Innovative Nanolithography: Techniques and Uses
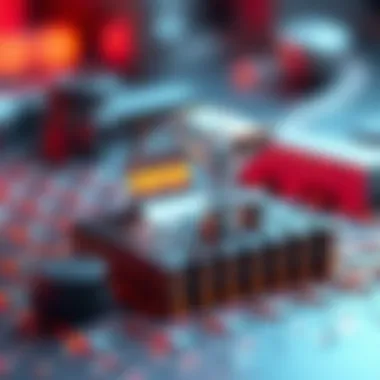
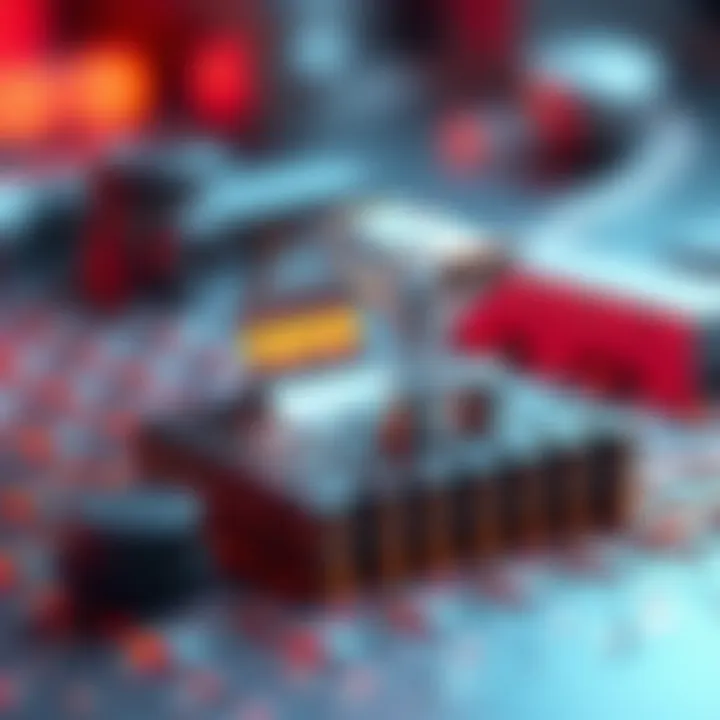
Intro
Nanolithography is like the magic wand in the realm of tiny tech. As the need for creating finer and more intricate patterns in materials becomes paramount, this technique stands out. With its roots deep in both scientific research and industrial applications, it plays a fundamental role in areas where precision is key.
Whether it's crafting the circuits of next-gen electronics or developing sophisticated drug delivery systems in biotechnology, the influence of nanolithography is everywhere. This article sheds light on the various methodologies used, the materials that serve as the building blocks, and the mammoth advancements made in this field. By diving into both the theoretical frameworks and practical uses of nanolithography, we aim to connect the dots between this fascinating discipline and its real-world implications, unfolding a narrative that serves students, researchers, educators, and professionals alike.
The relevance of nanolithography can no longer be overlooked. With its pivotal role in the ongoing technology revolution, it has not just paved the way for innovations but has also opened new avenues for research in electronics, materials science, and biotechnology.
"Understanding nanolithography is understanding the future of technology—it lays the groundwork for what’s to come."
This journey will guide you through the ins and outs of nanolithography, revealing its methodologies and applications while highlighting the critical insights gained from ongoing research.
Prelims to Nanolithography
Nanolithography stands as a key player in modern fabrication techniques, serving as a fundamental bridge between scientific theory and practical application. This intricate process involves creating nanoscale patterns on surfaces, and its significance has skyrocketed due to its utility in various cutting-edge fields. As we peel back the layers of this fascinating subject, we will encounter its myriad methodologies, materials, and the groundbreaking advancements it facilitates.
Definition and Importance
At its core, nanolithography is the art and science of manipulating materials at the nanoscale, typically defined as ranging from 1 to 100 nanometers. This definition may sound straightforward, yet it encapsulates a sophisticated interplay of physics, chemistry, and engineering principles. The need for such precision is paramount in industries where the size of components impacts functionality and performance. For instance, in semiconductor manufacturing, transistors now require intricate designs that can only be achieved through nanolithographic techniques.
The importance of this field is rooted in its ability to enable advancements in technology that drive daily operations in electronics, medicine, and material sciences. Below are a few key elements that illustrate its significance:
- Innovative Manufacturing: Nanolithography is essential in producing components with high density and improved performance, playing a crucial role in the miniaturization of electronic devices.
- Research and Development: The techniques developed pave the way for discoveries in material properties, opening new avenues for research across multiple scientific disciplines.
- Tailored Solutions: Nanolithography allows for the customization of materials and surfaces, which is instrumental in specialized applications such as drug delivery in nanomedicine.
Historical Background
The roots of nanolithography can be traced back several decades, heavily influenced by the advancement of optical microscopies and etching techniques. The early 20th century saw the birth of lithography techniques that catered primarily to larger scales. It wasn't until the late 1970s and early 1980s that researchers began to explore the potential for nanoscale patterning.
Key milestones in the evolution of nanolithography include:
- 1977: The introduction of electron-beam lithography, which allowed for unprecedented resolution and flexibility, enabling patterning at the nanoscale.
- 1990s: The birth of scanning probe lithography, offering innovative ways to manipulate materials at atomic or molecular levels. This marked a pivotal shift in how we understand and utilize nanostructures.
- 2000s - Present: Recent advancements have wildly expanded the possibilities, with techniques like nanoimprint lithography emerging to enable mass production of nanoscale patterns efficiently.
Although various nanolithography techniques have evolved, the journey of this domain continues to progress, driven by an insatiable quest for increased precision and efficiency. As we proceed, we'll uncover deeper insights into fundamental principles and various methodologies, reflecting on both the opportunities and challenges that lie ahead in this captivating field.
Fundamental Principles
Understanding the fundamental principles of nanolithography is essential for grasping how tiny structures are created and manipulated at the nanoscale. These principles serve as the bedrock upon which various nanolithographic techniques are built, encompassing both theoretical frameworks and practical methodologies. Without a solid comprehension of these foundations, the complexities of advanced nanolithography would be akin to navigating a maze with no map. The importance of these principles extends far beyond academic study; they hold significant implications for sectors like electronics, pharmaceutical development, and nanoengineering, influencing both ongoing research and commercial applications.
Basic Concepts of Lithography
At its core, lithography is a process used to transfer patterns onto a substrate. The basic workflow involves several stages, including coating, exposure, development, and etching. Each of these stages plays a crucial role in achieving the desired patterns with high precision.
- Coating: A light-sensitive material, known as a resist, is applied to the substrate. The choice of resist can affect not just the resolution but also the fidelity of the final pattern.
- Exposure: The coated substrate is then exposed to a radiation source (light, electrons, or x-rays, depending on the lithography type), which alters the chemical structure of the resist. This exposure determines the areas where material will either be removed or protected in subsequent steps.
- Development: After exposure, the substrate undergoes a development process, in which the unexposed or exposed areas of the resist are selectively removed, revealing the underlying substrate.
- Etching: Finally, an etching process is employed to remove material from the substrate in the areas that were not protected by the resist. This step is critical for achieving high-resolution features needed in nanostructures.
The intricacies involved in lithography require a careful balance of various parameters, such as exposure dose, resist thickness, and development time. The interaction between these elements often defines the overall success of the process, making a detailed understanding indispensable for researchers and practitioners alike.
"The secret to mastering lithography lies not just in knowing the steps, but in understanding the material science and physical principles behind them."
Dimensional Scaling and Resolution Limitations
As we aim for smaller structures in nanolithography, the principle of dimensional scaling becomes critically significant. In essence, dimensional scaling refers to the need to manage sizes and dimensions of features with increasing precision as they approach the nanoscale. Generally, as the length scales decrease, several challenges surface, particularly concerning resolution limits.
- Resolution Limitations:
- Material Limitations:
- The ability to define features is often constrained by the wavelength of light used during the exposure stage. For example, conventional photolithography is typically limited by the diffraction of light, which can prevent the accurate definition of features smaller than a certain threshold (often in the range of hundreds of nanometers).
- In electron beam lithography, while higher resolution can be achieved due to shorter wavelengths, trade-offs emerge related to throughput and costs. This means a careful balance of techniques must be applied based on the desired application.
- The materials used must also demonstrate appropriate physical and chemical properties to survive multiple exposure and development cycles. Selecting the right resist and substrate materials is paramount to overcoming limitations posed by dimensional scaling.
In summary, the fundamental principles of nanolithography create the framework for innovation in the field. By grasping the basic concepts and recognizing the challenges of scaling and resolution, researchers can better navigate the intricate landscape of nanostructured materials.
Types of Nanolithography
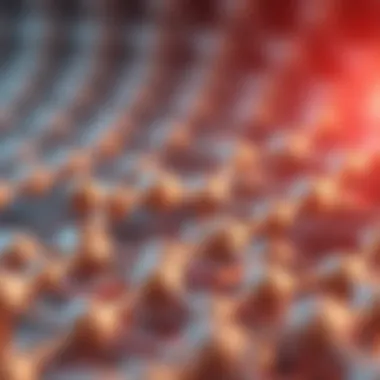
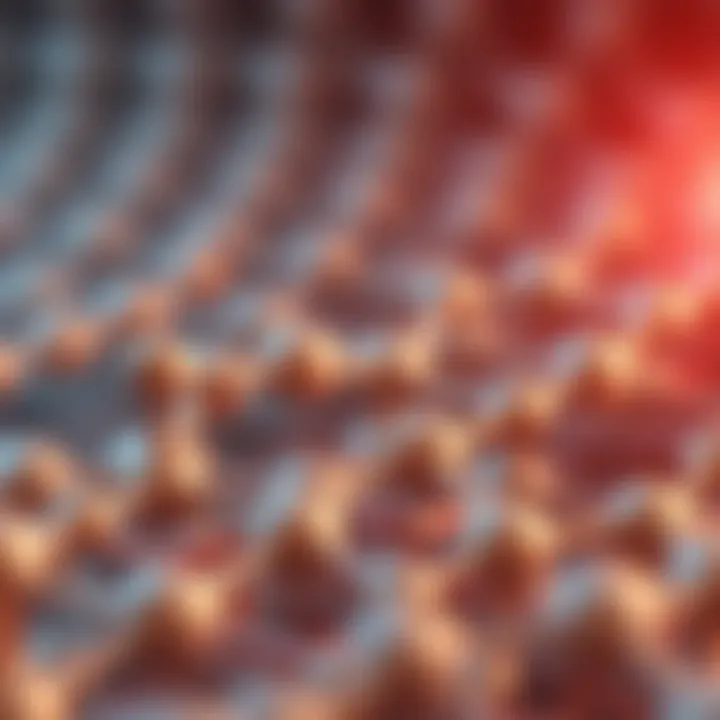
Understanding the diverse types of nanolithography is crucial for anyone interested in the field. Each method presents its own unique advantages and challenges, all while addressing specific needs within various applications. The evolution of nanolithography techniques has allowed for innovations in electronics, medicine, and material science, solidifying their importance in modern research and industry.
Photolithography
Photolithography is often the first method that comes to mind when discussing nanolithography. This process relies on light to transfer patterns onto a substrate coated with a photosensitive material known as a resist. The patterned light exposes specific paths on the resist, which are then developed to leave behind a precise structure.
The primary benefits of photolithography lie in its scalability and speed. It's capable of producing intricate patterns over large areas, making it essential for semiconductor manufacturing. However, one must consider the resolution limits inherent to this method. As feature sizes shrink to below 10 nanometers, photolithography faces significant challenges. These include diffraction limits of light, requiring continuous advancements such as the move towards extreme ultraviolet (EUV) lithography.
Electron Beam Lithography
Electron beam lithography (EBL) offers a different approach by employing focused beams of electrons to write custom patterns on a resist material. This method is noteworthy for its ability to achieve higher resolutions than photolithography. EBL allows for the creation of features well below 5 nanometers, making it particularly useful for prototyping and applications requiring ultimate precision.
Despite its accuracy, EBL comes with drawbacks such as slow processing speeds. The write time can be significant, especially for larger patterns, which limits its application for mass production. Nevertheless, EBL is invaluable in research settings, enabling detailed investigations into nanoscale phenomena and the development of new materials.
X-Ray Lithography
X-ray lithography introduces x-rays as the medium of exposure, offering advantages over optical techniques due to their shorter wavelengths, which allow for much smaller feature sizes. This method can achieve resolutions approaching 10 nanometers, making it suitable for advanced semiconductor applications.
One of the key advantages of X-ray lithography is its ability to work with thicker resists. However, it isn't without its challenges. The technology requires expensive x-ray sources and specialized facilities, making it less accessible than other methods. It's crucial to carefully evaluate whether the benefits outweigh the costs, especially for smaller operations.
Nanoimprint Lithography
Nanoimprint lithography (NIL) operates on a completely different principle. Rather than using light or electrons, it involves pressing a mold into a resist material to form nanostructures. This technique has been praised for its simplicity and the ability to produce high-resolution patterns at significantly lower costs compared to other methods.
The key advantage here is scalability; nanoimprint lithography can potentially achieve large-scale production of nanoscale features. However, it also suffers from limitations in material versatility and mold durability. Careful consideration is required when selecting resists and designs to ensure effectiveness and longevity in the final product.
"Each method of nanolithography has its unique advantages and drawbacks, making the choice largely dependent on application-specific requirements."
Using a combination of these techniques may provide the best results, allowing researchers and manufacturers to leverage the strengths of each method to meet their unique challenges.
Overall, the types of nanolithography provide a framework for advancing technology and innovation across various fields. As these techniques continue to evolve, their role in shaping future technologies cannot be underestimated.
Materials Used in Nanolithography
The materials employed in nanolithography are the unsung heroes that enable the precision and functionality of nanoscale devices. Selecting appropriate materials is not just a matter of convenience; it's crucial for enhancing performance, achieving desired structures, and addressing specific requirements in various applications. With the continuous evolution of technology, understanding these materials becomes paramount in harnessing the full potential of nanolithographic techniques.
Resists and Their Properties
Resists are specialized materials that play an essential role in the lithography process. They serve as a protective layer over substrates, allowing selective etching or deposition to occur on specific regions. There are several types of resists used, including positive and negative resists, each with unique properties that cater to various applications.
Positive resists become soluble when exposed to radiation, leading to the intended patterns. On the contrary, negative resists harden upon exposure, rendering the unexposed areas soluble. The choice between these types often depends on the resolution required and the nature of the subsequent processes.
Some essential properties to consider when selecting resists include:
- Sensitivity: The ability of the resist to react to the radiation exposure. Higher sensitivity can reduce exposure times but may affect the resolution.
- Resolution: The smallest feature size that can be reliably created, which can be influenced by factors such as resist thickness and the quality of the exposure system.
- Etch Resistance: A crucial factor; resists must withstand various etching processes without degrading, ensuring that the patterns remain intact.
"The choice of resist can significantly impact the fidelity and reliability of the nanostructures that are produced."
In terms of application, the semiconductor industry heavily relies on advanced resists to create intricate circuits with extremely high levels of integration. Innovations in resist materials, such as chemically amplified resists, continue to drive forward the capabilities of nanolithography, pushing the boundaries of technology.
Substrate Materials
The substrate acts as the foundation for the nanostructures being developed. Choosing the right substrate material is critical because it can profoundly influence the adhesion of the resist, the optical properties during exposure, and the overall functionality of the finished product.
Common substrate materials include silicon wafers, glass, and even flexible polymers, each bringing unique advantages:
- Silicon: Predominantly used in the semiconductor industry, silicon provides excellent compatibility with electronic devices. Its intrinsic electrical properties make it a natural choice for many applications.
- Glass: Used for optics and displays, glass substrates allow for high transparency, which is vital in processes requiring UV light in photolithography. Their smooth surface finish is beneficial for achieving accurate patterns.
- Flexible Polymers: Gain traction in applications like flexible electronics, these materials can offer advantages in terms of weight and flexibility. However, they may have limitations in terms of thermal stability and etching compatibility.
Key considerations when selecting substrates include:
- Thermal Conductivity: Important for managing heat during the lithography process and subsequent device operation.
- Surface Smoothness: Affects the quality of the resist application and the resolution during patterning.
- Chemical Compatibility: Ensures that the substrate can withstand the various chemical processes used during lithography.
Process Overview
Understanding the process overview of nanolithography is essential for grasping how this intricate art contributes to a range of industries, particularly those engaged with nanotechnology. This section sheds light on the various steps involved in the process, highlighting its significance, benefits, and the critical considerations that accompany each stage of nanolithographic applications.
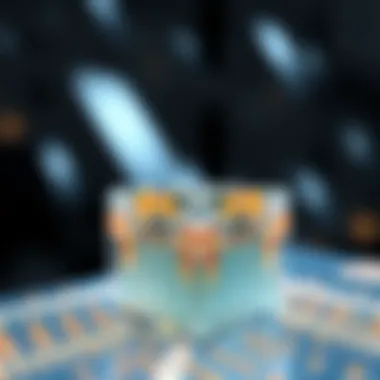
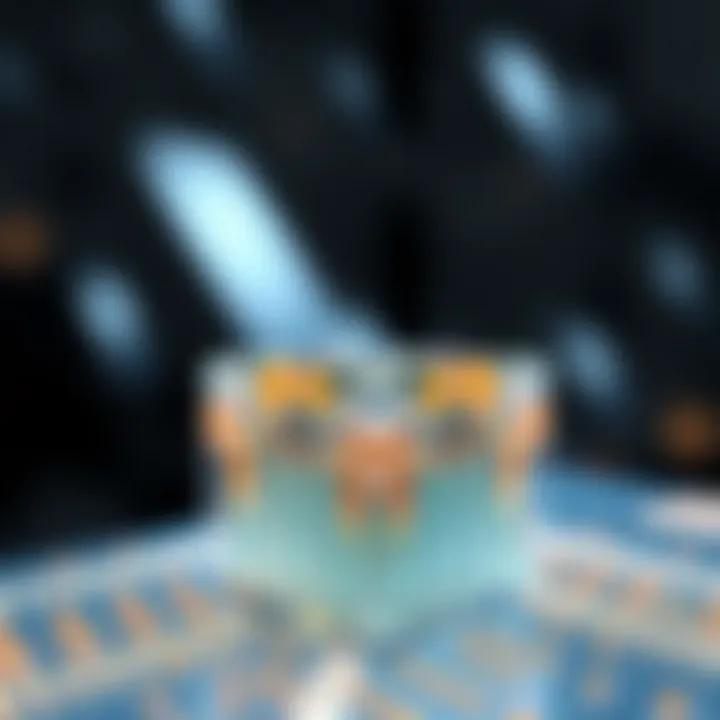
Nanolithography serves as a bridge between theoretical science and practical engineering. The process revolves around crafting nanoscale patterns on various materials, which is crucial for evolving technologies ranging from semiconductor devices to medical tools. By dissecting the stages of this process, one can better appreciate how each component fits into the larger framework that drives innovation in electronics, materials, and life sciences.
Step-by-Step Procedure
The step-by-step procedure in nanolithography typically unfolds as follows:
- Substrate Preparation: It all starts with cleaning the substrate material thoroughly. Any impurities can lead to defects in the final product. This preparation can involve chemical baths or plasma treatments to enhance adhesion and remove contaminants.
- Resist Application: A layer of photoresist material is then applied. This resist will form the pattern once exposed to the lithographic light or electrons. Application techniques may vary, but spin-coating is commonly favored due to its uniform thickness.
- Exposure: Next, the resist is exposed to a specific light wavelength or electrons. The exposure can be selective, depending on the desired pattern, such as through mask application in photolithography or direct writing in electron beam lithography.
- Development: After exposure, the substrate undergoes development. In this step, the unexposed areas of the resist are washed away, revealing the substrate beneath in the desired pattern. Care must be taken to ensure precise control over this process since under or over-development can lead to inaccuracies.
- Etching: Following development, etching removes the exposed substrate material, transferring the pattern from the resist onto the surface. Techniques such as wet or dry etching are utilized depending on the materials involved and the patterns needed.
- Resist Removal: After etching, the remaining resist is stripped away, leaving behind the final structure on the substrate. This step can use chemical solvents or plasma treatment.
This methodical approach allows researchers and manufacturers to craft nano-features with an impressive degree of precision, paving the way for advancements in various fields, particularly in the production of microelectronics and lab-on-a-chip devices.
Characterization Techniques Post-Processing
After the completion of the lithographic process, characterization techniques come into play. These methods are pivotal for validating the efficacy of the nanolithography process and the quality of the fabricated structures. A few widely used characterization techniques include:
- Scanning Electron Microscopy (SEM): This technique is invaluable for topographical mapping of nanostructures. By providing high-resolution images, SEM helps in assessing pattern fidelity and feature sizes.
- Atomic Force Microscopy (AFM): AFM offers a three-dimensional perspective on surface features. The resolution achieved can extend down to a single nanometer, making it crucial for depth profiling and surface roughness evaluation.
- X-Ray Diffraction (XRD): This helps determine crystallographic properties in nanostructures, essential for understanding the material's physical properties and performance in applications.
- Spectroscopic Techniques: Techniques like Raman or FourierTransform Infrared Spectroscopy (FTIR) assist in analyzing the chemical compositions and structural properties of the materials post-processing.
In summary, the insights gathered from these characterization techniques not only confirm the successful fabrication of nanostructures but also provide a feedback loop for improving and refining the lithography processes. By integrating these evaluations into the workflow, scientists can push the needle forward in the quest for higher precision and functionality in nanofabricated devices.
Understanding each phase of the process helps ensure that advancements in nanotechnology remain anchored in scientific rigor and practical applicability, reinforcing its role as a cornerstone of modern technology.
Applications of Nanolithography
The applications of nanolithography extend far and wide, playing a pivotal role in shaping modern technology and advancing various fields. This section delves into semiconductor manufacturing, nanomedicine, optoelectronics, and flexible electronics, all of which exemplify the incredible potential and versatility of nanolithographic techniques. By harnessing these methods, researchers and industries are able to create miniaturized components and novel devices that were once mere science fiction. Here's a closer look at each of these realms.
Semiconductor Manufacturing
In the realm of semiconductor manufacturing, nanolithography has become indispensable. Chips, which power everything from smartphones to supercomputers, rely heavily on nanoscale precision during their fabrication. Photolithography, a dominant technique, allows for the creation of intricate circuit patterns on semiconductor substrates. This process involves photosensitive materials that undergo a chemical change when exposed to light, enabling finer detail and higher resolution in chip designs.
The significance here cannot be overstated. As the demand for faster and smaller devices increases, semiconductor manufacturers chase Moore’s Law, which predicts that the number of transistors on a chip will double approximately every two years. As we push the limits of this law, nanolithographic advancements, such as extreme ultraviolet (EUV) lithography, promise to keep up with this relentless pace. With EUV technology, features as small as 7 nanometers can be etched onto silicon wafers, paving the way for the next generation of electronics.
Nanomedicine
Transitioning from silicon to the biological realm, nanolithography's contributions to nanomedicine showcase its impact on healthcare. Here, the technique is utilized to fabricate nanoscale devices that diagnose and treat diseases more effectively. For instance, targeted drug delivery systems can be designed using nanostructures that ensure the precise release of therapeutics at tumor sites, minimizing side effects and enhancing treatment efficacy.
Nanolithography also enables the creation of diagnostic tools that detect biomarkers at incredibly low concentrations. These tools can transform how diseases are diagnosed, allowing for earlier intervention and improved patient outcomes. As researchers design nanoparticles that can navigate through biological environments, the future of medicine may see even more integrated approaches to treatment.
Optoelectronics
In optoelectronics, nanolithography paves the way for devices that merge optical and electronic functionalities. Components such as light-emitting diodes (LEDs), solar cells, and photodetectors benefit from nanoscale structuring. By manipulating light at the nanoscale level, manufacturers can improve device efficiency dramatically.
Consider quantum dots, tiny semiconductor particles that exhibit unique optical properties based on their size. Through nanoscale fabrication, optoelectronic devices incorporating quantum dots can lead to advances in display technology, enhancing color accuracy in televisions and smartphones. More importantly, these advancements also hold promise for more efficient solar energy conversion, as nanoscale light trapping can enhance the absorption of sunlight.
Flexible Electronics
Lastly, the emergence of flexible electronics illustrates how nanolithography can adapt traditional methods to meet modern demands. These devices, which can bend, stretch, or twist, find applications in wearable technology, smart textiles, and flexible displays. The unique fabrication techniques enabled by nanolithography allow for the integration of electronic components onto pliable substrates without compromising functionality.
One example includes the development of flexible solar panels that can be integrated into clothing or used in unconventional surfaces. The lightweight design and efficient energy harnessing of these products are game-changers for sustainable energy solutions. Additionally, customizing wearable health-monitoring devices through nanolithographic processes opens new avenues for real-time personal health analysis.
"Nanolithography is not just shaping technology; it’s reshaping our reality by making once-impossible applications a tangible part of our everyday lives."
Challenges in Nanolithography
Nanolithography, while transformative, is not without its hurdles. Addressing the challenges inherent to this intricate process is critical for its advancement and broader application. From cost barriers to material constraints, the road ahead is filled with both opportunities and obstacles. Understanding these challenges provides vital context for the innovations that drive the field forward and highlights areas where focused efforts can yield significant rewards.
Cost and Scalability Issues
The financial implications of nanolithography cannot be understated. The high upfront investment associated with advanced equipment—such as electron beam lithography machines—often deters smaller companies and research institutions from diving into the fray. As a result, the technology remains largely in the hands of well-funded organizations or large-scale manufacturers.
Moreover, the cost extends beyond the machinery itself. Maintaining and operating these systems at the required standards demands both specialized personnel and considerable overhead costs. For example, companies are often faced with the dilemma of hiring highly skilled labor, which further inflates the budget.
Here are some key aspects to consider regarding cost and scalability issues:
- Operational Costs: Day-to-day running costs can spiral, especially in environments needing strict cleanroom standards.
- Economic Viability of Small-Batch Production: The processes are typically suited for high-volume outputs. Whether for academic research or small-scale startups, low output runs can become economically unviable.
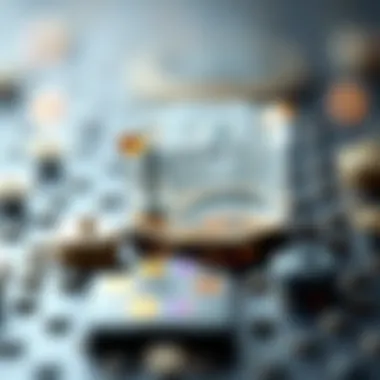
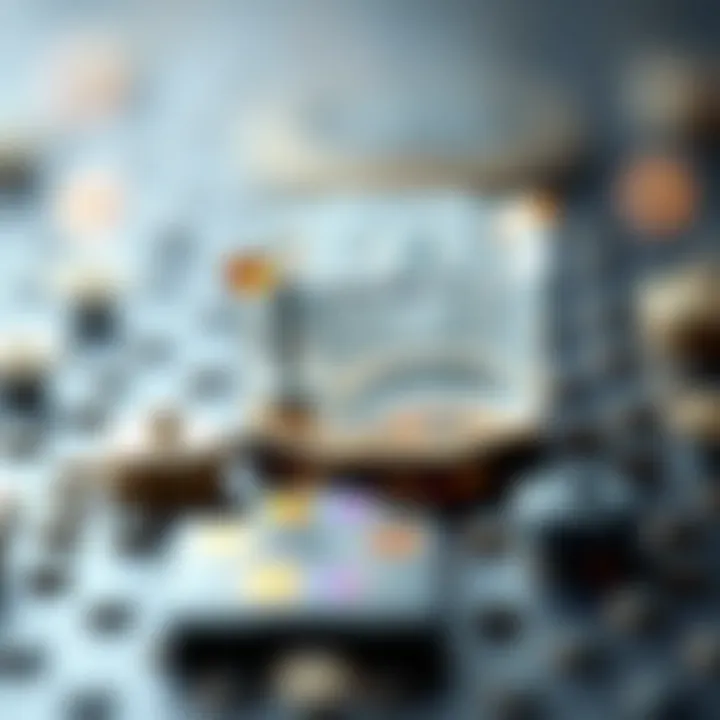
The solution to these issues may lie in innovation that allows for more versatile systems or the development of alternative methods that require lesser inputs to operate efficiently. Furthermore, cooperation between academia and industry could foster an ecosystem where resources are pooled, ultimately reducing costs.
Material Limitations
A critical aspect that complicates nanolithography is the variety of materials involved. Each material brings its own set of properties, which can be a double-edged sword. For instance, photoresists must exhibit excellent resolution while also being chemically stable during the process; however, many materials fail to strike the necessary balance, leaving researchers to search for that elusive ideal.
Some challenges in this realm include:
- Sensitivity to Light: The resists' sensitivity is a double-edged sword; while high sensitivity promotes fine resolution, it can also lead to performance issues like unwanted exposure during the lithography process.
- Thermal Stability: Many substrates can warp or degrade when exposed to the heat required for processing, directly affecting the precision of the end product.
Moreover, the quest for new materials that can effectively meet these stringent requirements consumes substantial resources and time. There's an ongoing search to discover innovative substances, yet these endeavors are often fraught with trial and error. Emphasizing collaboration among researchers could amplify the pace at which breakthroughs occur.
"The driving force behind overcoming these challenges lies in our ability to innovate and adapt, recognizing the complexity of materials only enhances our pursuit of excellence in nanolithography."
Future Directions of Nanolithography
Nanolithography stands at a crucial juncture where innovation meets necessity. As we look ahead, it’s evident that the future of this technology does not only hinge on advancements in technique but also on the identification of new applications that can leverage these advancements for practical uses. Understanding these forward-looking developments is key for students, researchers, and professionals alike, as it shapes the trajectory of fields ranging from electronics to pharmaceuticals.
Emerging Technologies
The horizon of nanolithography is illuminated by several promising technologies that have the potential to reshape the industry. Among these, the rise of plateaued materials is significant. Materials that can maintain their integrity at nanoscales while allowing flexibility enable the production of devices that were previously impractical. This is particularly useful in flexible electronics, where lightness and portability are essentials.
Multi-beam lithography is another exciting development. By using multiple electron beams, this method not only speeds up the process of lithography but also enhances precision. The synergy of speed and accuracy is a game-changer—especially in semiconductor manufacturing—where the demands for greater efficiency continue to escalate.
Moreover, machine learning algorithms are making their way into the nanolithography landscape. These smart technologies can optimize processes based on accumulated data, predicting the best conditions for material deposition and pattern transfer. It’s not just about creating patterns anymore but doing so in an intelligent way that optimizes time and resources.
Key aspects of these technologies include:
- Material innovations that support lower energy consumption.
- Enhanced precision through new imaging techniques.
- Integration with artificial intelligence for predictive accuracy.
To capture the essence of these developments, consider this thought:
"Innovation is the daughter of necessity and technology. In the realm of nanolithography, both are weaving an intricate tapestry of possibilities."
Potential Market Impact
The implications of the advancements in nanolithography stretch far beyond the confines of laboratories. The potential market impact is profound, as these technologies promise to revolutionize multiple sectors.
In consumer electronics, for instance, the move towards smaller, faster, and more efficient devices is constant. With improved nanolithographic techniques, manufacturers can produce complex circuits with unprecedented ease. This leads to gadgets that not only operate faster but are also more energy-efficient, aligning well with global sustainability goals.
Beyond electronics, the medical field is poised to benefit significantly. Nanolithography can aid in producing nanoscale drug delivery systems that target specific cells, minimizing side effects and maximising efficacy. The intersection between technology and healthcare is offering ground-breaking solutions to some of today’s most pressing health challenges.
Key Market Considerations:
- The green technologies sector, leveraging sustainable materials through nanolithography.
- Enhanced capabilities for fabricating components in biotech sectors, influencing drug formulation and delivery.
- Potential for growth in educational markets as more institutions adopt advanced nanotechnology curricula.
In summary, as these emerging technologies catalyze new market realities, both opportunities and challenges will need to be carefully navigated. Understanding the trajectory of nanolithography is essential as it forges the future of multiple industries.
As the landscape shifts, it invites collaborations between disciplines—engineering, materials science, and computational analysis—while fostering a spirit of creativity and challenge that is vital for continued advancements.
Concluding Remarks
As we draw to a close on our exploration of nanolithography, it becomes increasingly clear that this field stands at the nexus of advancement in technology and science. The intricate processes and various techniques presented highlight not only the potential of nanolithography but also its practical implications across diverse industries. Understanding these nuances is crucial for students, researchers, and professionals alike, as they navigate the evolving landscape of nanotechnology.
Summarizing Key Points
Throughout this discussion, several key aspects come to the forefront:
- Diverse Techniques: We've examined multiple methodologies, including photolithography, electron beam lithography, and nanoimprint lithography. Each approach comes with unique advantages and limitations, catering to different applications.
- Material Selection: The choice of resists and substrate materials is essential. Their properties directly impact the efficacy and reliability of the nanolithographic process.
- Real-world Applications: The significance of nanolithography extends beyond theoretical implications. Its role in semiconductor manufacturing, nanomedicine, and flexible electronics showcases the transformative potential of this technology.
- Challenges and Future Directions: The ongoing obstacles in cost, scalability, and material constraints shed light on the vibrant thread between challenge and opportunity, guiding future innovations in the field.
Collectively, these points offer a comprehensive overview of how nanolithography is not just a technical endeavor but a cornerstone of modern science and industry.
The Role of Nanolithography in Modern Science
Nanolithography plays a critical role in various scientific and technological realms. The ability to create patterns at the nanoscale has fueled innovations in:
- Semiconductors: As devices shrink and power density increases, nanolithography allows manufacturers to produce the next generation of chips, vital for computing and data processing.
- Nanomedicine: Tailoring drug delivery systems and creating nanoscale diagnostic tools hinge upon the precision that nanolithography provides. This has profound implications for personalized medicine.
- Energy Solutions: In renewable energy technologies, nanolithographic techniques can assist in the development of efficient solar cells and improved battery systems.
- Sensors: Nanolithography enables the creation of highly sensitive sensors that can detect even minuscule changes in environmental conditions.
In summary, the ability of nanolithography to influence various scientific disciplines underlines its significance in today's world. Embracing its implications encourages further exploration and innovation, solidifying the foundation upon which future breakthroughs can be built.