provides valuable insights into the stability of DNA fragments under varying conditions.
At a basic level, the stability of a DNA molecule hinges on factors such as its base composition, length, and the presence of mismatches. For instance, GC-rich regions, which contain guanine-cytosine pairs, generally exhibit higher melting temperatures than AT-rich regions due to the three hydrogen bonds formed between guanine and cytosine. Hence, the thermodynamic properties govern the reliability of the melting point identification in HRM.
Several interpretations can be attained from the melting curve:
- Shape of the curve: Abnormalities in the curve may indicate genetic variations or the presence of mutations.
- Position of the curve: Significant shifts may correspond to differences in the sequence, leading to diagnostic conclusions.
Such thermodynamic factors must not be taken lightly, as they shape the correctness of results produced and ultimately feed into the larger interpretations that researchers draw from the data.
Detection Technologies
Moving onto the technologies that allow for HRM analysis, numerous tools exist to capture the dynamics of DNA melting. These technologies not only enable precise measurements but also enhance the overall efficiency of the analysis process.
- Real-Time PCR Systems: These systems allow for the monitoring of fluorescence during PCR amplification. The incorporation of specific dyes that intercalate into double-stranded DNA is crucial, as this takes advantage of the changes in fluorescence signal during melting. Common dyes include SYTO 9 and EvaGreen.
- Fluorescent Probes: Some HRM applications use specific probes that detect melting in a different manner. These can provide real-time data about DNA transitions, offering another layer of detail during analysis.
- Software and Algorithms: Advanced analytical software can interpret data from HRM devices, allowing for comparative analyses that are critical when distinguishing between subtly different DNA strands. This opens new avenues for researchers looking to fine-tune their methodologies.
In sum, the principle of high resolution melting can be seen as a balance of thermodynamics and innovative detection technologies. Understanding these concepts lays the groundwork for successful HRM implementation and its advancement in molecular biology.
Methodology of HRM Analysis
High Resolution Melting (HRM) analysis has emerged as a vital technique in molecular genetics, enabling researchers to delve deep into the subtleties of DNA. The methodology behind HRM is not only important; it's pivotal in ensuring accurate identification of genetic variations. Understanding this methodology begins with a well-thought-out approach to sample preparation through to PCR amplification and the execution of the HRM procedure itself. Each element infuses the process with specificity and nuance, shedding light on previously opaque areas of molecular biology.
Sample Preparation Protocols
In any laboratory, the saying goes, "a chain is only as strong as its weakest link," which rings true for HRM analysis. A precise sample preparation protocol sets the stage for success. This step involves careful extraction and purification of DNA from the biological material. The quality of the extracted DNA plays a crucial role in the results obtained from subsequent processes. If the DNA carries contaminants, it may hinder amplification or introduce noise in the melting curves.
The protocols often include steps like:
- Lysis of Cells: Using buffers or enzymes to break down cell membranes.
- Precipitation: Employing alcohol or salt solutions to condense DNA.
- Purification: Utilizing column-based or magnetic bead methods to clean up the DNA.
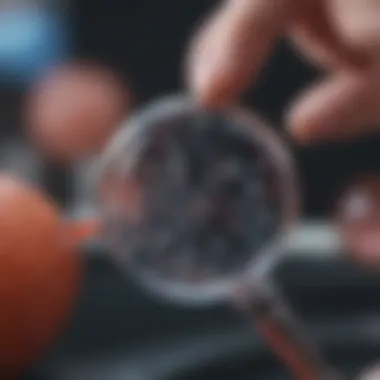
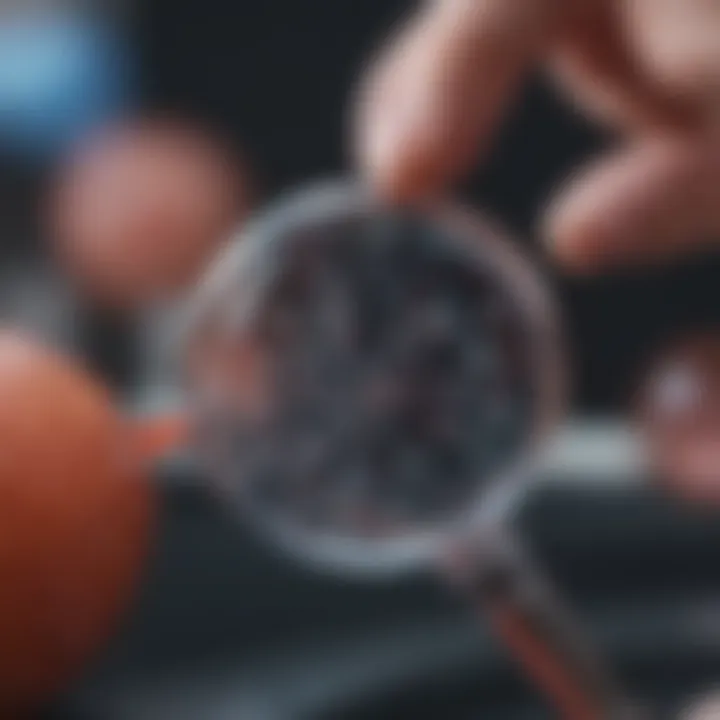
Adequate quantification of the DNA concentration can alsoāt be overlooked, as this ensures that optimal amounts are used in the PCR, thus preventing skewed results.
PCR Amplification Techniques
Once the samples are ready, PCR amplification becomes the next crucial piece in the puzzle of HRM analysis. Polymerase Chain Reaction is the method that amplifies the target DNA, making it detectable through HRM. It's here where the art of temperature cycling comes in playādenaturation, annealing, and extension steps are carefully orchestrated to generate high yields of the desired DNA fragments.
Key considerations in PCR include:
- Choice of Primers: Designers should ensure that primers are specific to the target sequence, reducing the chance of non-specific amplification.
- Optimization of Cycling Conditions: These conditions must be rigorously tested for each new template, including temperatures and timeframes.
- Contamination Prevention: Avoiding cross-contamination by using separate work areas and equipment for PCR setup and handling post-PCR samples is imperative.
Each of these steps influences the efficiency and specificity of the amplification, ultimately leading to more reliable HRM results.
High Resolution Melting Procedure
Now, letās get to the heart of HRM analysis ā the melting procedure itself. Itās during this phase that the magic happens. After PCR amplification, the DNA samples are subjected to gradual heating while monitoring the fluorescence generated by double-stranded DNA-binding dyes. As the temperature increases, the DNA starts to denature ā forming single strands, which corresponds to a specific melting temperature (Tm).
The typical steps in the melting procedure involve:
- Incremental Heating: The samples are gradually heated, often within a range of 60°C to 95°C.
- Fluorescence Monitoring: Real-time monitoring of fluorescence signals provides critical data.
- Data Collection and Analysis: The resulting melting curves are analyzed to identify differences in Tm between samples.
Each curve tells a story. A slight variation in melting behavior may signify a genetic polymorphism or an atypical mutation. Itās through interpretation of these curves that the true potential of HRM analysis comes to light.
"In the intricate dance of DNA, every change in temperature plays a note, revealing the symphony of lifeās genetic code."
Through an understanding of the HRM methodology, researchers can enhance their ability to detect genetic variations precisely and efficiently. This chapter not only illustrates how to embark on HRM analysis but also underscores its relevance in advancing genetic research today.
Applications of HRM Analysis
High Resolution Melting (HRM) analysis is not merely a theoretical pursuit; its applications resonate across various fields, making it a vital tool in modern genetics. This section will explore how HRM is leveraged in different practical contexts, highlighting its significance in detecting genetic variations, screening for mutations, identifying pathogens, and ensuring quality control in genetic research and biotechnology. Each of these applications emphasizes the versatility and precision of HRM, which translates to considerable benefits in both research and clinical settings.
Genetic Variation Detection
Detecting genetic variations is at the heart of HRM analysis. The technique enables researchers to discern single nucleotide polymorphisms (SNPs) with remarkable sensitivity. By closely examining the melting curves of DNA, one can identify these variations that might be associated with specific traits or diseases. This capability is essential for population genetics and evolutionary studies, as it helps track allele frequency changes over time.
For instance, consider a study focused on a particular genetic marker linked to a hereditary condition. HRM can effectively reveal changes in the DNA sequence that could contribute to the risk of developing this condition in certain populations. The precise measurement of these variations facilitates a deeper understanding of genetic predispositions and can guide targeted interventions.
Mutation Screening
Mutation screening is another critical application of HRM analysis. The ability to detect mutations rapidly and accurately makes it invaluable in both clinical diagnostics and research. For example, in oncology, oncologists can employ HRM to identify mutations in cancer-related genes, allowing for tailored therapies based on the specific mutation profile of a tumor.
HRM serves as a frontline technique after PCR amplification to identify mutations that may alter the protein's coding sequence. For cases where conventional sequencing methods could be time-consuming and cumbersome, HRM offers a rapid alternative. Its robustness in identifying even subtle changes (like point mutations) means that it can play a crucial role in early cancer detection and prognostication, enriching the overall understanding of tumor biology.
Pathogen Identification
When it comes to infectious diseases, HRM analysis proves to be a game changer. The method can be employed for the identification of pathogens with notable efficacy. By analyzing the melting behavior of pathogen-specific DNA, researchers can distinguish between strains of a pathogen, a particularly useful feature for tracking outbreaks and determining infection sources.
For example, in an outbreak of a viral disease, HRM can help identify the responsible strain quickly. Using specific primers, the melting curves can reveal minute differences in the viral genome, allowing health authorities to respond promptly and efficiently. This swift identification has implications not just for treatment but also for controlling the spread of infections in public health settings.
Quality Control in Genetics
Ensuring quality control in genetics is paramount in both research and application. HRM analysis contributes significantly to the integrity of genetic testing and research protocols. By serving as a validation tool for PCR products, HRM can confirm that the amplified regions are free from contamination and that the assays are functioning appropriately.
Employing HRM for quality control involves comparing the melting profiles of experimental samples against control samples. This ensures consistency and reliability within experiments, leading to more trustworthy results. Such rigorous quality assurance processes are essential in clinical settings where genetic tests guide treatment decisions.
"The integration of HRM analysis in quality control protocols not only enhances the reliability of genetic results but also boosts the confidence of clinicians and researchers alike."
Ultimately, the applications of HRM analysis extend beyond academic curiosity; they hold concrete implications in healthcare, research, and biotechnology. The techniqueās ability to uncover genetic variations, screen for mutations, identify pathogens, and enforce quality control makes it a cornerstone of modern molecular biology.
Interpreting HRM Data
Understanding how to interpret HRM data is crucial for unraveling the complexities of genetic analysis. High Resolution Melting (HRM) has gained traction due to its ability to detect variations in DNA with a remarkable level of precision. However, the value of HRM lies not just in the technology itself, but in how well researchers can analyze the outcome of their experiments. Without the ability to interpret melting curves effectively, the potential of HRM could be severely diminished.
Melting Curve Analysis
Melting curve analysis is the heart of interpreting HRM data. Each DNA variant exhibits a unique melting curve during the HRM process, which arises when the double-stranded DNA denatures into single strands. This denaturation occurs at a specific temperature, which varies depending on the DNA sequence's composition. Variations, such as single nucleotide polymorphisms (SNPs), can shift the melting temperature, thereby creating a distinct melting curve for each variant.
A well-defined melting curve can reveal crucial information:
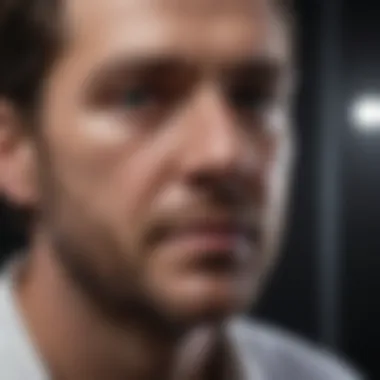
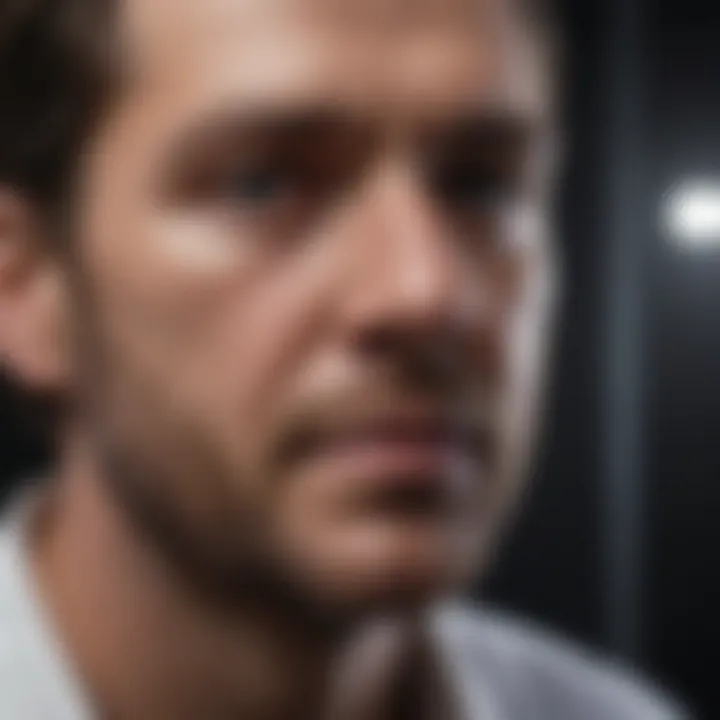
- Shape and Peak Location: The shape of the melting curve can indicate the presence of specific polymorphisms. A sharp peak typically corresponds to homogenous DNA, while broader peaks might suggest the presence of variants or heterogeneity.
- Temperature Range: Analyzing the temperature range over which melting occurs can help identify the exact nature of the genetic variant. For example, different temperatures might infer the degree of GC content in the DNA, which correlates with stability under heat.
In executing melting curve analysis, it is important to employ software tools that can offer an accurate readout of these curves. Tools can analyze the data to spot shifts and discrepancies, thus enhancing the reliability of results. Furthermore, visualization of the results can be crucial, as graphical representations often lend themselves to clearer insights than numerical data alone.
Comparative Analysis Techniques
Comparative analysis techniques form a backbone for validating HRM data interpretations. These approaches allow researchers to contrast their findings against known standards or controls. Here are some techniques that are commonly used:
- Standard Reference Comparison: This involves comparing melting curves from test samples against those from standards. Such a practice not only bolsters reliability but can also enhance the sensitivity and specificity of results.
- Cluster Analysis: By grouping similar melting curves, cluster analysis can simplify the interpretation of HRM data. It provides a visual guideline and empowers the researcher to identify distinct genetic populations.
- Machine Learning Approaches: Emerging technologies apply machine learning algorithms to analyze melting curves. This innovative approach processes large datasets more efficiently than traditional methods and could unveil subtle differences that might otherwise go unnoticed.
- Standard Deviation Evaluation: By assessing the variability in melting temperature and shape within replicates, researchers can gauge the consistency of their data. High standard deviations may signify potential issues in sample preparation or amplification.
Overall, the fusion of melting curve analysis with comparative techniques enhances the robustness of HRM data interpretation. Ultimately, the ability to discern these subtleties not only enriches scientific understanding but drives further advancements in genetic research.
"The true power of genomics lies in the interpretation and application of data; melting curves are our insights into the world of genetics."
Interpreting HRM data can be likened to piecing together a puzzle, where each melting curve reveals a fragment of the bigger picture. As researchers hone their skills in this area, the potential for breakthroughs in genetic understanding expands exponentially.
Advantages of HRM Analysis
The landscape of molecular biology continually evolves, and within this realm, High Resolution Melting (HRM) Analysis emerges as a technique not just relevant but transformative. Understanding its advantages is pivotal for anyone dabbling in genetic analysis today. Its role is particularly significant when evaluating genetic variation, which is central to numerous applications ranging from clinical diagnostics to genetic research. Here, we dig into two primary facets: speed and efficiency, alongside high sensitivity and specificity.
Speed and Efficiency
Time is of the essence in laboratories, where every minute can mean the difference between successful outcomes and missed opportunities. One of the hallmarks of HRM analysis lies in its remarkable speed. Unlike traditional methods such as sequencing or extensive gel electrophoresis, HRM provides rapid results. Typically, the entire processāfrom sample preparation through to analysisācan often be condensed into mere hours. This offers researchers a timely way to get answers and pivot their strategies accordingly.
Moreover, consider the automation possibilities with HRM. Many instruments are designed to handle multiple samples simultaneously, streamlining workflows. This reduces manual labor and the potential for human error, giving researchers more time to focus on critical interpretations rather than labor-intensive processes.
- Rapid DNA quantification of multiple samples
- Cuts down turnaround time significantly
- Minimal intervention required due to automation
HRM analysis allows laboratories to operate at a pace that meets the demanding nature of modern research and clinical environments. This efficiency does not merely save timeāit translates into cost reductions and optimized resource allocation. A laboratory that can perform its analyses swiftly doesnāt just increase productivity; it enhances its capacity to contribute to ongoing projects and research initiatives.
High Sensitivity and Specificity
When it comes to detecting genetic variations, precision is essential. HRM is particularly valued for its high sensitivity and specificityāattributes that are vital when researchers are identifying subtle differences in DNA sequences. The technique employs a stringent melting curve analysis, which can distinguish even slight discrepancies in genetic material.
- Sensitivity: HRM is capable of detecting low-frequency variants that might go unnoticed using less sensitive methods. This makes it invaluable in scenarios like early cancer detection, where mutations could exist in minuscule quantities.
- Specificity: The technique excels at differentiating between closely related sequences. This specificity minimizes false positives, ensuring that results reflect true genetic variations.
With HRM, the stakes are often high; a misinterpretation can have serious implications in clinical settings, particularly in diagnostics and treatment plans. The technology's uncanny ability to maintain accuracy is what makes it a preferred choice in diverse applications, from research laboratories to clinical settings.
"High Resolution Melting Analysis stands as a benchmark for reliable genetic testing, bridging the gap between the known and the unknown in molecular diagnostics."
As we consider the merging demands of research integrity and operational efficiency, HRM analysis undoubtedly proves itself a formidable tool in the geneticist's arsenal. The fusion of speed, sensitivity, and specificity facilitates a robust framework for investigating the complexities of genetic variation. This position in molecular biology is not merely a matter of convenience; it is central to accurate diagnoses and the development of innovative treatments.
Challenges and Limitations
High Resolution Melting (HRM) analysis presents an innovative frontier in molecular biology. Yet, like any tool in a scientistās kit, it comes with its share of challenges and limitations. Understanding these challenges is essential for anyone who aims to leverage HRM effectively in their research or clinical applications. Itās not just about harnessing the power of the technique but also recognizing its boundaries.
Technical Difficulties
HRM analysis is not without its technical hurdles. Several factors can pose significant obstacles during experimental procedures.
First, thereās the quality of the sample. DNA samples need to be pure, intact, and free from contaminants. If a sample is degraded or contaminated, it may produce misleading results, leading researchers down a rabbit hole of erroneous interpretations. Moreover, PCR conditions must be meticulously optimized. Even the slightest deviation in temperature or timing can affect the amplification of the DNA, which, in turn, alters the melting curve analysis.
Another technical aspect involves instrumentation. The precision and sophistication of the HRM equipment play a pivotal role. Some instruments may have limitations in their resolution or sensitivity, which can compromise the accuracy of detecting subtle variations in melting temperatures. Settings often require careful calibrations, and operators need to be well-versed in the specifics of the machinery they are using.
Itās also essential to remember that not all fluorescent dyes perform equally well or are compatible with every type of fragment. Selecting an appropriate dye is crucial for achieving reliable results, contributing to the overall complexity of the procedure.
Data Interpretation Issues
Once the technical aspects have been addressed, data interpretation represents another realm of difficulty. Melting curve profiles can be challenging to analyze. Factors such as the presence of multiple variants and overlapping curves can make it tricky to distinguish between them. Often, researchers find themselves in a situation where visual cues are insufficient to make definitive conclusions about gene variations.
Furthermore, software tools utilized for analysis may vary widely in their capabilities and user-friendliness. While some algorithms provide advanced statistical analyses, others may be somewhat rudimentary and unable to meet the analysis needs of sophisticated experiments. As a consequence, a researcherās skill in navigating and understanding these tools becomes critical. Misinterpretation can lead to incorrect assumptions about genotypes, which can have significant downstream effects on studies.
To add another layer of complexity, biological variability itself can interfere with data interpretation. Variations in biological processes can result in unexpected melting patterns that could easily be mistaken for genetic variations. Thus, thorough knowledge of both the biological system being studied and the technical aspects of HRM is paramount to avoid missteps in data interpretation.
"Awareness of these challenges can significantly bridge the gap between potential and actual success in HRM analysis."
In summary, while HRM analysis is a powerful tool for genetic assessment, itās crucial to maintain a realistic perspective regarding its challenges. By understanding the technical difficulties and data interpretation issues that may arise, users can better prepare themselves to navigate the intricacies of HRM, leading to more reliable and informative results.
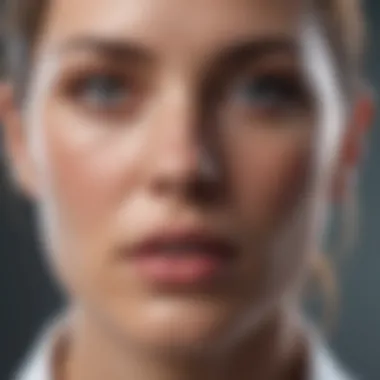
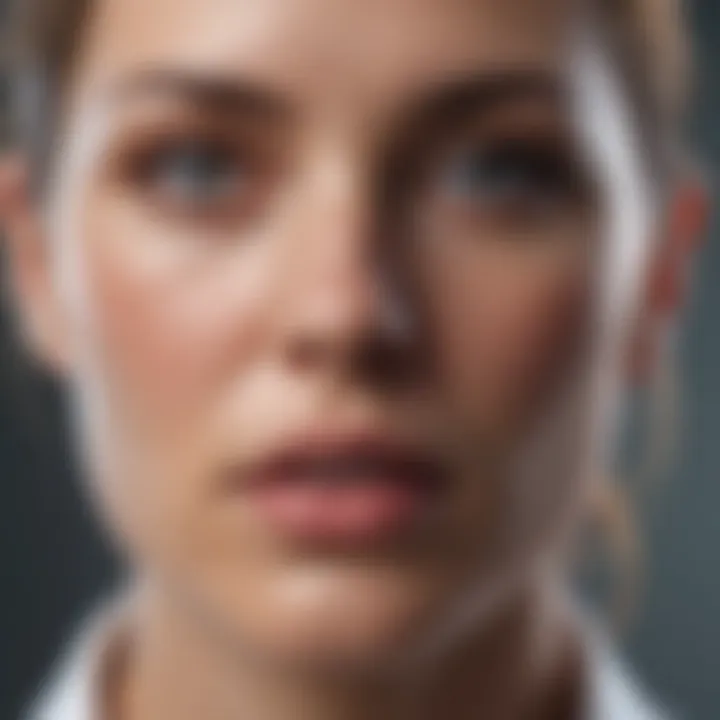
Future Directions in HRM Analysis
The exploration of future directions in High Resolution Melting (HRM) analysis is essential for harnessing its full potential in genetic research and diagnostics. As technology continues advancing, there's a palpable excitement surrounding the prospective applications that HRM can offer. This section highlights some of the key innovative hues of HRM and its integration with next-generation sequencing, showing how these pathways could reshape the landscape of molecular biology.
Innovative Applications in Research
One of the most exciting prospects of HRM analysis is its burgeoning role in research settings. Laboratories are not just viewing HRM as a mere adjunct to other molecular techniques but are now recognizing its intrinsic value for groundbreaking research.
For instance, HRM techniques have begun to find their footing in the world of epigenetics. Scientists are uncovering how DNA methylation patterns influence gene expressionāsomething traditional methods flounder with. With HRM, researchers can differentiate between methylated and unmethylated DNA templates efficiently. Additionally, the precision of this technique can aid in identifying alleles or variants that may convey different disease susceptibilities.
Here are some notable applications emerging in the field:
- Populational Genomics: By analyzing melting curves, researchers can track genetic diversity within populations. This understanding is vital for conservation efforts.
- Pharmacogenomics: HRM can help in profiling genetic variations that affect individual responses to drugs, thus tailoring personalized medicine.
- Microbial Ecology: This technique can assist in identifying and characterizing microbial strains in environmental samples, which is becoming increasingly pertinent in understanding ecosystem dynamics.
These innovative applications underscore the adaptability of HRM technology across diverse research landscapes, indicating a bright future ahead.
Integration with Next-Generation Sequencing
Another compelling future direction for HRM analysis lies in its synergistic integration with next-generation sequencing (NGS). As NGS technology has advanced, dropping costs and increased throughput have made it a go-to method for comprehensive genomic analysis. However, the data complexity often becomes a double-edged sword.
By combining HRM with NGS, researchers can tackle some of the shortcomings inherent in NGS data interpretation. After sequencing a target region, HRM can provide a rapid screening tool to confirm findings. This dual-layered approach ensures that any possible sequencing artifacts are quickly identified, enhancing reliability and confidence in results.
Moreover, the amalgamation helps refine existing workflows.
- Streamlined Confirmation: HRM can act as a preliminary confirmatory step before diving into deeper, more complex analyses.
- Cost Efficiency: Validate a narrowed-down selection of variants using HRM, minimizing the need for extensive follow-up sequencing.
- Enhanced Resolution: The ability of HRM to detect low-level variants or genetic heterogeneity provides added nuance to genomic datasets.
"By marrying HRM's precision with NGS's expansive reach, the bounds of genetic analysis could be pushed farther than ever before."
Envisioning a synergistic future is crucial for maximizing the benefits of both technologies, allowing for a more nuanced understanding of genetic variations and their implications. This integration could very well open the floodgates to advancements that were previously unimagined.
In summary, the future of HRM analysis presents remarkable possibilities within both academic and practical applications. As we continue to hone these methodologies and explore their boundaries, the potential contributions to genomics, diagnostics, and beyond will be substantial.
Case Studies and Real-World Applications
High Resolution Melting (HRM) analysis has carved out a substantial niche in both clinical and agricultural settings. The breadth of its applications showcases how this technique can illuminate genetic nuances, providing valuable insights that drive advancements in health and environmental sustainability. Case studies in these areas are crucial, as they not only demonstrate the practical utility of HRM but also highlight the challenges faced by practitioners and researchers in real-world scenarios.
Clinical Diagnostics
In the landscape of clinical diagnostics, HRM plays a pivotal role. With the ability to detect subtle changes in DNA sequences, it is particularly useful in identifying genetic mutations linked to various diseases. For instance, in the realm of oncology, HRM has been employed to screen for mutations in cancer-related genes such as TP53 and KRAS. These mutations are often harbingers of disease progression, and their early detection can significantly influence treatment decisions.
A noteworthy case involves the application of HRM in the detection of hereditary breast and ovarian cancer syndrome (HBOC). By analyzing melting curves of BRCA1 and BRCA2 genes, researchers successfully identified known mutations, aiding in risk assessment for patients. This approach not only enhances the speed of genetic testing but also reduces costs compared to traditional methods.
"HRM provides a rapid, cost-effective tool for detecting genetic variations linked to hereditary cancer syndromes, streamlining patient management."
In addition to cancer, HRM is used for diagnosing infectious diseases. For example, it has been applied to identify strains of Mycobacterium tuberculosis with varying drug resistance phenotypes. This is vital in public health management, as rapid identification of resistant strains can effectively guide treatment strategies.
Agricultural Biotechnology
Agricultural biotechnology stands as another prominent arena where HRM analysis has made its mark. The world of crop improvement relies heavily on understanding genetic diversity and variation. HRM provides a means to assess polymorphisms among crop varieties rapidly. This has enormous implications in breeding programs aimed at enhancing traits like yield, disease resistance, and environmental resilience.
A practical instance is found in the study of Zea mays, commonly known as maize. Researchers have utilized HRM to identify genetic variations associated with drought tolerance. By comparing melting curves from different maize lines, significant polymorphisms were detected, paving the way for the selection of superior traits in breeding programs.
Moreover, HRM has also been valuable in the certification of genetically modified organisms (GMOs). By detecting specific DNA sequences associated with GM traits, it enables compliance with regulatory requirements while ensuring that labeling standards are met. This practice can minimize consumer concerns, as the transparency surrounding GMO presence in food products becomes more achievable.
In closing, the case studies surrounding HRM in clinical diagnostics and agricultural biotechnology reveal a technique that is not just academically interesting, but practically vital. Each application illustrates the method's benefits and offers a roadmap for addressing challenges found in field settings. As research continues to expand this methodologyās boundaries, both human health and food security can only stand to gain.
Culmination
High Resolution Melting (HRM) analysis plays a pivotal role in modern molecular biology, providing researchers and clinical practitioners with a robust methodology for exploring genetic variations. Through distinct and precise melting curve profiles, HRM readily identifies even subtle differences in DNA sequences. The importance of this conclusion lies in its synthesis of the previously discussed elements, particularly the high sensitivity and specificity that HRM delivers. This technique transcends basic genetic analysis, offering a pathway to greater insights into heredity, disease susceptibility, and therapeutic targets.
Summary of Findings
As we dissected throughout this article, several key takeaways underscore the significance of HRM:
- Versatile Applications: HRM's usage extended from genetic variation detection to its role in pathogen identification and clinical diagnostics, emphasizing its importance across various domains.
- Robust Methodology: The quality of the HRM methodology, from sample preparation to data interpretation, supports reliable outcomes, yet does not compromise on speed and efficiency.
- Revolutionary Potential: Future integrations of HRM with next-generation sequencing technologies promise to widen the horizons of genetic research, potentially revolutionizing our approach toward understanding complex genetic traits.
Furthermore,
"the efficient analysis of melting curves can be transformative in the early detection of diseases, aiding in timely clinical decisions."
Final Thoughts on HRM Methodology
HRM methodology embodies a cutting-edge stride in genetic analysis, combining simplicity with high throughput capabilities. As researchers, educators, and professionals venture into the future of genetics, considering HRM's methodology means embracing a technique that not only adapts but also evolves in its applications. The methodological transition from traditional approaches to HRM lays down a substantial groundwork for innovative research breakthroughs. Fostering a deeper appreciation for HRM will drive its integration into more complex genetic models, enhancing the realm of personalized medicine and beyond.