Understanding Soil Bioremediation Techniques and Processes
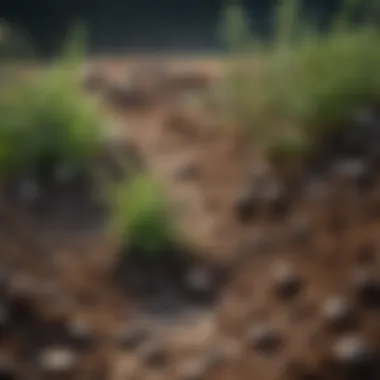
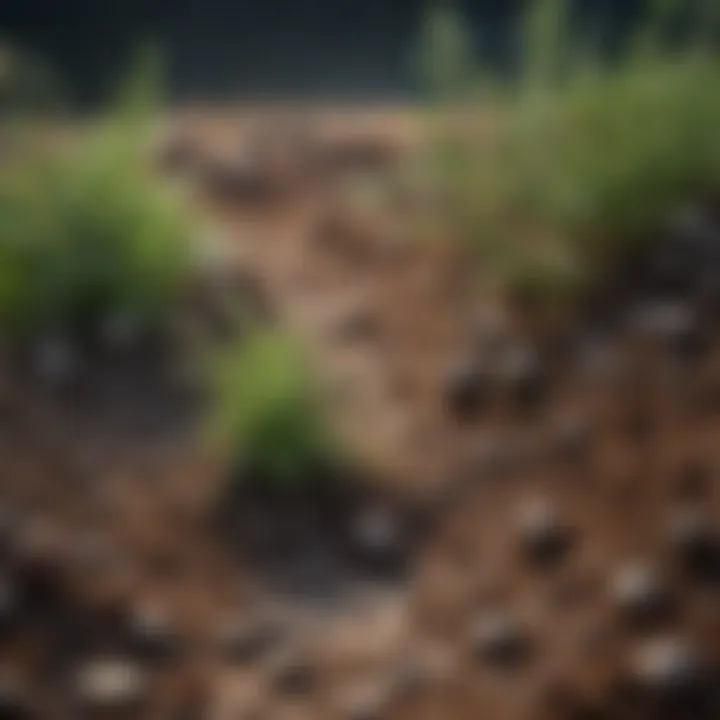
Intro
The soil beneath our feet often holds the key to a thriving ecosystem. Yet, due to various human activities, this essential resource can become tainted, leading to dire consequences for both the environment and public health. Bioremediation emerges as a vital practice, harnessing the power of living organisms to cleanse contaminated soils. By exploring this fascinating subject, we aim to shed light on the intricate processes through which microorganisms and plants collaborate to restore soil health.
Through this article, readers will uncover the remarkably dynamic field of soil bioremediation. Not only will we delve into the mechanisms employed by different biological agents, but we will also examine the practical applications, challenges faced, and the exciting innovations reshaping sustainability efforts.
Research Overview
Summary of Key Findings
The extensive body of research in soil bioremediation reveals multiple pathways through which contaminants can be effectively degraded. There are two primary approaches typically utilized: in situ and ex situ bioremediation.
- In situ bioremediation: This method involves treating contaminated soil without removing it. Microorganisms native to the site are often harnessed alongside conditions modified to enhance their growth and activity. For example, adding nutrients or oxygen can significantly accelerate the degradation of pollutants.
- Ex situ bioremediation: In contrast, this technique requires the removal of contaminated soil to a controlled environment. This approach allows for meticulous monitoring and can lead to quicker results, albeit at a higher cost and with a greater ecological footprint.
An important breakthrough highlighted in recent studies shows how hybrid systems—integrating both phytoremediation and microbial techniques—can lead to enhanced efficiency and remediation rates. These findings indicate the potential of combining plant roots that uptake pollutants with microbes that break them down.
Importance of the Research in Its Respective Field
Understanding the processes and methodologies associated with soil bioremediation is pivotal in environmental science. As industrial practices continue to generate waste, mitigating hazardous substances becomes paramount. Therefore, research in this domain plays a crucial role in:
- Restoring ecosystems already stressed by pollution.
- Advancing innovations in sustainable practices that mitigate future contamination.
- Guiding policies and regulations regarding environmental stewardship.
This research not only feeds into the broader tapestry of environmental recovery efforts but also champions the notion that nature can indeed heal itself if given the right tools and support.
Methodology
Description of the Experimental or Analytical Methods Used
To facilitate a deeper understanding of soil bioremediation, researchers typically engage a variety of experimental methods. These may encompass:
- Field studies observing bioremediation in natural settings.
- Laboratory-based experiments testing specific organisms against selected contaminants.
- Modeling techniques simulating biological interactions and degradation pathways.
By employing a multifaceted approach, the research can elucidate which organisms or conditions yield the most successful outcomes in different scenarios.
Sampling Criteria and Data Collection Techniques
The collection of samples for study is critical and usually follows strict guidelines. Key criteria might include:
- Contaminant type and concentration in the soil.
- Environmental factors such as pH, moisture levels, and nutrient availability.
- The presence of specific microbial populations or plant species.
Data collection often involves both quantitative metrics, such as rates of pollutant degradation, and qualitative observations, including soil health indicators. This comprehensive data helps researchers discern efficacies of various bioremediation strategies while also identifying new opportunities for enhancement.
"Bioremediation can serve as a bridge, connecting humans back to the natural solutions environment has crafted over millennia."
As the story of soil health unfolds, the contributions of bioremediation take on a broader significance, reminding us that our ecological future relies on understanding and utilizing these natural processes.
Preface to Bioremediation
Understanding bioremediation is crucial in today’s world, where soil contamination poses significant challenges to health and sustainability. The degradation of pollutants through biological processes stands at the forefront of environmental restoration strategies. By using the natural abilities of microorganisms and plants, we can work towards reversing the damage caused by pollutants, making bioremediation not just a useful technique but a key player in our environmental recovery strategy.
Bioremediation offers several important benefits:
- Cost-Effectiveness: Compared to traditional remediation methods, biological processes often require less investment and can be implemented on-site.
- Sustainability: Utilizing natural organisms for cleanup minimizes the environmental footprint of remediation processes.
- Versatility: Bioremediation can be applied to diverse soil contaminations, from agricultural pollutants to industrial waste.
While the advantages are compelling, several considerations come into play. Factors such as soil conditions, the nature of contaminants, and the microbial community present, all influence the effectiveness of bioremediation. Thus, understanding these dynamics is pivotal for a successful bioremediation process. In this section, we will sketch a clearer picture of bioremediation, starting with its definition and historical background.
Defining Bioremediation
Bioremediation can be defined as the process of using living organisms, primarily microorganisms, to degrade or detoxify contaminants in soil and other environments. This process not only aims to remove hazardous substances but also restores the ecosystem’s natural ability to function.
The key players in this process are local bacteria, fungi, and even plants, which interact with pollutants, breaking them down into less harmful substances. There are two primary methods of bioremediation: in situ, where remediation occurs at the site of contamination, and ex situ, where contaminated soil is removed and treated elsewhere.
In essence, bioremediation taps into nature's own capabilities to enable our recovery from human-induced environmental impacts, hence redefining remediation strategies.
Historical Context
The concept of using biological processes to clean up contaminated environments isn’t new but has deep historical roots. Early practices can be traced back to ancient civilizations that unknowingly engaged in forms of bioremediation by planting specific vegetation to prevent soil erosion or cultivate crops that could restore soil fertility.
However, the modern understanding of bioremediation emerged during the latter part of the 20th century, particularly in the wake of several environmental disasters. For instance, the Exxon Valdez oil spill in 1989 became a turning point, showcasing the potential of microorganisms in breaking down hydrocarbons. This event highlighted the urgency for effective restoration methods, which led to a surge in research focused on enhancing microbial capabilities and understanding their ecologies.
The historical context of bioremediation underpins its evolution into a sophisticated science where researchers work tirelessly to optimize microbial strains and techniques. Today, we stand at a promising juncture of technology and nature, working hand-in-hand to heal our battered environments.
Types of Soil Contaminants
Understanding the various types of soil contaminants is crucial to effectively addressing soil pollution through bioremediation techniques. Different contaminants pose unique challenges and require distinct approaches for removal or degradation. The presence of these contaminants not only affects the ecological balance but can also have detrimental impacts on human health. By identifying the types of soil pollutants, we can gain insight into how they interact with different bioremediation agents, ultimately paving the way for more effective restoration strategies.
Organic Pollutants
Organic pollutants are primarily derived from human activities, often stemming from industrial processes, agricultural runoff, or improper disposal of waste. These compounds include a wide range of substances such as hydrocarbons, solvents, and pharmaceuticals. One notable example is oils and grease, which can dramatically alter soil structure and inhibit the growth of plants and microorganisms.
These compounds can be particularly stubborn, as they may resist degradation under natural conditions. However, certain microorganisms possess the ability to metabolize these pollutants, breaking them down into harmless byproducts. For instance, bacteria such as Pseudomonas aeruginosa have been known to effectively degrade aromatic hydrocarbons found in oil spills. This is where the beauty of bioremediation shines, as it harnesses the natural capabilities of life to restore contaminated environments.
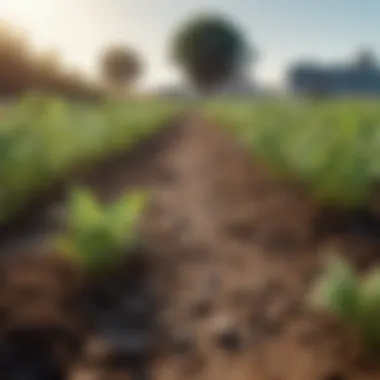
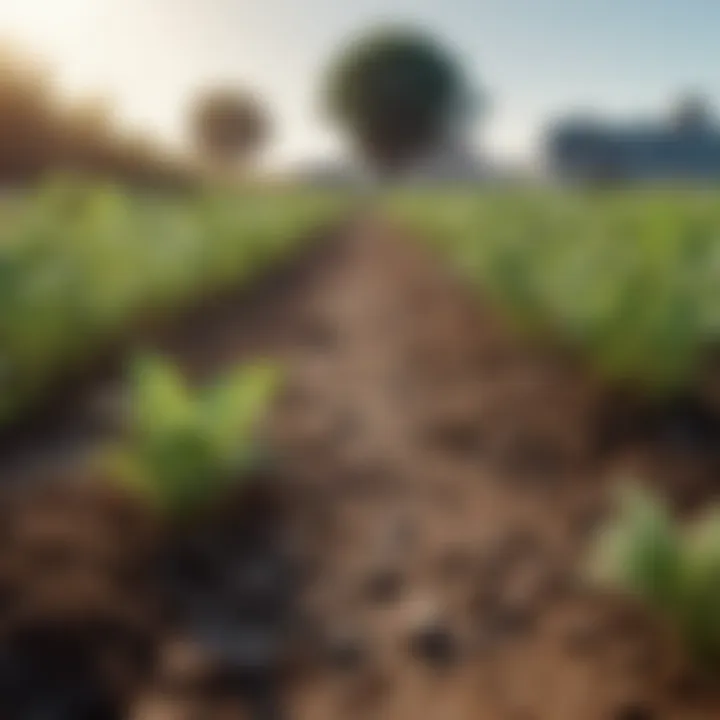
Heavy Metals
Heavy metals represent another class of soil contaminants that poses significant risks to both health and the environment. Substances such as lead, cadmium, and arsenic often find their way into soil through improper waste management, mining activities, or atmospheric deposition from industrial processes. Unlike organic pollutants, heavy metals do not degrade, which makes their persistence in the environment a crucial concern.
The challenges posed by heavy metals extend beyond their toxicity; they can accumulate in the food chain, resulting in long-term implications for animal and human health. Some plants and microorganisms have developed mechanisms to tolerate or even uptake these metals, offering potential avenues for bioremediation. For instance, hyperaccumulator plants like Thlaspi caerulescens can extract and store heavy metals effectively, making them excellent candidates for phytoremediation strategies.
Pesticides and Herbicides
Pesticides and herbicides are another significant group of soil contaminants. These chemicals are widely used in agriculture to manage pests and promote crop production, but their residual presence in soil can lead to various ecological disturbances. For example, the herbicide glyphosate has been identified for its potential to disrupt microbial communities, affecting soil health and biodiversity.
The behavior and degradation of these substances in soil can be influenced by several factors, including soil composition and environmental conditions. Certain microorganisms have evolved to metabolize these compounds, often doing so more efficiently than their natural counterparts, thus offering solutions for bioremediation. Understanding their degradation pathways is essential, as it paves the way for developing effective bioremediation strategies tailored to specific pesticides and herbicides.
In summary, the recognition and understanding of diverse soil contaminants are pivotal for developing effective bioremediation strategies. Each category presents unique challenges but also opportunities for harnessing biological processes to cleanse our soils.
Mechanisms of Bioremediation
Understanding the mechanisms of bioremediation is like peeling back the layers of an onion. Each layer reveals the intricate processes that not only elucidate how biological agents tackle soil contamination, but also highlight the potential benefits for restoring ecosystems and enhancing soil quality. This section delves into two primary mechanisms: microbial metabolism and phytoremediation strategies, both pivotal in employing natural processes to address pollution.
Microbial Metabolism
Microbial metabolism is essentially the powerhouse of bioremediation. This process involves the breakdown of contaminants by microorganisms, primarily bacteria and fungi, which can convert hazardous substances into less harmful forms. It’s important to grasp that microbes are not just passive players; they actively alter their environment to facilitate decomposition.
For instance, some bacteria utilize hydrocarbons, commonly found in oil spills, as a source of energy. These organisms metabolize the compounds, transforming them into harmless byproducts. Consider the remarkable versatility of a bacterium like Pseudomonas aeruginosa. This species can thrive in environments laden with pollutants, breaking down complex compounds into simpler, non-toxic forms.
The effectiveness of microbial metabolism hinges on several factors:
- Nutrient Availability: Microbes require nutrients such as nitrogen and phosphorus to grow and thrive.
- Environmental Conditions: Factors like temperature, pH, and moisture levels directly affect microbial activity.
- Oxygen Levels: Aerobic conditions often enhance metabolism, while anaerobic conditions might see different metabolic pathways activated.
In practical applications, biostimulation or bioaugmentation can be utilized to enhance microbial activity. These methods involve either adding nutrients or introducing microbial strains into contaminated sites, essentially giving nature a helping hand. The beauty of microbial metabolism is its adaptability, allowing for a broad range of applications in various environmental contexts.
Phytoremediation Strategies
While microorganisms are the unsung heroes below ground, plants play a crucial role above. Phytoremediation refers to the technique whereby plants absorb, degrade, or stabilize pollutants from the soil. This method can be remarkably effective, capitalizing on the natural processes of plant growth.
Different plants have varying abilities to remediate different types of contaminants. For example, willows and poplars are known for their ability to absorb heavy metals, while Sunflowers have shown efficacy in dealing with radioactive contaminants. The process can be broken down into several key strategies:
- Phytoextraction: Plants absorb pollutants through their roots and accumulate them in their stems and leaves.
- Phytostabilization: Here, plants contain contaminants in the soil, preventing them from leaching into groundwater.
- Phytodegradation: In this strategy, plants transform toxic substances into less harmful ones through metabolic processes.
A remarkable instance of successful phytoremediation occurred at a former manufacturing site in Canada, where Brassica napus (rapeseed) was employed to reduce levels of heavy metals in the soil. This approach not only cleaned the environment but also offered ancillary benefits, such as landscape restoration and additional plant biomass.
In essence, the mechanisms of bioremediation intertwine microbial metabolism's efficiency with the ecological prowess of plants. The collaboration between these two processes can lead to a holistic approach to soil restoration, addressing pollution in a sustainable manner that benefits both the environment and human health.
"Nature does not hurry, yet everything is accomplished." — Lao Tzu
In summary, recognizing the significance of both microbial metabolism and phytoremediation strategies lays the groundwork for broader understanding and effective application in the field of soil bioremediation. As we delve deeper into this realm, it's imperative to consider the context in which these methods are applied, paving the way for innovative solutions in soil health restoration.
Microorganisms Used in Bioremediation
In the ever-growing quest to rehabilitate our polluted soils, microorganisms stand at the forefront as both unsung heroes and indispensable tools in the bioremediation process. These microscopic organisms are nature's recyclers, playing a critical role in breaking down environmental contaminants while contributing to soil health and stability. Understanding their various types and mechanisms is essential for developing effective bioremediation strategies.
Microorganisms such as bacteria, fungi, and algae each offer unique benefits and capabilities that can be harnessed to address different types of soil pollution. Their inherent ability to adapt and thrive in various environmental conditions makes them highly versatile agents in cleaning up polluted sites.
Bacteria
Bacteria form the backbone of bioremediation efforts. Their sheer abundance in nearly every ecosystem and their varied metabolic pathways allow them to degrade a wide array of organic and inorganic contaminants. Key species, like Pseudomonas, Bacillus, and Mycobacterium, have shown remarkable effectiveness in breaking down hydrocarbons, solvents, and other pollutants. The versatility of bacteria lies in their ability to utilize various energy sources, which enables them to survive in adverse conditions.
One of the major benefits of using bacteria in bioremediation is their rapid reproduction rate. Under optimal conditions, certain strains can double their population in less than an hour. This rapid growth is crucial for scaling up bioremediation efforts and producing significant results in a timely manner. However, the success of bacteria-based remediation hinges on environmental conditions, such as temperature, pH, and nutrient availability.
Fungi
Fungi, though often overshadowed by their bacterial counterparts, offer powerful bioremediation capabilities that should not be overlooked. Their extensive mycelial networks can break down complex organic pollutants, such as wood preservatives and pesticides, through processes known as ligninolytic and cellulolytic degradation. Fungi like Phanerochaete chrysosporium, known for its ability to break down lignin, have been studied extensively for their role in enhancing soil bioremediation.
The mycelia can also bind to metals and some organic pollutants, which aids in immobilizing toxic substances and preventing their spread. This property makes fungi particularly effective in areas where heavy metals and recalcitrant organic compounds coexist. While their degradation processes might be slower than those of bacteria, the resilience of fungi in harsh conditions lends them an important place in soil restoration.
Algae
Algae are gaining recognition in the bioremediation of soils due to their ability to absorb nutrients and heavy metals from contaminated environments. Their fast growth rates and the capability to photosynthesize allow them to thrive even in environments deficient in organic matter. For instance, Chlorella and Spirulina spp. are two examples of algae that have been successfully employed to remediate nutrient-rich runoff and heavy metal contamination.
Additionally, algae can produce biofuels and other valuable byproducts during the remediation process, making them an attractive option from both an environmental and economic perspective. Furthermore, algae are capable of enhancing soil structure, promoting a healthier microbial community, and aiding in the overall restoration of soil health.
Techniques in Bioremediation
Techniques in bioremediation are critical to addressing soil contamination effectively. These methodologies employ various strategies that utilize natural processes to eliminate pollutants, essential for restoring balance in ecosystems. Achieving successful bioremediation can significantly enhance soil health, facilitate agricultural productivity, and promote sustainable practices. Understanding the nuances of these techniques helps stakeholders decide on the most appropriate approaches for different contamination scenarios.
In Situ Bioremediation
In situ bioremediation is a technique that processes soil contamination on-site, minimizing disturbance and leveraging the existing biological activity in the ground. By maintaining the natural ecosystem's balance, this method can often be more cost-effective and less invasive than other alternatives. The benefits are manifold:
- Cost Efficiency: Since the process doesn’t require the removal of soil, expenses related to transportation and treatment are minimized.
- Minimal Disruption: Maintaining the natural soil structure can preserve the habitat for beneficial microorganisms.
- Sustainability: Techniques such as bioaugmentation or biostimulation can enhance the indigenous microbial communities without significantly altering the ecosystem.
Adopting methods like bio-nutrient amendments and controlled aeration can stimulate microbial action, promoting decomposition of contaminants. While implementing in situ techniques, it’s crucial to monitor environmental factors like temperature, pH, and moisture to ensure optimal conditions for microbial activity.
Ex Situ Bioremediation
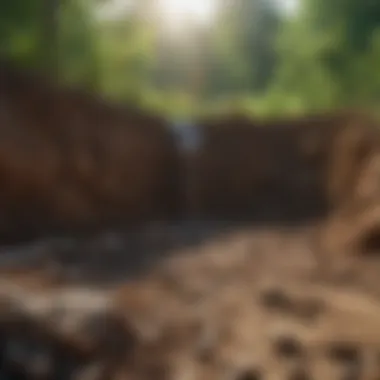
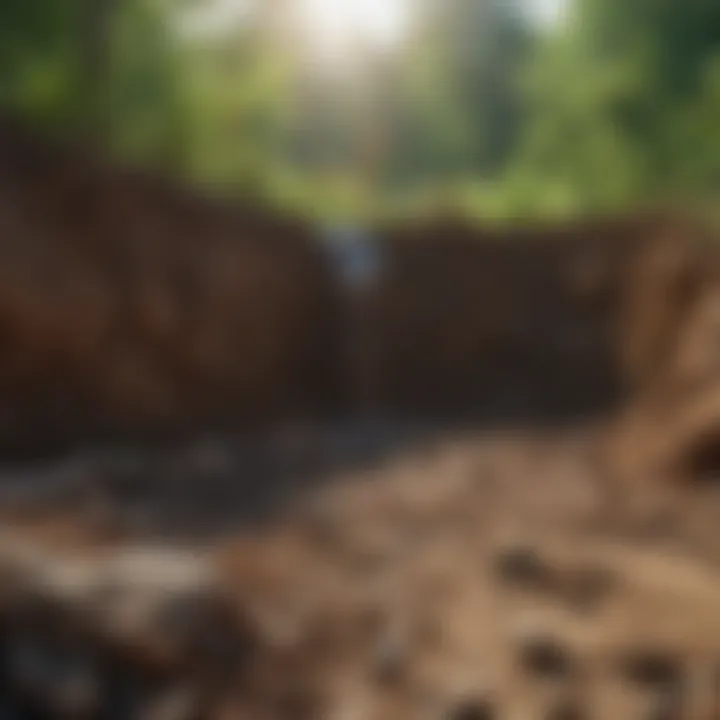
Unlike its counterpart, ex situ bioremediation involves removing contaminated soil for treatment elsewhere. This approach can be particularly effective when dealing with severe contaminant levels or when immediate remediation is necessary. One major advantage is the ability to control the treatment environment more effectively:
- Enhanced Control: Treatment conditions can be optimized for maximum microbial efficacy, potentially accelerating degradation processes.
- Flexibility: Different treatment methods can be applied, from landfarming to biopiles, based on specific contaminants and soil types.
- Comprehensive Analysis: Off-site analysis allows for a deep dive into the contaminant profile, enabling tailored strategies.
Common ex situ techniques include landfarming, where contaminated soils are spread in thin layers and treated with nutrients, and biopiles, which are mounded soils that facilitate aeration and microbial growth. However, it requires careful consideration of logistics and potential secondary contamination during transport and handling.
"Balancing the ecosystem while addressing contamination is the essence of successful bioremediation."
Factors Influencing Bioremediation Efficacy
Understanding the efficacy of bioremediation is like peeling an onion—each layer reveals crucial insights that impact the overall success of restoring contaminated soils. Several factors play a vital role in determining how successful these bioremediation efforts can be. By grasping these elements, practitioners can tailor their strategies more effectively.
Environmental Conditions
The dynamic nature of environmental conditions makes them a pivotal aspect in bioremediation. This includes factors like temperature, pH level, moisture content, and nutrient availability. Each of these conditions can either hinder or enhance the process of pollutant degradation.
- Temperature: Microbial metabolic rates are sensitive to temperature fluctuations. A temperature that's too low can slow down microbial activity, while excessively high temperatures might kill off the microorganisms involved in the degradation process.
- pH Levels: The acidity or alkalinity of the soil can affect the viability of microbial populations. For example, some bacteria thrive in acidic conditions while others prefer alkaline environments.
- Moisture Content: Water is essential. Dry soils might hinder the microbial activities necessary for bioremediation, while overly saturated soils can lead to oxygen deficiency, further stalling the degradation process.
- Nutrient Availability: Just like us, microbes need a balanced diet; lacking essential nutrients can lead them to underperform.
These environmental factors not only influence how efficiently pollutants are broken down but also how quickly bioremediation can take place.
Contaminant Characteristics
Not all contaminants are created equal, and understanding their specific characteristics is essential for effective bioremediation. The chemical structure, toxicity, and concentration of pollutants significantly influence the entire bioremediation process.
- Chemical Structure: Complex organic compounds can be tough nuts to crack, often resisting bioremediation efforts. Conversely, simpler structures may be more readily degraded by microbial action.
- Toxicity: The degree of toxicity can affect which microorganisms are able to thrive in contaminated environments. If a contaminant is highly toxic, it may be lethal to certain microbial populations needed for remediation.
- Concentration: High levels of pollutants can lead to inhibition of microbial activity. Once concentrations exceed certain thresholds, survival and function of microbial communities can be compromised.
The more one knows about the specific contaminants involved, the better one can adapt the biological agents used in bioremediation to tackle them effectively.
Microbial Community Dynamics
The community of microorganisms indigenous to the contaminated site plays a fundamental role in bioremediation efficacy. These microbial populations aren’t just random; they form complex interactions that can either help or hinder bioremediation efforts.
- Diversity: A diverse microbial community may provide a broader range of metabolic pathways to degrade various pollutants. This diverse community can also offer resilience against environmental changes.
- Synergistic Relationships: Some microorganisms can work better together. For instance, certain bacteria produce metabolites that can feed fungi, further enhancing the degradation of pollutants.
- Adaptation: Over time, microbial communities may adapt to the presence of specific contaminants. This adaptation can lead to increased degradation rates as these microbes evolve to better target specific pollutants.
Understanding microbial community dynamics helps practitioners identify operations that might be necessary to enhance bioremediation, such as bioaugmentation—adding specific strains to the mix to promote faster degradation.
"Factors influencing the efficacy of bioremediation are not just technicalities but lifelines that can save our soils from the brink of contamination."
By taking a close look at these factors, stakeholders—from researchers to field practitioners—can better develop strategies that not only address soil contaminants but also safeguard the ecological integrity needed for future sustainability.
Challenges of Bioremediation
Bioremediation stands as a pivotal method for detoxifying contaminated soil, but it is not without its share of hurdles. Understanding these challenges is crucial for stakeholders in environmental science, policy-making, and industry. Comprehending these difficulties can guide more successful implementation and innovate strategies for soil restoration. Specifically, the challenges can be categorized into technical limitations and public perception, both of which play significant roles in shaping the effectiveness of bioremediation projects.
Technical Limitations
Despite its promise, the technical limitations of bioremediation can present considerable roadblocks. The complexity of soil ecosystems means that factors affecting the effectiveness of bioremediation are numerous and often interrelated. For instance, contaminants differ greatly in terms of their chemical structure and toxicity levels. As a result, a one-size-fits-all approach simply doesn't apply; each contaminant may require a unique microbial or phytoremediation strategy.
In particular, the efficacy of bioremediation can be affected by:
- Microbial Adaptation: Not all soil microorganisms are equipped to deal with specific pollutants. Some may take a long time to adapt, or they may not adapt at all, leading to ineffective treatments.
- Environmental Factors: Conditions such as temperature, pH, and moisture levels are critical. For example, severe drought can limit microbial activity, thus hampering the degradation process.
- Scale of Contamination: Larger contaminated areas may present logistical challenges for applying bioremediation techniques effectively.
- Toxicity of Byproducts: Sometimes the breakdown of contaminants can produce toxic byproducts that can further complicate remediation efforts.
The reality is that these technical limitations require a robust understanding of soil microbial ecology and careful planning in project design. Without addressing these concerns, bioremediation projects risk underperformance or total failure.
Public Perception
Public perception is another significant facet that often creates challenges for the implementation of bioremediation. The gap between scientific optimism and public skepticism can influence funding, support, and the adoption of bioremediation projects. Often, laypeople confuse bioremediation with other less effective remediation methods, leading to skepticism about its efficacy.
In many communities, previous negative experiences with pollution cleanup can foster mistrust. To ameliorate these perceptions, effective communication about bioremediation's safety and advantages is crucial. Here are some key points to consider regarding public perception:
- Education and Awareness: Providing clear information on how bioremediation works is vital for building trust. Misunderstandings can often be resolved through community workshops and outreach programs.
- Transparency: Keeping communities updated during the bioremediation process can help counteract concerns about environmental safety.
- Proven Success Stories: Highlighting successful case studies in bioremediation can serve as a positive reinforcement tool. Sharing these local or regional success stories can help the public see the tangible benefits of bioremediation.
- Community Involvement: Engaging the public in the remediation process itself allows them to have a stake in the outcome, helping to turn skeptics into supporters.
"Understanding public sentiment can be as crucial as the scientific process itself; informed communities can lead to more effective and accepted bioremediation strategies."
A successful bioremediation effort hinges on navigating both technical challenges and public perception. To realize the full potential of bioremediation, stakeholders must adopt a proactive approach to address these issues, ensuring both scientific and community buy-in for a cleaner, healthier environment.
Case Studies in Soil Bioremediation
The examination of real-world applications through case studies in soil bioremediation is crucial. These case studies don't just provide theoretical insights; they bring to light practical challenges and triumphs in restoring contaminated land. By analyzing specific instances, researchers and professionals can gather lessons learned, assess the effectiveness of various methods, and ultimately refine bioremediation techniques for future applications.
Through these case studies, one can appreciate the significant benefits of bioremediation, such as its cost-effectiveness compared to traditional remediation methods, and often its ecological advantages, like less disruption to surrounding environments. Also, they present an opportunity to address public misconceptions about the feasibility and safety of using biological processes to clean contaminated sites.
Industrial Sites
Industrial contamination often involves a cocktail of pollutants, from hydrocarbons to heavy metals, which pose severe risks to both the environment and human health. Case studies in these settings illustrate a variety of bioremediation techniques in action. For instance, the Exxon Valdez oil spill in Alaska remains a pivotal reference point. Researchers employed microbes, specially selected for their oil-degrading capabilities, to alleviate the effects of the spill in the soil and surrounding areas.
This application not only showcased microbial efficiency in degrading complex hydrocarbons but also raised awareness about the importance of selecting the right microorganisms for specific contaminants. The methods used included:
- Biostimulation: Adding nutrients to the environment to enhance microbial growth.
- Bioaugmentation: Introducing specifically cultured strains of microorganisms that have a higher ability to degrade pollutants than microorganisms present in the native community.
One learns here that the context of industrial contamination—factor such as soil type and pre-existing microbial community—greatly influences the success of bioremediation efforts.
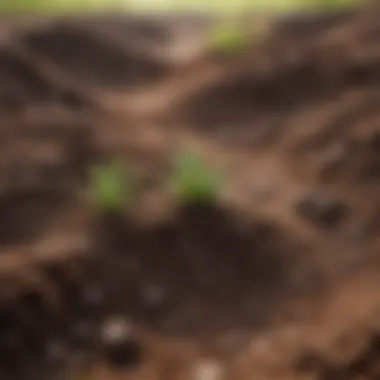
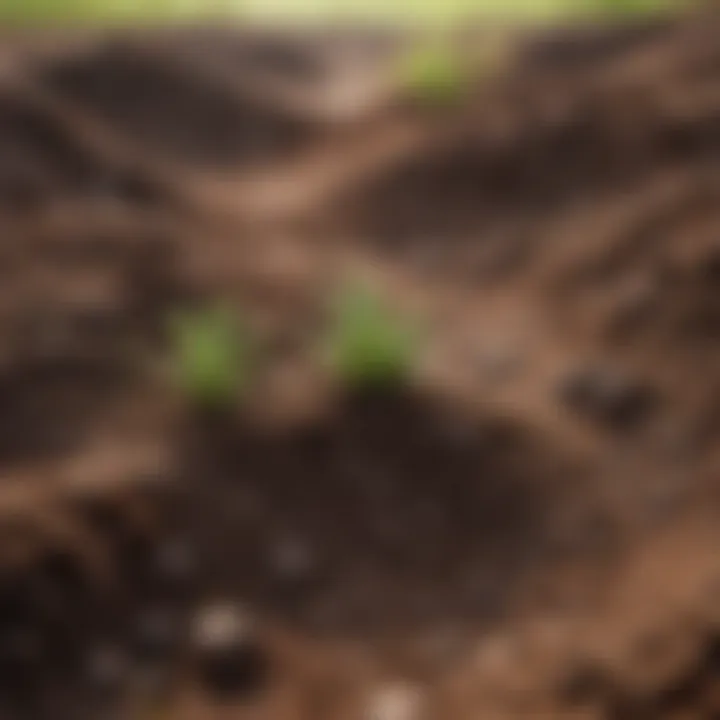
Agricultural Land
Agricultural lands often face contamination from pesticides, fertilizers, and other chemicals that can disrupt soil health and crop production. Case studies provide a stark contrast between chemical and biological rehabilitation strategies. In a notable case in India, phytoremediation was successfully employed on farmland affected by heavy metals, primarily lead and cadmium.
The study utilized specific plant species known for their hyperaccumulating abilities, like Brassica juncea (mustard plant), which can extract metals from the soil and store them in their tissues. This not only recovers soil health but also contributes to the agricultural productivity of the land, which is a win-win.
Moreover, by looking at agricultural applications, we see the integration of bioremediation into sustainable farming practices. Skills and knowledge gathered from these cases substantiate a call for:
- More research on plant species that are effective in diverse soil conditions.
- Implementing crop rotation strategies that can naturally mitigate the effects of soil contaminants.
In summation, case studies in bioremediation are indispensable. They highlight not only specific methods and their success rates but also the interplay between local conditions and the chosen techniques. As stakeholder interest grows, continuing to document these narratives will only fortify the commitment to environmental restoration.
Innovations in Bioremediation Research
In today's environmental landscape, innovative research in bioremediation plays a pivotal role in advancing our ability to tackle soil pollution. The continuous evolution of techniques and methodologies is not just a matter of scientific curiosity; it is an essential response to an ever-growing pollution crisis impacting both ecosystems and human health. Through the eyes of students, researchers, educators, and professionals, these innovations represent hope and utility for rehabilitating contaminated sites.
The significance of innovation in bioremediation research can be unpacked into several dimensions:
- Efficiency: New methods are aimed not only at improving the speed of pollutant degradation but also at enhancing overall effectiveness. This means getting contaminants down to levels that are considered safe for human health and the environment.
- Cost-effectiveness: Innovative approaches often come with reduced operational costs. Techniques that maximize the use of native organisms or optimize substrate use can lessen the financial burden of cleanup efforts.
- Adaptability: Pollutants differ widely in their chemical makeup and toxicity. Innovations strive for versatile solutions that can be modified to suit various contaminants found across diverse geographical regions.
Ultimately, these advancements could redefine how we perceive soil health and remediation processes.
Genetic Engineering of Microbial Strains
As we venture into the realm of genetic engineering, it becomes clear that this cutting-edge technology can revolutionize bioremediation practices. Genetic engineering of microbial strains involves altering the genetic material of microorganisms to enhance their ability to degrade specific pollutants. For instance, researchers have succeeded in modifying strains of Pseudomonas fluorescens to produce enzymes that target and break down heavy metals or organic contaminants more effectively.
Some benefits of genetic engineering in bioremediation include:
- Tailored solutions: Scientists can design microbes specifically to tackle stubborn pollutants that traditional methods struggle with. This is akin to fitting a key into a lock—only by understanding the structure of the contaminant can we choose or design the correct biological agent to dismantle it.
- Increased resilience: Modified strains can be engineered to withstand harsh environmental conditions, making them more viable for real-world application.
Despite its promise, genetic engineering is not without its ethical considerations. Concerns about genetic modification and its implications for ecosystems and public health require ongoing dialogue among scientists, community stakeholders, and regulatory bodies.
Bioreactor Technologies
Bioreactor technology is another exciting avenue in bioremediation research. These systems provide controlled environments for microbial growth, enabling scientists to optimize conditions for the degradation of contaminants. Bioreactors can vary in complexity from simple batch reactors to sophisticated continuous-flow systems, offering a range of scalability suitable for different contamination scenarios.
Key aspects of bioreactor technologies include:
- Controlled Conditions: By modulating temperature, pH, oxygen levels, and nutrient availability, researchers can create ideal conditions for microbial activity tailored to specific pollutants. This control maximizes the effectiveness of bioremediation efforts.
- Scalability: Bioreactors can be adjusted in size from laboratory settings to full-scale operations, making them versatile in addressing both small-scale site assessments and large-scale remediation projects.
Moreover, bioreactors can integrate with other technologies, such as treatment wetlands or phytoremediation, creating holistic systems that tap into multiple remediation mechanisms.
"Innovative methods in bioremediation not only push the boundary of environmental science but also build a bridge towards a sustainable future."
Regulatory Framework and Guidelines
Understanding the regulatory framework surrounding soil bioremediation is essential. These guidelines help establish the path for addressing soil contamination through biological processes. They create a structured approach that not only safeguards the environment but also supports the sustainable use of land and resources. Compliance with regulations ensures that all stakeholders—from researchers to practitioners—are aligned in their objectives and methods. Furthermore, these regulations lend credibility to bioremediation efforts, assisting in the acquisition of funding and public support.
National Guidelines
On a national level, various guidelines dictate the bioremediation practices that can be legally employed. These rules address the types of contaminants that may be treated, permissible methods for remediation, and safety standards mandated to protect both the ecosystem and community health. For instance, many countries have established environmental protection agencies that issue specific recommendations for bioremediation technologies. These recommendations might range from the bioremediation of petroleum hydrocarbon spills to the treatment of solid waste on agricultural land.
- Establishment of Standards: National guidelines often define acceptable limits for soil contaminants that can be targeted through bioremediation.
- Monitoring Requirements: They stipulate the need for ongoing monitoring of the remediation site to measure effectiveness and ensure compliance with pollution standards.
- Public Safety: Guidelines often emphasize protecting public interests, outlining mandatory safety procedures for handling hazardous materials.
In many jurisdictions, these national guidelines also foster collaboration between government agencies, research institutions, and private industry. This collaboration can lead to shared best practices and innovative approaches in bioremediation projects.
International Regulations
On a broader scale, international regulations provide a vital framework for bioremediation efforts, especially when dealing with cross-border pollution. Organizations like the United Nations Environment Programme (UNEP) and the World Health Organization (WHO), lay the groundwork for global standards and approaches.
Such regulations often advocate for:
- Transboundary Cooperation: They emphasize the need for neighboring countries to collaborate on remediation efforts when contamination crosses borders, allowing for a coordinated response.
- Sustainable Development Goals: Many international guidelines align with broader targets, encouraging countries to pursue bioremediation as part of sustainable practices for environmental remediation.
- Global Best Practices: These regulations frequently include guidelines that promote the adoption of advanced technologies and methods, ensuring that countries benefit from international research and innovation.
In summary, both the national and international regulatory frameworks play a significant role in guiding bioremediation strategies. They not only help in maintaining the integrity of the environment but also ensure that the public is adequately protected. Having a clear understanding of these regulations is crucial for anyone involved in soil bioremediation, as it can dictate the feasibility and scope of their efforts. Ultimately, effective frameworks and guidelines translate into successful remediation projects, establishing a benchmark for environmental sustainability.
"Regulatory frameworks can be the bedrock upon which successful bioremediation initiatives are built. They’re not mere suggestions but are mandatory paths to achieving successful outcomes."
Future Directions in Bioremediation
As we peer into the future of bioremediation, it becomes evident that this field is poised for a transformation. The significance of comprehending future directions in bioremediation lies not just in the advancement of technology but in the escalation of environmental challenges posed by ongoing pollution. Human activities continue to lay waste to our soils, making it imperative to discover and refine strategies that effectively rehabilitate these ecosystems. Moreover, it’s crucial to orient this research not only toward addressing contamination but also to adaptively manage the intricate relationships within soil microbiomes.
Emerging Technologies
New technologies sprouting in the field of bioremediation create an exciting horizon for environmental science. A couple of significant areas warrant attention:
- Nanobiotechnology: By employing nanoparticles, scientists can enhance the degradation process of pollutants. These tiny particles can interact with microbes at a cellular level, leading to increased efficiency in pollutant breakdown. For instance, a specialized application of silver nanoparticles showed promise in degrading phenolic compounds in contaminated soils.
- Bioreactor Innovations: Advancements in bioreactor designs allow more control over environmental conditions, optimizing microbial activity. These systems can be fine-tuned to accommodate specific contaminants, thereby improving overall remediation rates. For example, utilizing vertical bioreactors has demonstrated significant improvement in degrading oil spills in marine environments.
The incorporation of synthetic biology is another development that cannot be overlooked. By engineering microbes to possess unique metabolic pathways, targeted degradation of specific pollutants can be achieved, opening new avenues in the fight against soil contamination. These innovations promise enhanced scalability of bioremediation efforts, crucial for large-scale applications.
Socioeconomic Impacts
While technological advancements pave the way for bioremediation's future, socioeconomic considerations play an equally pivotal role in shaping its adoption and success. Understanding these implications helps to ensure that solutions are not only viable scientifically but also practically applicable in communities facing contamination issues.
- Community Engagement: It is vital for local stakeholders to be involved in bioremediation projects. This involvement fosters a sense of ownership and responsibility. When communities comprehend and support bioremediation efforts, it can significantly enhance project success rates.
- Economic Viability: Cost is often a sticking point in deploying bioremediation technologies. Understanding the economic benefits, such as reduced expenditures on pollution cleanup and improved land value, can persuade investors and policymakers to fund these initiatives.
- Policy Development: Implementing supportive policies can catalyze the deployment of innovative bioremediation techniques. As governments recognize the significance of soil health and its link to public health, laws and incentives can directly impact the speed at which new technologies gain traction.
"The integration of emerging technologies in bioremediation, coupled with active community involvement, presents a multifaceted approach to restore soil's health and mitigate future contamination challenges."
By fostering an inclusive, tech-driven landscape, we craft a resilient framework for bioremediation practices that embrace both current environmental demands and future ecological stewardship.