Comprehensive Insights into Carbon Capture and Storage
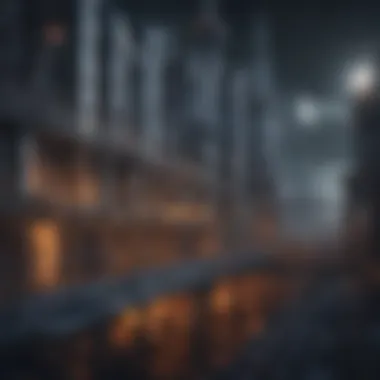
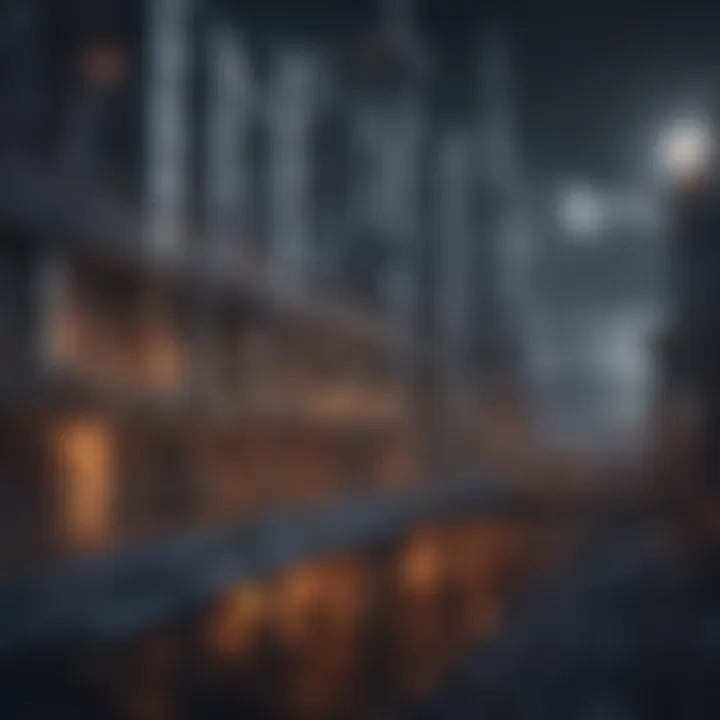
Intro
In the context of climate change, carbon capture and storage (CCS) has emerged as a crucial technology aimed at reducing greenhouse gas emissions. As carbon dioxide (CO2) levels in the atmosphere continue to rise, understanding the dynamics of CCS becomes increasingly important for both researchers and policymakers. CCS encompasses a range of processes and technologies designed to capture CO2 emissions from sources like power plants, transport them to a storage location, and sequester them underground or utilize them in various applications.
This article provides an in-depth examination of CCS, including theoretical frameworks, current methodologies, practical applications, and associated challenges. The importance of CCS lies not only in its potential contribution to climate change mitigation, but also in its relevance as part of broader environmental policies and strategies aimed at achieving sustainability.
By diving deep into the intricate details of CCS, including case studies and future trends, this article aims to equip readers with comprehensive insights that will foster a better understanding of the potential and the limitations of this technology as a response to global warming.
Research Overview
Summary of key findings
The exploration of carbon capture and storage has yielded several significant findings:
- Effectiveness of Technologies: Various CCS techniques, including pre-combustion capture, post-combustion capture, and oxy-combustion, have demonstrated differing degrees of effectiveness in capturing CO2.
- Cost Considerations: Implementing CCS can be costly, influenced by factors such as technology choice, location, and scale of operations.
- Global Case Studies: Successful CCS projects, such as the Sleipner project in Norway and the Gorgon project in Australia, provide valuable lessons on best practices and operational challenges.
Importance of the research in its respective field
Research on CCS is vital for several reasons:
- Climate Policy Alignment: Effective CCS technologies can align with international agreements, including the Paris Agreement, aimed at limiting global warming.
- Economic Impacts: A well-implemented CCS approach may create economic opportunities in sectors related to energy production and CO2 utilization.
- Sustainable Development: CCS is viewed as a critical pathway to achieving sustainable development goals by mitigating emissions while allowing economic growth.
Methodology
Description of the experimental or analytical methods used
The analysis of CCS primarily involves:
- Literature Review: Comprehensive review of existing studies, reports, and case studies about CCS technologies.
- Quantitative Analysis: Assessment of CO2 capture rates, costs, and operational efficiencies in various projects throughout the world.
Sampling criteria and data collection techniques
Data for this analysis was gathered from multiple sources:
- Case Studies: Carefully selected based on their prominence and relevance in the field of CCS.
- Academic Publications: Peer-reviewed articles, theses, and reports that provide empirical data on CCS methodologies and outcomes.
โThe challenge of climate change requires innovative solutions like carbon capture and storage to ensure a sustainable future.โ
Preamble to Carbon Capture and Storage
Carbon capture and storage (CCS) represents a pivotal advancement in our response to climate change. The importance of this technology stems from its capacity to reduce greenhouse gas emissions from multiple sectors. As global temperatures rise, the urgency to manage carbon dioxide levels in our atmosphere intensifies. CCS not only serves to capture emissions at their source, but it also holds potential for storage and even utilization in various applications. This multi-faceted approach is what makes CCS a significant topic of discussion in contemporary climate discourse.
Itโs essential to understand the key elements of carbon capture and storage to appreciate its relevance. CCS consists of processes that involve the capture of CO2, its transportation, and ultimately its storage underground or even its conversion into useful products. One primary benefit is that it allows continued reliance on fossil fuels while reducing their environmental impact. This capability positions CCS at the intersection of energy production and sustainability strategies.
However, consideration of CCSโs benefits must include thoughtful dialogue about its limitations and challenges. Economic factors, technological readiness, and public perception play critical roles in the deployment of CCS initiatives. By engaging with these dimensions, stakeholders can better assess the viability and future of CCS as part of a more extensive climate strategy.
"The investment in carbon capture is not just a technological fix; it is also a strategic necessity for achieving our climate targets while managing energy demands."
Ultimately, this section sets the stage for a deeper exploration of carbon capture and storage. With an understanding of its definitions and historical context, we can navigate through the mechanisms, applications, and implications of CCS more effectively.
Defining Carbon Capture and Storage
Carbon capture and storage is a process designed to capture CO2 emissions produced by industrial facilities, power generation plants, and various other sources. The captured CO2 is then transported and stored to prevent it from entering the atmosphere. The components of CCS mainly consist of three stages:
- Capture: This is the first step where CO2 is separated from other gases produced during industrial processes. Various technologies exist for capturing carbon, each suitable for different sources and conditions.
- Transportation: Post-capture, CO2 needs a method of transport to reach the storage site. This can involve pipelines, ships, or trucks, depending on the distance and quantity of CO2.
- Storage: Safe storage sites are crucial. CO2 can be injected into geological formations, such as depleted oil and gas fields or deep saline reservoirs, where it can be monitored over time.
This definition frames a fundamental understanding of CCS and introduces the technical language surrounding the topic.
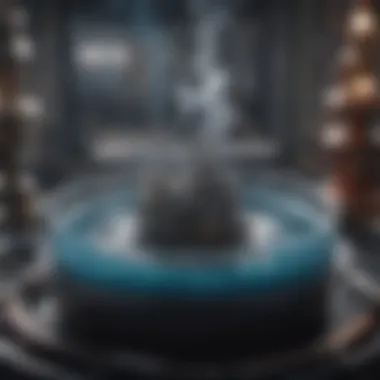
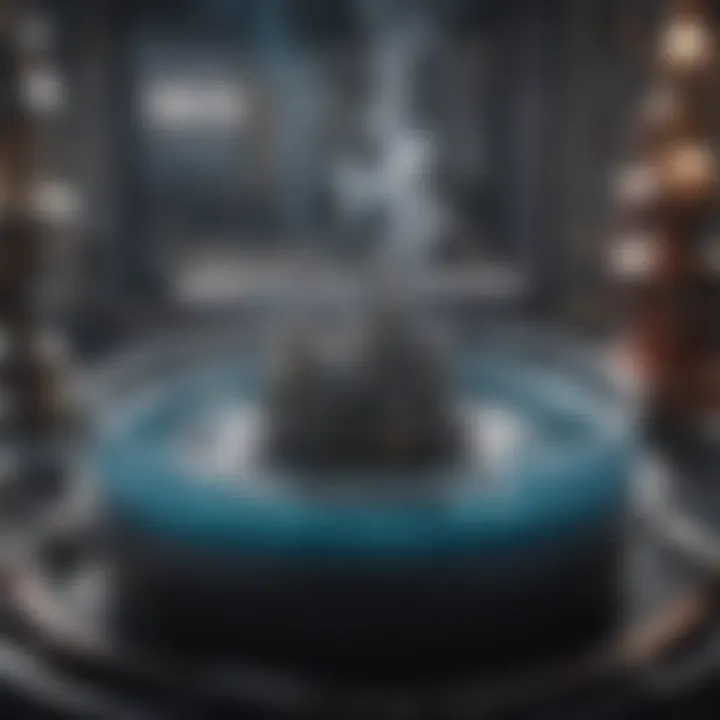
Historical Context of CCS Development
The development of carbon capture and storage can be traced back several decades. Early applications of CCS technology began in the 1970s, often linked with enhanced oil recovery projects. These initial projects provided a dual benefit: capturing CO2 while simultaneously increasing oil extraction efficiency.
The real push for CCS, however, gained prominence with the adoption of international climate agreements in the 1990s. The Kyoto Protocol, established in 1997, led to increased research funding and regulatory frameworks that spurred further exploration of CCS technologies. By the early 2000s, numerous pilot projects were operational globally, illustrating the feasibility of large-scale application.
Many landmark projects like the Sleipner Project in Norway, initiated in 1996, showcased both the technical viability and environmental benefits of CCS. Despite this progress, the pace of implementation faced hurdles related to regulatory policies, investment challenges, and public perception. Today, CCS remains essential in discussions about achieving net-zero emissions and aligns with various countries' climate action plans.
Mechanisms of Carbon Capture
Carbon capture plays a crucial part in combating climate change. By capturing carbon dioxide emissions, we aim to prevent them from entering the atmosphere and contributing to global warming. Understanding the mechanisms of carbon capture is essential for researching its effectiveness and practical applications. The methods involved in carbon capture can be broadly categorized, each having distinct mechanisms, benefits, and challenges.
The Science of Carbon Capture
Carbon capture technology primarily aims at isolating CO2 from industrial processes or atmospheric sources. This is achieved through several scientific principles, which include absorption, adsorption, and membrane separation.
- Absorption: This method involves using a liquid solvent to chemically react with CO2. Common solvents are amines, which react with CO2 to form a compound that can later be heated to release pure CO2.
- Adsorption: In this process, CO2 molecules adhere to the surface of solid materials, known as adsorbents. This method is highly efficient but often requires regeneration cycles to release captured CO2.
- Membrane Separation: This technique utilizes selective permeable membranes to separate CO2 from other gases, such as nitrogen. Membranes can selectively allow CO2 to pass through while blocking other components, making it an effective method.
Each of these methods presents its own advantages and limitations, and ongoing research aims to optimize these processes for enhanced efficiency.
Direct Air Capture Technologies
Direct air capture (DAC) technologies are among the most innovative in the field of carbon capture. Unlike traditional methods that target emissions directly from industrial sources, DAC captures CO2 from ambient air. This is crucial for achieving negative emissions targets. DAC systems can vary in design but generally involve similar core processes.
- Air Contacting: DAC systems utilize fans to draw in ambient air to a capture unit, where the air comes in contact with a CO2 absorbent material.
- CO2 Release: After the CO2 is absorbed, it is then released from the material through a heating process. The captured CO2 can either be utilized directly or stored.
- Utilization or Storage: Captured CO2 can be used for various applications, including in the production of synthetic fuels or geological storage.
Direct air capture provides flexibility and has the potential to address legacy emissions effectively, but it remains energy-intensive and costly.
Post-Combustion Capture Techniques
Post-combustion carbon capture techniques target CO2 emissions as they arise from burning fossil fuels. This is crucial for industries like power generation and heavy manufacturing. The processes generally include the following steps:
- Flue Gas Treatment: The flue gases produced during combustion, which contain CO2 along with other gases, are cooled and treated to remove pollutants.
- Capture Process: The treated flue gas is then passed through a capture unit using either absorption or adsorption methods to isolate the CO2.
- Compression and Transport: Once captured, CO2 is compressed for transportation, either for storage or utilization.
Post-combustion techniques have shown promise in reducing emissions significantly, but they require upfront investments in infrastructure and can also face operational challenges.
Storage Mechanisms for Captured Carbon
Storage mechanisms for captured carbon play a pivotal role in the usability and efficiency of carbon capture and storage (CCS) technologies. The significance of these mechanisms lies in ensuring that the carbon dioxide captured from various sources does not re-enter the atmosphere but is instead sequestered safely and securely. This section delves into the available strategies for storing CO2, focusing on both geological and alternative utilization methods, all of which present unique benefits and considerations.
Geological Storage Options
Geological storage involves placing captured CO2 deep underground in suitable geological formations. This method is one of the most studied and widely practiced approaches in CCS. The geological formations can include depleted oil and gas reservoirs, saline aquifers, and unmineable coal seams. These sites have characteristics that allow them to securely contain carbon dioxide.
The importance of geological storage is multifaceted:
- Long-term storage: Geological formations offer the potential for long-term containment of CO2, potentially for thousands of years. This durability is crucial in the fight against climate change.
- Safety and integrity: The deep underground settings are often stable and monitored to prevent leakage, ensuring that the storage remains secure over time.
- Enhanced oil recovery: Some projects utilize CO2 for enhanced oil recovery (EOR) in depleted oil fields. Injecting CO2 can increase extraction efficiency while simultaneously sequestering CO2, making it economically viable.
However, several challenges remain:
- Site selection: Identifying suitable sites requires significant geological surveying and risk assessments.
- Monitoring and verification: Continuous monitoring is essential to ensure that stored CO2 does not leak, adding to the operational costs.
"The successful implementation of geological storage is vital to achieving global climate goals, providing a dual function of emissions reduction and energy resource recovery."
Alternative Utilization Methods
Aside from geological storage, alternative utilization methods present innovative ways to repurpose captured carbon dioxide. Carbon capture utilization (CCU) emphasizes the use of CO2 as a raw material for various industrial applications. This approach can enhance economic feasibility while also providing environmental benefits.
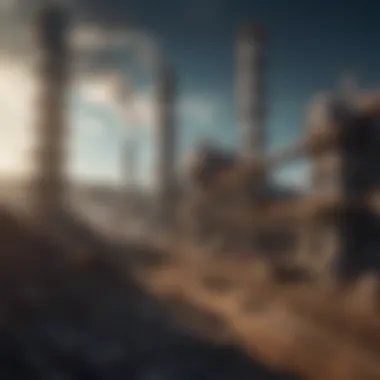
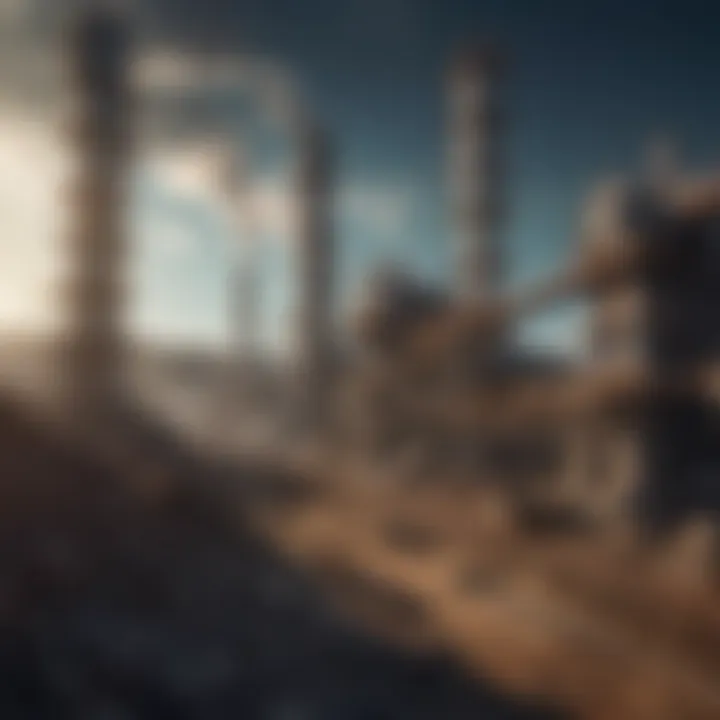
Key applications of CO2 utilization include:
- Chemical production: CO2 can be used to produce chemicals like methanol, which can be utilized in fuel production and other industries.
- Materials: Innovations in construction technologies allow for the incorporation of CO2 into materials, such as concrete. This not only sequesters CO2 but can also enhance the strength and durability of the materials.
- Agriculture: Captured CO2 can be employed in greenhouses to promote plant growth. Increased carbon availability can improve crop yields under controlled environments.
Despite these prospects, the alternative utilization of CO2 comes with its challenges:
- Economic viability: Many CCU processes are still not cost-competitive with traditional methods, requiring advancements in technology and reductions in costs.
- Scale of operations: For true impact, utilization methods need to be adopted on a large scale, which may require significant infrastructure investments.
The Role of CCS in Climate Change Mitigation
Carbon Capture and Storage (CCS) plays a critical role in the broader narrative of climate change mitigation. As global temperatures rise, the urgency for effective solutions has intensified. CCS serves as a key technology in reducing greenhouse gas emissions, particularly carbon dioxide, from industrial sources. This function positions CCS not only as an innovative approach but as an essential component in achieving significant environmental goals.
CCS and Global Climate Goals
Understanding the alignment of CCS with global climate goals is crucial. The Paris Agreement established targets to limit global warming to well below 2 degrees Celsius, aiming for a maximum of 1.5 degrees Celsius. Meeting these targets necessitates substantial reductions in CO2 emissions. CCS can account for approximately 15% of the needed mitigation measures by 2050. This makes it an invaluable ally to renewable energy strategies and energy efficiency improvements.
Governments and organizations acknowledge the potential of CCS to transform heavily polluting sectors, including cement and steel production. By integrating CCS, these industries can drastically lower their carbon footprint while maintaining production levels. Countries like Norway and the Netherlands have already incorporated CCS into their climate targets, illustrating its growing prominence within global strategies. Successful deployment of CCS is tied to advancements in technology, investment initiatives, and supportive regulatory frameworks.
Comparative Analysis with Other Emission Reduction Strategies
While CCS is influential, it is vital to compare it with other emission reduction strategies. Renewable energy, energy efficiency, and carbon pricing are also significant. Each strategy contributes uniquely to lowering emissions. However, one limitation of renewables is their dependency on consistent energy sources, which may not always align with demand. CCS can complement renewables by providing a steady energy supply from fossil fuels while capturing emissions.
When examining energy efficiency, it is essential to recognize that improving efficiency alone may not meet the ambitious targets imposed by climate agreements. In many cases, industries must still rely on fossil fuels. CCS offers a transitional solution, enabling stewardship of existing fossil fuel usage until more comprehensive clean alternatives fully materialize.
Furthermore, carbon pricing mechanisms incentivize businesses to reduce emissions; yet, they may have limitations without a concrete plan for capturing unavoidable emissions. Thus, the integration of CCS can enhance the effectiveness of carbon pricing strategies.
Challenges in Implementing CCS
Carbon capture and storage (CCS) stands out as a potential tool in mitigating climate change. However, multiple challenges are faced in its implementation. Understanding these barriers is crucial for developing strategies that enhance CCS adoption. Each challenge represents not only an obstacle but also an opportunity for innovation and problem-solving.
Economic Barriers to Adoption
The economic landscape surrounding CCS is complex. The capital costs associated with CCS technologies are significant. Building the necessary infrastructure requires substantial investment. For instance, the development of capturing facilities, transport pipelines, and storage sites involves multi-million dollar budgets.
- Uncertain Financial Returns: There's often uncertainty in financial returns due to fluctuating carbon prices and government policies. Industries might hesitate to invest in CCS if the benefits do not clearly outweigh the expenses.
- Incentives Needed: Government incentives can help alleviate some of this burden. However, many regions still lack supportive policies that can stimulate further investment in CCS initiatives.
- Competition with Renewable Energy: Furthermore, the competition with renewable energy sources also makes CCS appear less attractive. As wind and solar technology becomes more affordable and accessible, CCS can seem like an outdated solution in the eyes of investors.
Technical Limitations and Risks
While CCS has advanced significantly, technical issues remain a concern. Engineers and scientists continue to face several limitations that hinder its efficiency and reliability.
- Capture Efficiency: The capture process itself can be energy-intensive, which decreases its overall effectiveness. Efforts are underway to improve efficiencies, but this remains a significant challenge.
- Storage Integrity: There are also concerns regarding the long-term integrity of storage sites. Unintended leaks can occur, leading to carbon dioxide emissions that would negate the progress made. Monitoring tech is vital to ensure secure storage, but this adds complexity and cost to the CCS projects.
- Scale for Impact: Additionally, scaling CCS to a level that can impact global emissions is daunting. Most current projects operate at a small scale compared to global needs, necessitating significant expansion in capacity and distribution.
Public Perception and Acceptance Issues
Public perception represents another key challenge for CCS. Without public buy-in, projects will struggle to move forward. Many individuals and communities have mixed feelings about CCS technologies.
- Awareness and Understanding: One obstacle is a general lack of awareness about what CCS entails. Many people are unfamiliar with the technology and its benefits. Campaigns aimed at educating the public are essential.
- Concerns about Safety: Public safety concerns around potential leaks or other environmental impacts can create resistance. Transparent communication about risks and safety measures is crucial in building trust.
- NIMBY Syndrome: The 'Not In My Back Yard' (NIMBY) phenomenon can also limit site selection for CCS plants. Communities may be supportive of climate initiatives, but oppose local installations due to fears about safety and changes to their environment.
Addressing these challenges is vital in ensuring the future viability of CCS. Through collaboration and innovation, solutions can emerge that benefit both the environment and economic growth.
Case Studies of Successful CCS Projects
Case studies of successful carbon capture and storage (CCS) projects illustrate the practical applications of CCS technology and its impacts on reducing carbon emissions. They offer tangible examples of how this technology has been implemented effectively in various contexts. These case studies help to highlight the potential of CCS to contribute meaningfully to climate change mitigation strategies. Evaluating these projects provides valuable insights into best practices, potential obstacles, and the importance of community and stakeholder engagement.
Notable Global Initiatives
Various countries have initiated notable CCS projects, showcasing different methodologies and applications of this important technology. Some key successful projects include:
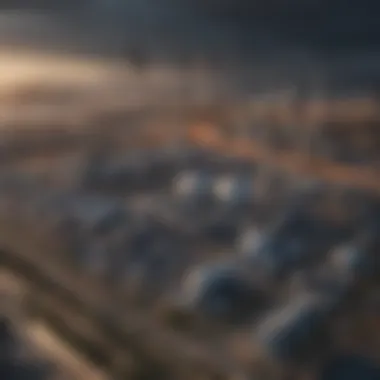
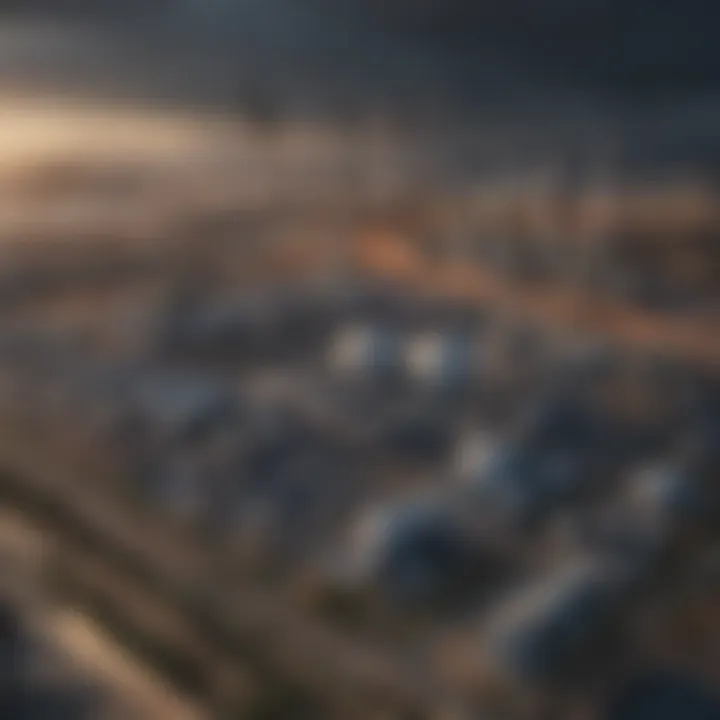
- Sleipner Project in Norway: One of the earliest CCS projects, operational since 1996, the Sleipner project captures CO2 from natural gas production and injects it into a geological formation deep beneath the North Sea. This project has facilitated a better understanding of subsurface storage dynamics and safety.
- Gorgon Project in Australia: This initiative aims to capture and store up to 4 million tons of CO2 each year. The Gorgon project integrates carbon capture as part of its natural gas extraction process, serving as a model for industrial CCS.
- Boundary Dam in Canada: This power plant retrofit project has significantly reduced CO2 emissions by capturing over a million tons of carbon dioxide annually. It is a landmark example of integrating CCS technology into existing fossil fuel power plants.
These projects demonstrate the adaptability of CCS technology across different industries and geographical contexts. They also showcase its scalability, marking CCS not just as a theoretical concept but as practical solution.
Lessons from Implementation
Learning from successful initiatives is key for future enhancements in CCS technology. Several critical lessons emerge from these case studies:
- Importance of Monitoring and Verification: Continuous monitoring is essential for ensuring that captured CO2 remains securely stored. Notable projects have implemented comprehensive monitoring programs to verify safety and efficacy, providing transparency and public confidence in CCS.
- Stakeholder Engagement: Active engagement with local communities and stakeholders is vital. Successful projects often involve dialogues that educate and incorporate community input, ensuring that concerns are addressed.
- Economic Viability: Financial feasibility is often a hurdle for CCS projects. Successful initiatives frequently rely on public-private partnerships and government incentives, suggesting pathways for future projects.
- Technology Integration: The best results come from projects combining CCS with existing infrastructure. This can lower capital costs and smooth the transition to lower emissions.
"Successful CCS exemplifies not just technological advancement but also the critical need for cross-sector collaboration and community involvement."
Overall, these case studies not only highlight the effectiveness of current CCS technologies but also provide a roadmap for future endeavors in the field. By dissecting real-world applications, stakeholders can better understand both the potential and the limitations of carbon capture and storage.
Future Perspectives on Carbon Capture and Storage
Future perspectives on carbon capture and storage (CCS) are critical for understanding how this technology can evolve and integrate into our global efforts to combat climate change. As demands for reducing greenhouse gas emissions intensify, innovative approaches in CCS will play a vital role in addressing environmental challenges. This section will explore several key areas that define the future landscape of CCS, including emerging technologies, the alignment with climate policies, and the significance of global cooperation.
Innovative Technologies on the Horizon
The landscape of carbon capture is rapidly changing, as new technologies emerge with potential advantages over conventional methods. Innovative advancements in materials science lead to the development of more efficient sorbents for absorbing CO2, essential to streamline the capture process. Technologies like membrane separation and bioenergy with carbon capture and storage (BECCS) are currently being researched for their ability to enhance overall efficiency.
- Membrane Technologies: Membranes designed to selectively allow CO2 to pass through while blocking other gases show promise in more efficient gas separation. Research in this area aims to reduce energy requirements and overall costs associated with the capture process.
- Machine Learning and AI: Advances in artificial intelligence can optimize operational parameters in CCS facilities. By analyzing large datasets, AI can enhance performance and predict maintenance needs, thus reducing downtime and elevating the sustainability of the capture processes.
- Innovative Chemical Processes: New chemistries, such as organic solvents and ionic liquids, are being explored to enhance CO2 absorption rates and regenerate quickly, minimizing the energy penalty often associated with carbon capture.
These technological developments create a backdrop of excitement and hope for the future of CCS, as they could significantly lower costs and enhance the scalability of carbon capture projects.
CCS within Emerging Climate Policies
CCS is increasingly being woven into global climate policies, proving itself as a necessary tool for meeting various climate goals. Many countries are setting ambitious targets to achieve net-zero emissions by mid-century, and CCS is seen as a crucial pathway to assist in reaching these targets. Governments and policymakers recognize the need for a diverse array of solutions in their climate strategies, where CCS holds a prominent position.
- Integration into National Commitments: Federal and international climate agreements often cite CCS as part of the strategy to reduce emissions, showcasing its importance in achieving commitments made under frameworks such as the Paris Agreement.
- Funding Initiatives: Several governments and institutions are increasing financial incentives for research and development of CCS technologies. These grants can accelerate innovations and facilitate projects that demonstrate the practical application of CCS.
- Regulatory Frameworks: Emerging guidelines are being formulated to support the expansion of CCS projects, including policies that clarify liability concerns for long-term storage and tax incentives for companies adopting these technologies.
Incorporating CCS within these climate policies signals a shift towards recognizing its role not just as a theoretical solution but as an actionable component of climate strategy.
Collaborative Efforts and Global Partnerships
Collaboration on a global scale is essential for the advancement of CCS technologies. The complex nature of climate change requires various stakeholders to work together, sharing knowledge, resources, and technologies.
- International Research Collaborations: Various organizations, including the International Energy Agency (IEA) and the Global CCS Institute, facilitate collaborative research and development initiatives. These partnerships allow for diverse perspectives and access to a broader range of funding opportunities.
- Public-Private Partnerships: Engaging the private sector through public-private partnerships encourages investment and innovation. By working together, firms can leverage each other's expertise and address challenges in technology development and implementation more effectively.
- Knowledge Exchange: By sharing successful case studies and best practices through forums or workshops, both developed and developing nations can learn from each other. Such exchanges pave the way for rapidly deploying CCS strategies across different regions globally.
Ultimately, forming robust partnerships enhances the ability to tackle the challenges associated with carbon capture, ensuring a more comprehensive and efficient approach to climate change mitigation.
"Carbon capture is not just a technology; it's a means to transform our energy systems and align them with our climate goals."
By focusing on these future perspectives of CCS, we can visualize a multifaceted approach to one of the most pressing issues of our time, engaging future innovations, policies, and collaborations that can make a significant impact.
The End and Recommendations
The final section of this article addresses the critical conclusion and recommendations regarding carbon capture and storage (CCS). In a world increasingly aware of the urgency to tackle climate change, CCS emerges as a pivotal technology. Its potential to significantly reduce greenhouse gas emissions is noteworthy. However, various challenges still surround its realization and optimization.
Summary of Key Findings
In reviewing the topic, several key findings stand out:
- Importance of CCS: Carbon capture and storage plays a crucial role in addressing climate change. It effectively sequesters carbon dioxide emissions from industrial sources before they enter the atmosphere.
- Technological Diversity: Various technologies, such as direct air capture and post-combustion capture, offer unique solutions to different sectors. Each method presents distinct advantages and constraints.
- Storage Options: Geological storage remains the most extensively researched method; however, alternative utilization methods may present economically viable opportunities.
- Economic and Societal Factors: Economic barriers and public misconceptions continue to inhibit widespread CCS adoption. Education and engagement are vital for stakeholders to understand the technology's importance.
- Global Collaboration: Successful CCS initiatives often rely on international partnerships, cross-sector collaboration, and shared research. This coordinated approach ensures the rapid advancement of CCS technologies and methodologies.
Path Forward for CCS Implementation
Moving forward, several recommendations can enhance the implementation and effectiveness of CCS. These include:
- Enhancing Research and Development: Continued investment in research will foster innovation and improve existing technologies. This investment must focus on reducing cost and increasing efficiency.
- Policy Support: Governments should establish clear policies and regulatory frameworks that incentivize CCS projects. Financial incentives, such as tax credits or grant programs, will stimulate investment from private sectors.
- Public Engagement and Education: Raising awareness among the public regarding the benefits of CCS will aid in overcoming skepticism. Clear communication and transparent dialogue are crucial.
- Demonstration Projects: Expansion of pilot projects will showcase the viability of CCS systems. By providing tangible examples of success, these initiatives can help build confidence in CCS technology.
- Global Partnerships: By fostering international cooperation, knowledge sharing can be streamlined, ultimately accelerating technological advancements in CCS. Joint ventures can mitigate financial risks associated with large-scale implementation, making projects more attractive to investors.
"Implementing carbon capture and storage successfully requires not just technological innovation but also broad societal acceptance and industrial commitment."