Exploring Hydrogen Storage Methods: A Detailed Guide
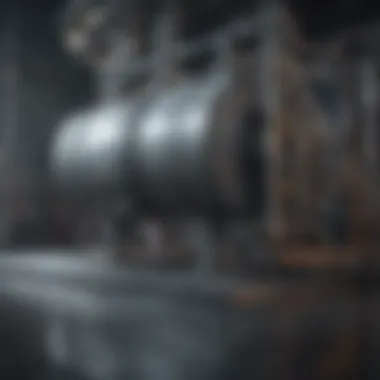
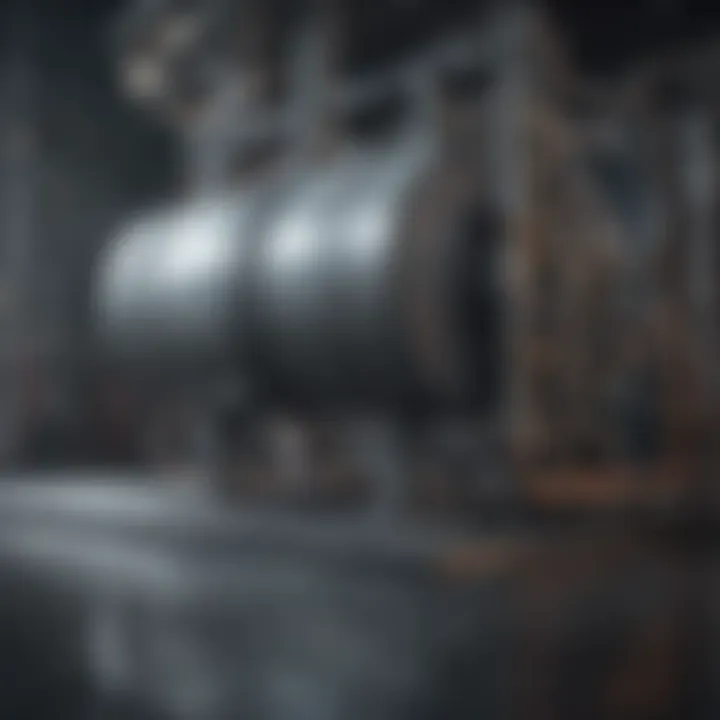
Intro
Hydrogen as an energy carrier has gained considerable attention in recent years. Its potential as a clean fuel supports a shift towards sustainable energy systems. However, storing hydrogen effectively presents a significant challenge. Given hydrogen's low density, traditional storage methods often face limitations. Therefore, exploring various hydrogen storage techniques is imperative for optimizing its use in energy applications.
This section provides a foundational overview of current methods for hydrogen storage, emphasizing both the advantages and pitfalls of each approach. The diversity in techniques reflects the complexity of hydrogen as a fuel source, allowing stakeholders to make informed decisions based on their specific operational requirements and conditions.
Research Overview
Summary of key findings
Numerous studies reveal that hydrogen can be stored through various methods, including compressed gas storage, liquid hydrogen, metal hydrides, and chemical hydrogen storage. Each method has distinct characteristics.
- Compressed Gas Storage: This is the most common method, using high-pressure tanks to hold gaseous hydrogen. It operates typically at pressures around 350–700 bar. Its simplicity and established technology make it favorable for many applications.
- Liquid Hydrogen Storage: Hydrogen can be stored in liquid form at extremely low temperatures, offering a higher density than gaseous storage. However, this method requires significant energy for liquefaction and maintenance of low temperatures.
- Metal Hydrides: These materials can absorb hydrogen, forming solid compounds. They offer higher volumetric storage density but often need high temperatures to release the hydrogen, which could limit their practical use in some scenarios.
- Chemical Hydrogen Storage: This innovative approach involves chemical reactions to store hydrogen. It can provide high energy density and safety, although the complexity of the dehydrogenation process poses challenges.
Importance of the research in its respective field
As the world transitions to more sustainable energy solutions, understanding the nuances of hydrogen storage is critical. This research informs stakeholders about the trade-offs between each method, optimizing the use of hydrogen in a broader energy context. By addressing these challenges, the study not only contributes to academic discourse but also paves the way for future technological advancements in hydrogen storage.
Methodology
Description of the experimental or analytical methods used
The research employs a comparative analysis to explore the effectiveness of each hydrogen storage method. Data is collected from various scientific journals and credible industry reports to assess storage capacity, safety, costs, and energy efficiency. Laboratory experiments further support findings regarding performance under different conditions.
Sampling criteria and data collection techniques
Selected studies are chosen based on their relevance to contemporary hydrogen storage technologies. Criteria include publication date, the scope of research, and the applicability of results to real-world scenarios. Data collection involves reviewing peer-reviewed articles and regulatory publications, ensuring a well-rounded examination of the topic.
"Efficient hydrogen storage solutions are key to advancing hydrogen as a viable energy carrier in the global context."
In summary, the scope of this article encompasses various hydrogen storage methods. By understanding the benefits and challenges associated with each, the groundwork for potential innovations in hydrogen technology can be established.
Prologue to Hydrogen Storage
The growing interest in hydrogen as a sustainable energy source has brought forth the critical need for efficient hydrogen storage solutions. As hydrogen holds great promise as an energy carrier, examining its storage methods gives us insights into its potential role in modern energy systems. This section aims to elucidate the fundamental importance and challenges surrounding hydrogen storage, setting the stage for understanding various storage methods.
Importance of Hydrogen as an Energy Carrier
Hydrogen is increasingly recognized for its potential in transforming energy sectors. It offers several key benefits that make it an attractive energy carrier:
- High energy density: Hydrogen has a higher energy content per unit mass than conventional fossil fuels, making it an efficient option for energy transport.
- Clean combustion: When used in fuel cells or combusted, hydrogen produces only water vapor as a byproduct, presenting an environmentally friendly alternative.
- Versatile applications: Hydrogen can be utilized in multiple sectors, including transportation, power generation, and industrial processes.
The rise of renewable energy generation has further amplified the demand for effective hydrogen storage technologies. Hydrogen can be produced through renewable means, such as electrolysis of water, especially when excess energy from sources like wind and solar goes unused. By efficiently storing this hydrogen, it can be released when needed, enabling a more sustainable energy network.
Key Challenges in Hydrogen Storage
Despite the advantages hydrogen presents, there are significant challenges corresponding to its storage:
- Physical properties: Hydrogen is a low-density gas at ambient conditions, necessitating high-pressure conditions or cryogenic temperatures for storage. This increases the complexity of storage solutions.
- Material compatibility: Hydrogen can cause embrittlement in certain metals, which complicates the design and choice of storage vessel materials.
- Energy efficiency: The processes involved in capturing, compressing, or liquefying hydrogen can lead to energy losses. Optimizing these processes is crucial to enhance overall efficiency.
- Safety concerns: Hydrogen is flammable and requires careful handling and storage to mitigate explosion risks.
Addressing these challenges is essential not only for the advancement of hydrogen technologies but also for integrating hydrogen into our existing energy systems.
Overview of Storage Methods
Understanding the various storage methods for hydrogen is essential in addressing how this versatile energy carrier can be effectively utilized. With the increasing importance of hydrogen in clean energy strategies, grasping the different techniques available helps to unlock its full potential. Each method has distinct characteristics, applications, and challenges, making it important for scientists, engineers, and policymakers to conduct a thorough evaluation when considering hydrogen storage solutions. This overview provides insight into the categorization and comparative analysis of these methods, laying the groundwork for deeper exploration in the subsequent sections.
Categorization of Storage Techniques
Hydrogen storage techniques can be categorized primarily based on their physical state and the methods used to store the hydrogen. This categorization helps identify which technique may be most suitable for specific applications. The main categories include:
- Compressed Gas Storage: This method involves storing hydrogen at high pressure in cylindrical tanks. It's a widely used technique and involves technologies that allow for safe handling of high-pressure gases.
- Liquid Hydrogen Storage: This technique involves cooling hydrogen to extremely low temperatures until it becomes a liquid. Liquid hydrogen has a higher density compared to gaseous hydrogen, making it advantageous for certain applications.
- Metal Hydride Storage: In this method, hydrogen is absorbed by certain metals allowing for storage at lower pressures and higher densities. This technique is notable for its compactness and safety.
- Chemical Hydrogen Storage: This involves storing hydrogen in the form of chemical compounds which can release hydrogen through chemical reactions. This method offers potential in long-term storage applications.
Each of these categories presents unique attributes, making it important to analyze them in context with intended use. For instance, compressed gas storage is commonly found in industrial applications, whereas liquid hydrogen may be more relevant in applications like space exploration where storage density is crucial.
Comparative Analysis of Storage Methods
When evaluating hydrogen storage methods, a comparative analysis sheds light on several critical factors:
- Energy Density: This is a crucial metric. Liquid hydrogen boasts a greater energy density than compressed and metal hydride storage.
- Cost Efficiency: The economics of each storage technique vary. For example, compressing hydrogen requires significant energy input, while chemical methods may involve complex processes but can also lead to high energy recovery potential.
- Safety: Safety concerns differ by method. Pressurized systems require robust safety measures to prevent leaks and bursts, whereas metal hydrides are generally safer but require considerations around material fatigue.
- Environmental Impact: Each method has different implications for environmental sustainability. Some processes may emit greenhouse gases depending on the source of energy used for hydrogen production.
Through this analytical lens, stakeholders can make informed decisions that align with energy requirements and sustainability goals.
Compressed Gas Storage
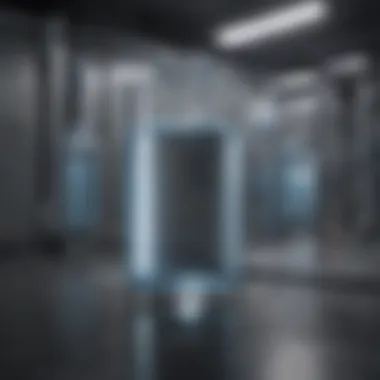
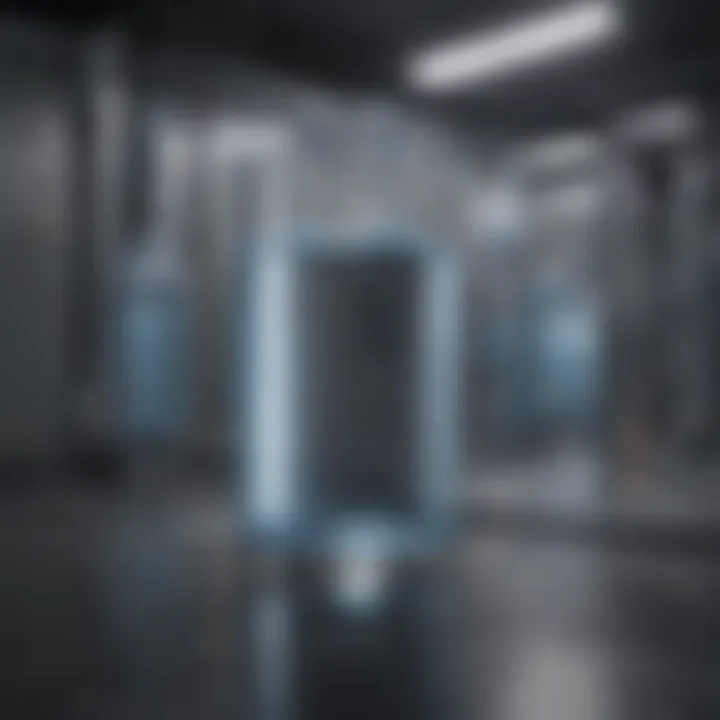
Compressed gas storage is a prominent method for storing hydrogen due to its practical benefits and established technology. This technique enables hydrogen to be stored at high pressures, typically ranging from 200 to 700 bar, which allows for efficient use of space. The significance of compressed gas storage lies not only in its physical benefits but also in its cost-effective nature. Implementing this method can facilitate hydrogen distribution for various applications such as fuel cell vehicles and industrial processes.
Process and Equipment
The process of compressing hydrogen gas involves utilizing a compressor, which reduces the volume and increases the pressure of the gas. Compressors vary in design, including reciprocating and rotary types, each chosen based on required efficiency and application needs. Once compressed, the hydrogen is stored in high-pressure tanks made of durable materials such as carbon fiber or steel. These tanks are designed to withstand the immense pressures and ensure safety during handling and transport.
There are several key components involved in the compressed gas storage system:
- Compressors: Devices that compress hydrogen to the desired pressure.
- Storage Tanks: High-pressure vessels that hold the compressed hydrogen.
- Pipelines: Tubes that facilitate the transfer of hydrogen between different points.
The efficiency of this storage method depends on the quality of equipment used and the pressure levels achieved during the compression process.
Safety Considerations
Safety considerations are paramount in compressed gas storage. The high pressures involved can pose risks, including leaks, ruptures, or even explosions. Rigorous adherence to safety protocols is essential.
- Regular Inspections: Compressed gas systems should undergo regular checks to ensure integrity and reliability.
- Pressure Relief Devices: These devices are installed to release excess pressure and prevent explosions.
- Training: Personnel handling hydrogen must be well-trained in safety procedures.
Maintaining proper venting, managing leaks promptly, and ensuring appropriate emergency procedures can substantially enhance safety during operation.
Applications in Industry
Compressed gas storage finds diverse applications across multiple industries. Its versatility and practicality make it an attractive option for businesses focused on maximizing efficiency in hydrogen use. Noteworthy applications include:
- Fuel Cell Vehicles: Many manufacturers employ compressed gas storage systems to power their hydrogen fuel cell vehicles, offering an efficient energy source.
- Industrial Hydrogen Supply: Industries requiring hydrogen for processes like refining or ammonia production benefit from the quick and easy availability of compressed hydrogen.
- Energy Storage Solutions: Compressed hydrogen serves as a form of energy storage that can balance supply and demand in renewable energy systems.
In summary, compressed gas storage is vital for hydrogen utilization across various sectors. It combines established technology with practical application, which is crucial for the growth of hydrogen as a sustainable energy source.
"Compressed gas storage remains a favored choice for hydrogen storage due to its long-standing reliability and cost-effectiveness."
Overall, understanding the ins and outs of compressed gas storage allows industry stakeholders to navigate the challenges and opportunities in hydrogen storage solutions.
Liquid Hydrogen Storage
Liquid hydrogen storage is a critical area of focus in hydrogen storage methods. It offers a way to manage hydrogen effectively for energy applications. Liquid hydrogen (L) is favored for its high energy density, which is essential for various modern applications ranging from aerospace to fuel cell vehicles. Storing hydrogen in liquid form allows for efficient transportation and usage in sectors where space and weight limitations are significant.
However, achieving and maintaining the necessary conditions for liquid hydrogen can be challenging. Analyzing the operational methods, design considerations, and associated benefits and hurdles of liquid hydrogen storage reveals significant insights about its role in the evolving energy landscape.
Cooling and Liquefaction Processes
The process of cooling and liquefaction is fundamental in converting gaseous hydrogen to its liquid state. This process typically involves lowering the temperature of hydrogen to below its boiling point of -252.87 °C under standard atmospheric pressure.
Several cooling methods, including Joule-Thomson expansion and self- refrigeration cycles, are employed to achieve these low temperatures.
- Joule-Thomson Expansion: This method exploits the expansion of hydrogen through a throttling valve, leading to a drop in temperature.
- Cryogenic Cooling: Liquid nitrogen or helium often assists in the cooling process, providing an effective heat exchange medium.
Maintaining hydrogen in a cryogenic state protects it from re-gasifying, ensuring that it can be stored efficiently. Proper monitoring of temperature and pressure is essential to ensure that the liquefaction process operates smoothly.
Container Design and Materials
Container design for liquid hydrogen storage is vital due to the unique properties of L. Containers must keep the hydrogen at extremely low temperatures while preventing heat transfer from the environment. The materials used must also withstand the low temperature without becoming brittle.
Typically, materials like stainless steel or specialized aluminum alloys are used. These materials have properties that allow them to retain structural integrity at cryogenic temperatures.
Some important design features include:
- Insulation: Multi-layer insulation is common to reduce heat transfer.
- Pressure Release Mechanisms: Containers should include failsafe systems to prevent overpressure scenarios, which can occur due to heat ingress or failure in cooling.
- Shape and Size Specifications: The shape impacts how the hydrogen behaves within the container and its efficiency in transportation.
Advantages and Limitations
Liquid hydrogen storage comes with distinct advantages and limitations, influencing its use in practical applications.
Advantages:
- High Energy Density: Liquid hydrogen provides a compact storage solution, allowing for greater energy content per unit volume compared to gaseous forms.
- Established Technology: The infrastructure for handling LNG and L is relatively well-developed, especially in industries like aerospace.
Limitations:
- Storage Challenges: The extreme low temperatures require sophisticated systems to maintain, increasing operational costs and complexity.
- Boil-off Losses: Hydrogen can vaporize and escape, leading to potential losses in storage efficiency.
Overall, liquid hydrogen storage presents a compelling option for energy storage, particularly where high energy density is critical. Understanding the challenges and benefits of liquid hydrogen is essential for advancing its application in a sustainable energy system.
Metal Hydride Storage
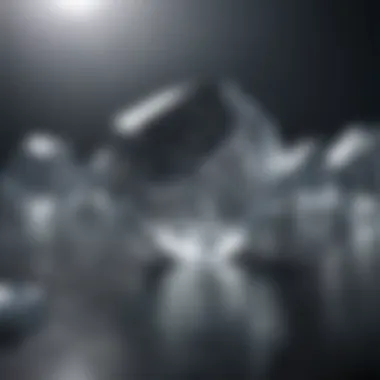
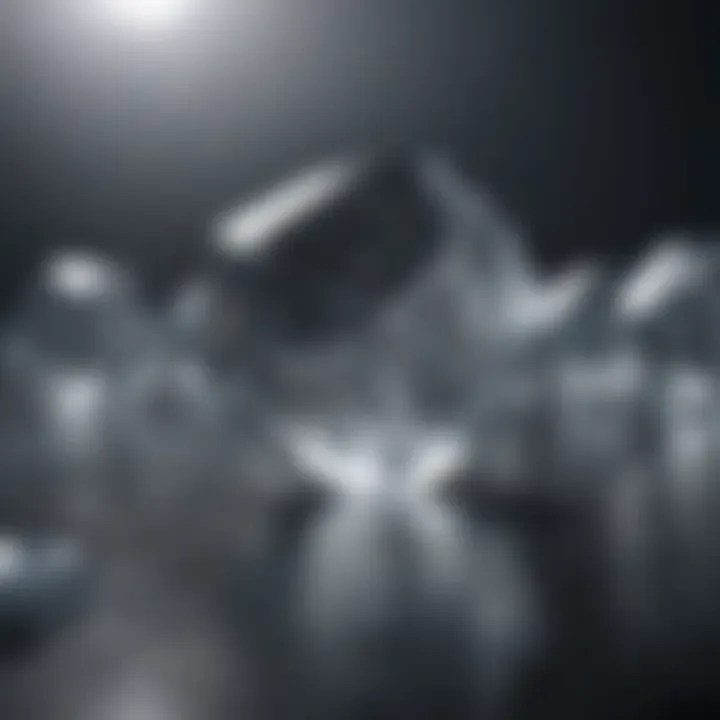
Metal hydride storage methods present a compelling approach to hydrogen storage due to their unique ability to absorb hydrogen gas into solid metal lattices. This section delves into the specifics of how this technology functions, the materials used, and its practical applications. Understanding metal hydride storage is vital for advancing hydrogen energy as it represents a promising solution in the quest for efficient and safe hydrogen storage.
Mechanism of Hydrogen Absorption
Metal hydrides behave as sponges for hydrogen. The absorption process begins when hydrogen gas comes into contact with a suitable metal, such as titanium or magnesium. The gas then dissociates into atomic hydrogen, which is absorbed into the metal lattice structure. This process involves both physical and chemical interactions.
- Hydrogen Dissociation: Hydrogen molecules (H₂) split into two hydrogen atoms (H) upon contact with the metal surface.
- Solid Solution Formation: These hydrogen atoms then diffuse into the metal lattice, where they occupy interstitial sites.
- Thermal Effects: The temperature often influences the absorption rate, highlighting the need for optimal thermal management.
This absorption mechanism significantly increases hydrogen density, enabling a more compact storage solution than gaseous hydrogen or liquid hydrogen.
Material Options and Their Properties
Numerous materials can form metal hydrides, each exhibiting unique properties that influence their applicability:
- Titanium Alloys: Popular for their high storage capacity and stability; they also function effectively at moderate temperatures.
- Magnesium Hydride: Offers a high hydrogen weight ratio but requires higher temperatures for efficient absorption.
- Lanthanum Nickel Hydride: Known for rapid kinetics; useful in applications needing quick refueling times.
Understanding the properties of these materials allows for improved selection based on specific application requirements, such as weight sensitivity or economic feasibility.
Commercial Applications and Research Developments
Metal hydride storage finds application in several fields, primarily in fuel cell technology, transportation, and stationary energy storage. Current implementations include:
- Fuel Cell Vehicles: Companies like Honda and BMW utilize metal hydride systems to store hydrogen safely and efficiently.
- Portable Power Systems: Compact power sources for electronic devices frequently employ metal hydride systems due to their weight advantages.
- Research Innovations: Ongoing research focuses on developing new metal hydride compounds with better performance metrics, including lower operating temperatures and increased storage capacities.
“Metal hydride storage continues to evolve, helping bridge the gap between conventional energy resources and a sustainable hydrogen economy.”
The future looks bright for this technology as researchers explore novel alloys and composite materials aimed at maximizing storage efficiency and minimizing costs.
Chemical Hydrogen Storage
Chemical hydrogen storage plays an essential role within the broader context of hydrogen storage methods. The technique shifts the focus from physical to chemical interactions, enabling the capture and release of hydrogen through various reactions. This approach is significant for enhancing energy density compared to traditional storage methods, addressing some of the limitations encountered in sectors demanding higher efficiencies.
The primary benefit of chemical hydrogen storage lies in its potential to operate at moderate pressures and temperatures, easing some safety concerns. Furthermore, it offers pathways for long-term storage and transport, making it an attractive option for stationary applications as well as mobile ones.
Reaction Mechanisms and Kinetics
Understanding the reaction mechanisms and kinetics involved in chemical hydrogen storage is fundamental for optimizing its efficiency. Typically, chemical storage involves reversible reactions where hydrogen is released during certain processes.
Common reaction mechanisms include:
- Dehydrogenation: The process of releasing hydrogen from chemical compounds, often involving heat to facilitate the reaction.
- Hydrogenation: The addition of hydrogen to a substance, forming stable compounds.
Kinetic aspects greatly influence the efficiency of these processes. For instance, reaction rates, temperature stability, and catalyst presence can all determine how swiftly hydrogen can be stored or released. Therefore, research continues into finding or designing chemical compounds that enhance stability while providing the speed required for practical applications.
Potential Chemical Candidates
Numerous compounds have been investigated for their chemical hydrogen storage potential. These candidates must exhibit suitable thermodynamic and kinetic properties. Noteworthy candidates include:
- Ammonia (N): Serves as both a hydrogen carrier and an energy source, with well-established synthesis routes.
- Formic Acid (HCOOH): Known for its ease of decomposition and relatively low toxicity, it produces carbon dioxide and hydrogen upon breaking down.
- Metal Hydrides: Combinations of metals with hydrogen which release hydrogen upon reaction with heat or catalysts.
A thorough analysis of these candidates reveals their respective advantages and limitations. For example, while ammonia is favorable due to its existing infrastructure, its toxicity must be carefully managed.
Storage Efficiency and Environmental Impact
The storage efficiency of chemical hydrogen systems is a critical factor that determines their viability on a large scale. Evaluating performance often involves metrics such as gravimetric and volumetric capacities, which reflect how much hydrogen can be effectively stored relative to the weight and volume of the storage medium.
Additionally, the environmental impact of chemical hydrogen storage must not be overlooked. The carbon footprint, energy consumption in production, and end-of-life options for hydrogen carriers are crucial aspects. Ideally, the entire lifecycle must minimize emissions and waste, aligning with sustainable energy goals.
In summary, chemical hydrogen storage offers a promising avenue for meeting future energy demands. Its mechanisms, candidates, and considerations underpin the ongoing evolution of hydrogen energy technologies. This method not only possesses potential for improving storage capabilities but also emphasizes the need for responsible management of both resources and environmental implications.
Advanced Hydrogen Storage Technologies
Advanced hydrogen storage technologies are crucial in addressing the growing demand for hydrogen as a clean energy carrier. These methods aim to improve efficiency, reduce costs, and enhance safety compared to traditional storage techniques. Innovations in this field can support the production and distribution of hydrogen at a larger scale, which aligns with global energy goals.
Nanomaterials and Their Applications
Nanomaterials have emerged as a significant influencer in hydrogen storage solutions. Their unique properties at the nanoscale allow for higher absorption rates and greater surface area, leading to better performance. Materials like carbon nanotubes and metal-organic frameworks (MOFs) are now explored for their hydrogen storage capabilities.
- Carbon Nanotubes: These structures can potentially store hydrogen at a higher density than conventional materials. Their structure allows hydrogen molecules to be trapped within the tubes, enhancing storage capacity in smaller volumes.
- Metal-Organic Frameworks (MOFs): MOFs offer a tunable porosity. Their flexibility in design allows researchers to optimize hydrogen storage capacity while maintaining low weight.
The use of nanomaterials can significantly improve the overall energy efficiency and sustainability of hydrogen systems
Hydrogen Sorption Techniques
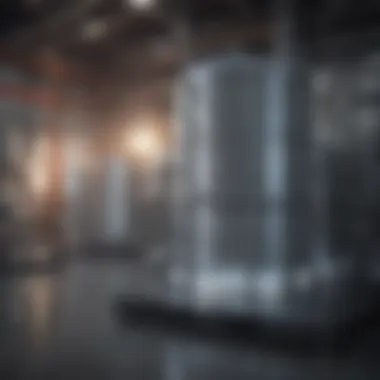
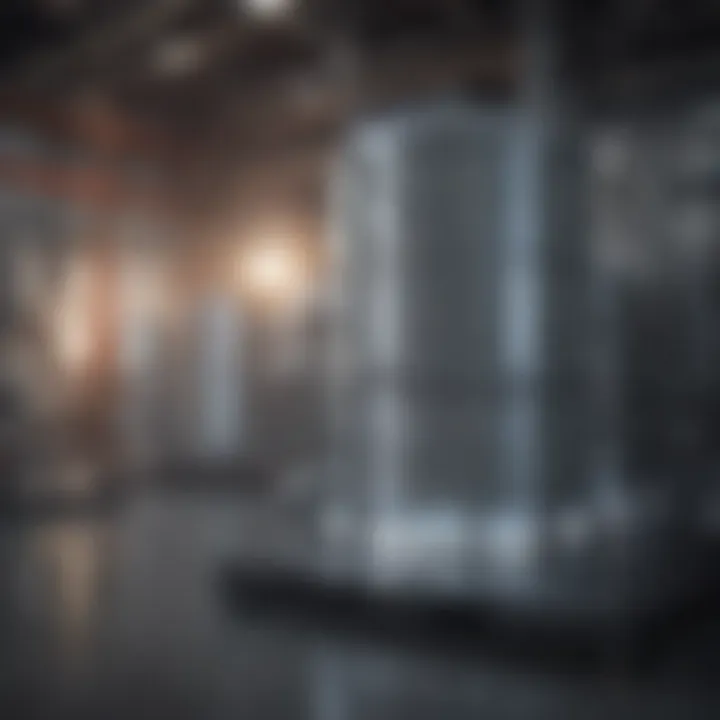
Hydrogen sorption involves the absorption of hydrogen into solid materials. This process can be used in applications with mobility, such as in storage tanks for fuel cell vehicles. Various strategies are currently used to improve the sorption process, such as modifying surface areas or changing temperature and pressure conditions.
- Physisorption: This technique relies on weak Van der Waals forces to bind hydrogen to the sorbent material. While the energy requirements are minimal, storage densities are lower compared to other techniques.
- Chemisorption: This process involves a chemical reaction, allowing for stronger bonding between the hydrogen and material. It can provide higher storage densities but often requires more energy during the adsorption and desorption processes.
Current research focuses on optimizing sorbent materials and conditions to achieve faster uptake and release while minimizing energy requirements.
Potential Future Innovations
The landscape of hydrogen storage is continuously evolving, with future innovations promising enhanced efficiency and cost-effectiveness. Some potential areas of development include:
- Advanced Alloys: Research into new metal alloys for hydrogen storage aims to enhance sorption capabilities while reducing weight.
- Synthetic Chemical Processes: Future advancements may involve novel chemical pathways for creating more effective hydrogen storage systems with lower environmental impact.
- Smart Storage Technologies: Incorporating IoT and advanced sensors could optimize storage environments, monitoring conditions to maximize safety and efficiency.
"With continuous research and technological advancements in hydrogen storage, we can expect solutions that significantly contribute to a sustainable energy future."
As these innovations emerge, hydrogen's role as a key player in the energy transition becomes more prominent. Understanding advanced technologies is essential for students, researchers, and professionals involved in energy production, storage, and utilization.
Regulatory and Safety Standards
The topic of regulatory and safety standards in hydrogen storage is of paramount importance due to the unique challenges associated with storing and handling hydrogen. As hydrogen is a highly flammable gas, strict protocols and regulations are essential to ensure safety across various sectors. Implementing appropriate standards helps prevent accidents, facilitates compliance among industry players, and promotes public confidence in hydrogen technologies. Without such regulations, the full potential of hydrogen as a clean energy carrier could remain unrealized due to safety concerns.
National Regulations Governing Hydrogen Storage
National regulations for hydrogen storage vary from country to country, reflecting local safety needs and industry standards. In the United States, the Department of Transportation oversees regulations concerning hydrogen transport, focusing on safe storage containers and transport methods. Additionally, the Occupational Safety and Health Administration establishes safety guidelines in workplaces where hydrogen is manipulated.
In regions like Europe, the European Union has set frameworks through various directives, such as the Pressure Equipment Directive, which specifies design and safety requirements for equipment used in hydrogen storage. These regulations help ensure that all installations meet safety standards while allowing ongoing innovation in storage technologies.
- Key Elements of National Regulations:
- Container specifications and strength requirements
- Safety training for personnel handling hydrogen
- Transparent reporting and signage for storage facilities
- Regular inspections to ensure compliance
International Guidelines and Best Practices
On an international level, several organizations, such as the International Organization for Standardization (ISO) and the International Electrotechnical Commission (IEC), play a crucial role in formulating guidelines that transcend national borders. These guidelines provide a unified framework for safe practices in hydrogen storage, addressing multiple aspects from engineering design to emergency response practices.
The key to effective international standards is harmonization. When different countries adopt similar safety standards, it eases international trade of hydrogen technologies and components. Guidelines help companies understand expectations in different jurisdictions, minimizing the risk of accidents and fostering a culture of safety across the industry.
Effective implementation of international guidelines not only enhances safety but also builds trust in hydrogen technologies, as industry stakeholders become confident that all parties comply with recognized safety norms.
- Critical Components of International Guidelines:
- Standardized testing methods for hydrogen storage systems
- Protocols for emergency protocols including hazard assessment
- Environmental considerations to minimize impact
- Certification processes for new technologies
Economic Considerations
The economic aspects of hydrogen storage are crucial for the viability of hydrogen as a clean energy source. Understanding these elements allows stakeholders to assess the feasibility of different storage methods. It also helps in identifying potential barriers that can affect large-scale adoption. Economic considerations inform policy-making. They guide investments and research directions, making them vital for creating a sustainable hydrogen economy.
Cost Analysis of Different Storage Methods
Performing a thorough cost analysis of various hydrogen storage methods reveals substantial differences that impact overall efficiency and practicality.
- Compressed Gas Storage: This method incurs expenses primarily due to equipment costs for high-pressure tanks. Operational costs include energy consumed during compression. While setup may be less costly, maintaining safety standards raises ongoing expenses.
- Liquid Hydrogen Storage: Liquefaction is energy-intensive. The initial costs for cryogenic tanks and facilities are high. However, this method allows for dense storage, making it economical for specific applications such as fuel for spacecraft.
- Metal Hydride Storage: High costs related to materials and manufacturing processes can be a barrier. Yet, the ability to operate at lower pressures could reduce infrastructure investments over time.
- Chemical Hydrogen Storage: The financial analysis reveals implications related to chemical processing costs and catalyst expenses. Long-term efficiency through regeneration can alleviate initial costs.
Thus, while some methods may seem expensive initially, the long-term financial implications and efficiency can shift the analysis significantly.
Impact on the Hydrogen Economy
Economic viability directly influences the hydrogen economy’s growth. A cost-effective method will make hydrogen storage accessible, consequently increasing hydrogen’s role in the energy sector.
- Investment in Infrastructure: As the demand for hydrogen rises, so does the need for advanced storage solutions. Economically favorable methods encourage investment in infrastructure, essential for a robust market.
- Job Creation and Growth: Development and implementation of hydrogen storage technologies can stimulate job growth in various sectors, from manufacturing to research and development.
- Sustainability and Energy Transition: Affordable storage options enhance hydrogen's potential to replace fossil fuels. This aligns with broader environmental goals and supports global sustainability efforts.
End and Future Perspectives
The importance of concluding remarks in this article centers on synthesizing the diverse methods of hydrogen storage. This reflection is not merely for summarization but serves to underscore the evolving landscape of hydrogen technologies. Current energy demands and environmental concerns drive the need for efficient and safe storage solutions. Understanding the methodologies discussed enables stakeholders to make informed decisions regarding hydrogen deployment in various sectors.
As hydrogen storage technologies continue to advance, the conversations around them gain significance. They play a critical role in paving paths toward sustainable energy systems. Stakeholders like researchers, policymakers, and industry players can benefit immensely from grasping the nuances of each storage method. We have seen that while conventional techniques, such as compressed gas and liquid storage, have established their place, advanced methods like metal hydrides and chemical storage display promising potentials.
Summary of Key Findings
In examining the various methods of hydrogen storage, this article highlighted the following key points:
- Conventional Techniques: Compressed gas and liquid hydrogen storage remain prevalent due to their maturity and established infrastructure. However, each has inherent limitations such as efficiency and safety issues.
- Advanced Storage Solutions: Techniques such as metal hydride and chemical hydrogen storage offer innovative approaches but come with their own sets of challenges related to cost and complexity.
- Safety and Regulations: Understanding existing safety standards and regulations is essential for any hydrogen storage method. This is pivotal in ensuring the safe handling, storage, and transportation of hydrogen.
- Economic Factors: The economics of hydrogen storage involve significant costs that impact the feasibility of adopting certain technologies in particular markets.
Research Directions and Innovations Ahead
Future research in hydrogen storage technologies holds vast potential. Several directions may shape how hydrogen storage develops:
- Nanomaterials: Exploring the application of nanotechnology in hydrogen storage may lead to materials with improved storage capacity and efficiency, addressing existing limitations.
- Chemical and Biological Processes: Innovative chemical methods and bio-inspired solutions could present new ways to improve hydrogen absorption and release mechanisms, enhancing overall performance.
- Focus on Safety and Regulations: Ongoing research directed at establishing better safety protocols and regulatory standards will be crucial as these technologies are commercialized.
- Sustainable and Cost-effective Solutions: The push for greener technologies demands research into more sustainable materials and processes that lower costs associated with hydrogen production and storage.
"The future of hydrogen storage is not only about novel methods but also about the integrated understanding of safety, economics, and sustainability."