Investigating Nearest Concentration in Science
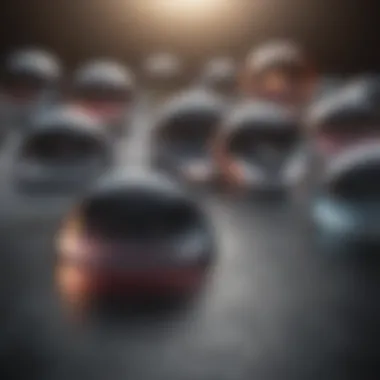
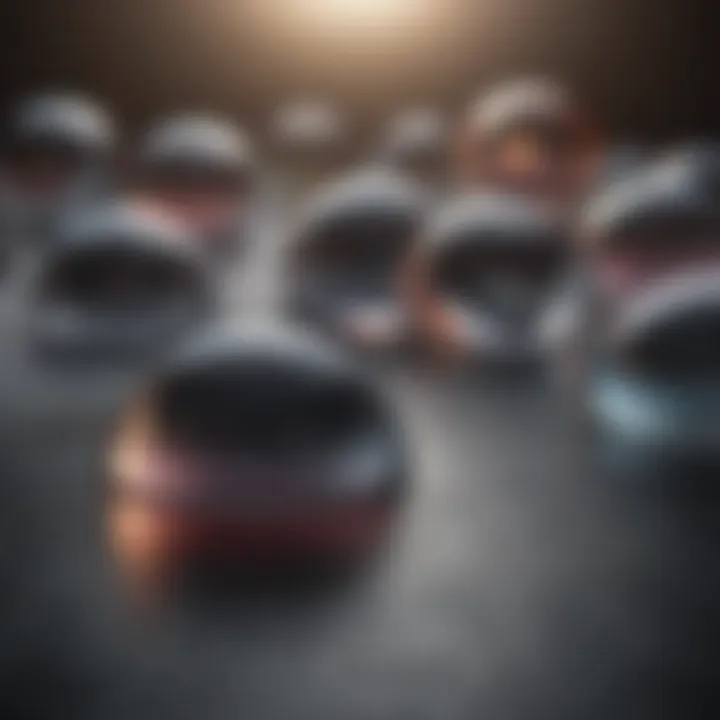
Intro
In scientific research, understanding the concept of nearest concentration plays a pivotal role across various disciplines. The variations in concentrations can significantly influence outcomes in experimental settings and real-world applications. By examining how concentrations behave within biological systems, chemical reactions, and physical phenomena, researchers can gain deeper insights into complex interactions.
This article delves into the factors affecting these concentrations, measurement methodologies, and the implications of their findings. It aims to provide a comprehensive resource for students, educators, researchers, and anyone keen to explore the depths of this significant concept.
Research Overview
Summary of key findings
The investigation into nearest concentration reveals several vital insights. For instance, in biology, concentrations of substrates or enzymes can dictate metabolic rates and, consequently, influence overall organism health. In chemistry, a shift in concentration can lead to changes in reaction rates and equilibrium states, impacting how products are formed. Physical processes, too, are not immune; the concentration of particles in a given volume can affect properties such as pressure and temperature, thereby altering material states.
- Variation in biological systems: Understanding enzyme concentration impacts metabolic pathways.
- Chemical reactions: Concentration shifts determine reaction rates and equilibria.
- Physical processes: Changes in concentration influence thermal and mechanical properties.
Importance of the research in its respective field
Analyzing nearest concentration is essential as it lays the foundation for advancing scientific knowledge across disciplines. In biology, grasping how substrate concentrations affect enzyme activity helps in designing effective pharmaceuticals. In chemistry, students and professionals use concentration principles to devise experiments that yield desired chemical products efficiently. Furthermore, in physics, understanding concentration aids in grasping fundamental principles affecting materials.
Methodology
Description of the experimental or analytical methods used
The methodologies utilized in exploring nearest concentration vary by field but generally involve quantitative approaches. For biological studies, spectrophotometry is a common technique for measuring concentrations of compounds in a solution. For chemistry, titration methods help determine concentration levels in mixtures. Physics often employs mass spectrometry to measure concentration in gaseous forms.
Sampling criteria and data collection techniques
Sampling plays a crucial role in ensuring reliable data. Depending on the discipline, samples must be selected based on criteria like purity, absence of contaminants, and representativeness. Techniques for data collection vary, encompassing both lab-based and field methods.
- Biological Sampling: Often utilizes cultured cell lines or blood samples.
- Chemical Sampling: Involves mixture aliquots subjected to titration or chromatographic analysis.
- Physical Sampling: Relies on gas collection techniques under controlled conditions.
"The understanding of concentration and its variations is central to unlocking mechanisms across diverse scientific fields, ultimately advancing our collective knowledge."
By systematically exploring these elements, we can enhance our grasp of near concentrations as they relate to real-world applications and scientific theory. Our exploration provides insight that can spur innovation and education, promoting an informed approach to science.
Understanding Concentration in Science
Concentration is a fundamental concept in various scientific fields. Its importance spans biology, chemistry, and physics, making it crucial for holistic scientific research. Understanding concentration allows researchers to quantify the amount of a substance in a given volume or area. This quantification affects how substances react and interact, making concentration a key variable in experimental design and data analysis.
By grasping the nuances of concentration, scientists can delve into the mechanisms underlying different phenomena. For instance, in biology, concentrations can influence cellular processes, impacting how cells communicate and function. In chemistry, concentration levels dictate how reactions proceed, determining rates and yields. Thus, a comprehensive understanding of concentration is essential to conducting precise and meaningful research.
Definitions and Importance
Concentration generally refers to the abundance of a particular substance within a specific volume or mass. It is often denoted in various forms, such as molarity, weight/volume, or parts per million. Understanding these definitions is vital for researchers aiming to communicate their findings accurately.
The importance of concentration is evident in its wide-ranging applications:
- Biological Research: Concentration can dictate enzyme efficiency, affecting metabolism and growth in organisms.
- Chemical Processes: In reaction mechanisms, concentration influences the rate at which reactions occur, a cornerstone of chemical kinetics.
- Environmental Science: Measuring the concentration of pollutants is essential for assessing environmental health and planning remediation strategies.
Concentration is not only a fundamental measurement but also a vital tool in scientific inquiry and innovation. Accurate measurements facilitate the reliable interpretation of data, leading to informed conclusions and advancements in various fields.
Units of Concentration Measurement
Different disciplines utilize distinct units for measuring concentration. Understanding these units is essential for effective communication and data analysis. Common units include:
- Molarity (M): Moles of solute per liter of solution. This unit is prevalent in chemistry, giving insight into the reaction conditions.
- Mass Percent: The mass of a solute divided by the total mass of the solution, expressed as a percentage. Used often in biological contexts.
- Parts Per Million (PPM): Indicates the number of parts of solute per million parts of the solution. Common in environmental science for pollutant measurement.
- Grams per liter (g/L): A straightforward measure used in various fields, including chemistry and biology.
It is crucial for scientists to select the appropriate units based on the context of their research. This choice affects the clarity and utility of their findings, ensuring effective communication in scientific discourse.
"Understanding the appropriate units of concentration is key to reproducibility in science. Accurate measurements influence outcomes and interpretations."
In summary, comprehending all aspects of concentration—including its definitions and the various units of measurement—forms the backbone of critical investigation across scientific disciplines. Effective communication of this concept enhances research accuracy and contributes to the advancement of knowledge.
Types of Concentration in Various Disciplines
Understanding different types of concentration across various scientific fields is crucial. Each discipline has its unique approach, yet all share a fundamental goal: to understand how substances interact within a given environment. This section will explore the significant elements of biological, chemical, and physical concentrations, shedding light on their benefits and considerations.
Biological Concentrations
Biological concentrations are fundamental in the study of life sciences. They involve the assessment of how various substances, such as nutrients or toxins, interact within living organisms. Concentration levels can have profound effects on biological processes, such as metabolism, growth, and reproduction.
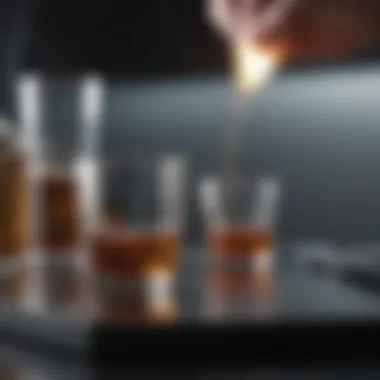
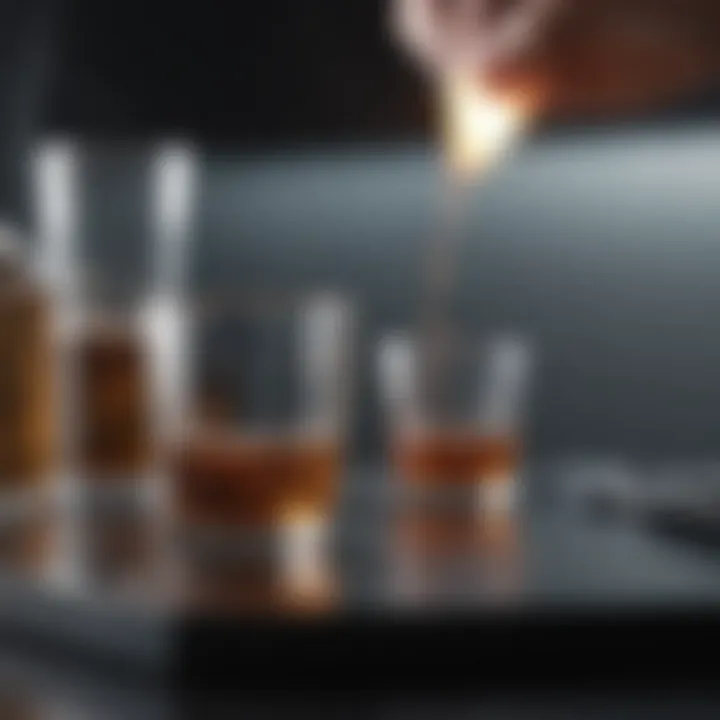
For example, the concentration of glucose in the bloodstream is critical for maintaining energy levels in humans and animals. If glucose levels drop too low, it can result in hypoglycemia, leading to significant health risks. Understanding these concentrations enables researchers to develop targeted therapies for managing diseases like diabetes.
Biological concentrations can influence ecosystems as well. Changes in nutrient concentrations in water bodies can lead to algal blooms, affecting aquatic life and water quality.
As researchers study biological concentrations, they must also consider variability. Seasonal changes, habitat types, and even interactions between species can influence these concentration levels.
Chemical Concentrations
Chemical concentrations deal with the proportions of substances in reactions. They play an essential role in both theoretical and applied chemistry. Understanding chemical concentrations helps chemists determine reaction efficiencies, optimize conditions, and predict outcomes.
For instance, in a reaction where hydrochloric acid combines with sodium hydroxide, the concentration of each reactant will dictate the rate and completion of the reaction. Different concentrations can lead to different product formations, and understanding this concept is crucial for fields like pharmaceuticals, where precise concentrations can mean the difference between success and failure of a remedy.
Additionally, chemical concentrations lead to safety considerations. Handling concentrated substances requires thorough knowledge to ensure safe practices, preventing mishaps that can arise during experimentation.
Physical Concentrations
The concept of physical concentration encompasses the distribution of matter in various states, such as solids, liquids, and gases. This can refer to properties like density and volume. Physical concentrations are particularly important in physics and engineering, where the understanding of how materials behave under various conditions is paramount.
For example, the concentration of gases affects pressure and temperature, which are described by the Ideal Gas Law. This law is fundamental for many applications, including meteorology and engineering.
Understanding physical concentrations also assists in material science, where the properties of materials are affected by their concentration. Researchers can modify these concentrations to achieve desired material characteristics, such as strength or conductivity.
In summary, understanding the types of concentration in various disciplines provides invaluable insights. Each type has its unique implications, influencing practical applications and theoretical understanding in science and engineering.
Factors Influencing Concentration Levels
Understanding concentration levels is critical in many scientific fields. Concentration is never static; it can fluctuate due to various factors. Recognizing these influences not only helps to predict concentration behavior but also informs practical applications in research and industry. This section will delve into key factors that influence concentration levels: environmental variables, biological interactions, and chemical reactions. By exploring these elements, researchers and practitioners can make more informed decisions and advance their work effectively.
Environmental Variables
Environmental conditions play a significant role in determining concentration levels. Factors such as temperature, pH, and presence of competing substances can all impact how concentrations are measured and understood. For instance, a change in temperature may increase or decrease solubility of a substance in a solvent, altering its concentration. Similarly, pH changes can affect the ionization of compounds, thereby influencing their effective concentrations in solution.
- Temperature: An increase in temperature often raises kinetic energy, leading to enhanced molecular interactions which may alter concentrations.
- pH Level: Variations in pH can change the chemical state of compounds, affecting their solubility and ionization.
- Competing Substances: The presence of other chemicals can influence how a compound behaves in a mixture, affecting its concentration.
"Understanding these environmental factors is essential for accurate measurement and interpretation of concentration data."
It is imperative for scientists to consider these variables when conducting experiments or when working with formulations in various disciplines.
Biological Interaction
The interactions among biological entities also significantly influence concentration levels in biological systems. The presence of enzymes, cellular uptake, and metabolic processes can lead to rapid shifts in the concentration of substances. For instance, during a physiological reaction, an enzyme may convert a substrate into a product, thereby decreasing the substrate's concentration and increasing the product's concentration.
Key considerations include:
- Enzyme Activity: The concentration of an enzyme can greatly affect how quickly a substrate is converted, thus impacting overall concentrations in biological reactions.
- Cellular Uptake: Various cells may absorb different amounts of substances, leading to variability in concentrations across tissues or organism levels.
- Metabolism: Metabolic pathways can lead to the production or consumption of substances, creating changes in concentration over time.
Chemical Reactions
Chemical reactions are fundamental processes that can change the concentration of reactants and products significantly. Depending on the reaction conditions, such as pressure, catalysts, and types of reactants, concentration distribution can vastly differ. Understanding reaction kinetics helps in predicting how concentration levels will shift during a reaction.
Considerations include:
- Reaction Kinetics: Faster chemical reactions lead to rapid changes in concentration, while slower reactions result in lower rates of concentration change.
- Catalysts: The presence of a catalyst can lower the activation energy required for a reaction, influencing how quickly concentrations reach equilibrium.
- Equilibrium: In reversible reactions, the concentrations of reactants and products can stabilize at a certain point, which is crucial for understanding dynamic systems.
Methodologies for Measuring Concentration
The methodologies used to measure concentration are vital in scientific research. Accurate measurement of concentration influences experimental outcomes and conclusions drawn from data. Various techniques can provide distinct insights into concentration levels, making the discussion of these methods essential for various scientific fields. Each technique has its own set of advantages, limitations, and optimal usage scenarios. This section evaluates the most significant methodologies, illustrating how they contribute to our understanding of concentration across disciplines.
Spectrophotometry
Spectrophotometry is a widely used technique for measuring concentration based on light absorption properties of substances. The technique relies on the principle that different compounds absorb light at different wavelengths. When light passes through a solution, the amount absorbed correlates directly with the concentration of the substance in the solution. This relationship is often described by Beer-Lambert Law.
The benefits of spectrophotometry include:
- Rapid Analysis: It allows for quick measurement, making it suitable for dynamic experiments.
- Sensitivity: This method can detect low concentrations, making it effective for trace analysis.
- Non-destructive: The sample remains intact, allowing for additional tests.
However, some considerations must be kept in mind:
- Interference: Other compounds may absorb light, leading to inaccuracies.
- Calibration Needs: Regular calibration is essential to maintain accuracy.
Chromatography Techniques
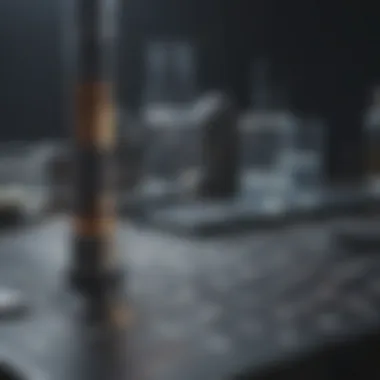
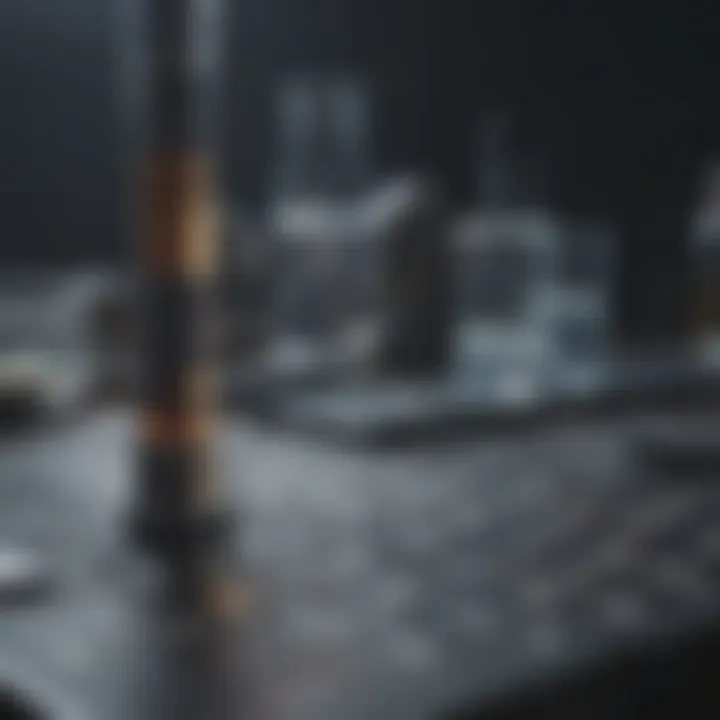
Chromatography encompasses a range of techniques used to separate mixtures into their individual components. This method is essential when dealing with complex samples, where individual substances need to be quantified. Different types of chromatography, such as gas chromatography and liquid chromatography, enable researchers to analyze numerous mixtures efficiently.
The importance of chromatography lies in:
- Separation: It allows for the isolation of individual components for accurate measurement.
- Versatility: Various types can be adapted for different sample types and objectives.
- Quantitative & Qualitative Analysis: Provides information about the concentration and identity of substances.
Considerations for chromatography include:
- Time-Consuming: Some methods require significant time for completion.
- Technical Complexity: Operating chromatography equipment requires training and expertise.
Mass Spectrometry
Mass spectrometry serves as a powerful tool for measuring concentration in a range of research areas. It ionizes chemical species and sorts them based on their mass-to-charge ratio. This technique offers quantification alongside structural analysis, providing a deep understanding of molecular composition.
Key aspects of mass spectrometry include:
- High Sensitivity: Capable of detecting very low concentrations, making it ideal for biomarker studies.
- High Resolution: It can provide precise mass measurements, leading to accurate molecular characterization.
- Versatile Applications: This method is used in diverse fields like proteomics, environmental analysis, and pharmacokinetics.
However, researchers should also consider:
- Cost: Mass spectrometry systems can be expensive to acquire and operate.
- Sample Preparation: Requires careful sample preparation which can introduce errors if not done correctly.
In summary, understanding the methodologies for measuring concentration enhances scientific exploration and innovation. Each technique has specific applications, benefits, and challenges that must be considered in research design.
Applications of Concentration Analysis
The study of concentration has profound implications across various scientific domains. Understanding how concentration affects systems can lead to enhanced methodologies, improved outcomes, and innovative solutions. This section discusses significant applications, focusing on their relevance and the benefits they present in environmental studies, pharmacology, and material science.
In Environmental Studies
In environmental studies, analyzing concentration levels of pollutants and natural substances is critical. Scientists monitor concentrations of heavy metals, nitrates, and carbon compounds to evaluate ecosystems' health. This data aids in pollution control and resource management.
Key elements in this application include:
- Assessment of Ecosystem Health: Measuring concentrations helps identify toxic substances that threaten flora and fauna.
- Regulatory Compliance: Environmental laws often mandate concentration testing. Understanding the levels present ensures compliance, avoiding legal repercussions.
- Pollution Mitigation: By understanding concentration trends, scientists can devise strategies to reduce pollutants in water, air, and soil.
In Pharmacology
In pharmacology, concentration analysis is vital for drug development and efficacy. Pharmacokinetics assesses how a drug behaves in the body over time, relying heavily on concentration levels.
Several key considerations include:
- Optimal Dosage: Through concentration studies, researchers establish effective dosage levels that maximize therapeutic effects while minimizing side effects.
- Drug Interaction: Understanding concentration variations helps scientists predict interactions between multiple drugs, improving patient safety.
- Clinical Trials: Concentration metrics help gauge a drug's effect during trials, guiding essential decisions regarding its viability for public use.
In Material Science
Material science leverages concentration analysis to enhance material properties and develop new materials. This has implications in various fields including electronics and plastics.
Crucial aspects in this field include:
- Material Characterization: Concentration data reveals the composition of materials, guiding the creation of superior materials with desired properties.
- Quality Control: Regular concentration measurements ensure consistency in manufacturing, vital for industries relying on precision.
- Innovation: By manipulating concentrations during synthesis, researchers can develop materials with unique characteristics, opening new avenues for technology.
Accordingly, understanding concentration is not just important; it is integral to advancing scientific knowledge and addressing global challenges.
The applications of concentration analysis demonstrate its versatility and crucial role in shaping scientific research. Moving forward, these insights will continuously inform best practices within various scientific disciplines.
Challenges in Concentration Measurement
Understanding the challenges in concentration measurement is crucial for advancing research and practical applications across disciplines. Accurate concentration measurements are vital for ensuring reliability in experimental results and for making informed decisions in environmental, pharmaceutical, and material science contexts. There are numerous factors that can hinder measurement accuracy, requiring careful consideration from scientists and researchers.
Accuracy and Precision Issues
Accuracy and precision are foundational concepts in measuring concentration. Accuracy refers to how close a measured value is to the true value, while precision conveys the consistency of repeated measurements under unchanged conditions. It is possible for a measurement to be precise but not accurate, leading to significant implications for research outcomes.
Common causes of accuracy issues include:
- Calibration errors in measurement instruments
- Environmental fluctuations such as temperature and humidity
- Human error during sample preparation or data recording
Efforts to ameliorate these issues often involve regular calibration and validation of instruments, alongside the implementation of standardized measurement protocols. Analytical methods such as spectrophotometry, chromatography, and mass spectrometry require stringent adherence to these practices, to mitigate any discrepancies in results.
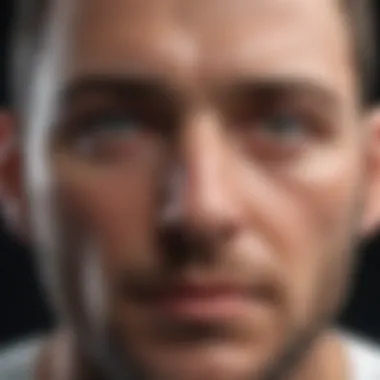
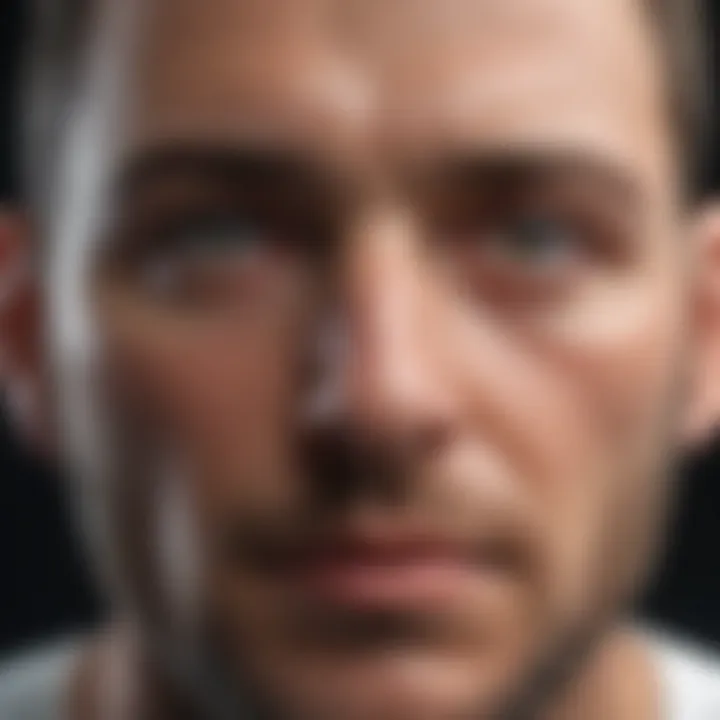
Matrix Effects
Matrix effects occur when the presence of other substances in a sample interferes with the measurement of the target analyte. This can lead to biased results, thus complicating the interpretation of concentration data. Matrix effects are particularly pronounced in complex samples, where various components can interact unpredictably.
Key considerations regarding matrix effects include:
- Identifying constituents in a sample that might affect the measurement
- Developing methods to isolate target analytes from potentially interfering substances
- Utilizing internal standards to help normalize matrix variations
Addressing matrix effects is essential for achieving reliable data. Understanding this challenge helps in optimizing methodologies and enhancing the accuracy of concentration measurements, particularly in fields that depend on precision such as pharmacology and environmental science.
In scientific research, understanding the challenges in concentration measurement is pivotal for ensuring the reliability and validity of findings. Without addressing these challenges, the integrity of scientific conclusions can be undermined.
Advancements in Concentration Research
Advancements in concentration research play a critical role in enhancing our understanding of complex systems across various scientific disciplines. The ability to accurately measure and analyze concentration levels has profound implications. This not only improves experimental outcomes but also drives innovation in subtle yet significant ways.
Innovative Measurement Techniques
Recent developments have introduced a range of innovative measurement techniques that push the boundaries of concentration analysis. For instance, advances in high-resolution mass spectrometry have enabled scientists to detect concentrations at extremely low levels. This is vital in fields like environmental science, where contaminants may exist in traces but have serious impacts.
Ultrasensitive optical sensors have also emerged. These sensors leverage light to identify and quantify molecules with unmatched precision. Such devices are valuable in biomedical applications, where monitoring concentrations of biomarkers is essential for early diagnosis of diseases.
Some notable innovative techniques include:
- Surface Plasmon Resonance: Offers real-time monitoring of interactions at molecular levels, which is highly beneficial in drug discovery.
- Fluorescence Resonance Energy Transfer: Allows for the observation of concentration changes at nanoscale, aiding in cellular studies.
These approaches not only enhance the sensitivity of measurements but also reduce the time required for analysis, further accelerating research and practical applications.
Computational Methods
In parallel with experimental advancements, computational methods have gained prominence in concentration research. These methods facilitate the modeling of concentration dynamics in complex systems. Through simulations and data analysis, researchers can predict how concentrations change under various conditions, which aids in optimizing experimental designs.
For example, computational fluid dynamics is utilized to model the behavior of substances in mixtures. This method allows for the analysis of how factors like temperature and pressure influence concentration profiles in real time.
Machine learning algorithms now provide further depth to this research. By analyzing vast datasets, these algorithms can identify patterns and correlations that might not be visible through conventional statistical methods. This leads to more informed decisions regarding experimental setups and the interpretation of results.
Consequently, researchers can better predict outcomes and devise more effective experiments.
"Integrating computational methods into concentration studies not only sharpens precision but also opens avenues for hypothetical explorations that were not previously conceivable."
Future Directions in Concentration Studies
This section explores pivotal future directions in concentration studies, an important aspect of scientific research. As our understanding of concentration in different contexts evolves, it becomes imperative to explore interdisciplinary approaches and the impact on policy and regulation. These elements not only enhance the quality of research but also align scientific findings with real-world applications. The necessity for improved methodologies and innovative research practices cannot be overstated. Institutions, researchers, and policymakers must work in tandem to address emerging challenges.
Interdisciplinary Approaches
The integration of various scientific disciplines holds the potential to advance the study of concentration significantly. Biology, chemistry, and physics, while traditionally viewed as distinct domains, can benefit from a collaborative approach. This synergy ensures that methodologies tailored for one field can be effectively adapted to another. For instance, analytical techniques developed in chemistry can be applied in biological settings to measure substance concentrations more accurately.
Key advantages of interdisciplinary approaches include:
- Enhanced Problem-Solving: Mixing perspectives from various fields can result in novel solutions to concentration-related challenges. This is particularly vital when addressing complex phenomena such as environmental degradation or public health issues.
- Shared Methodologies: As techniques are refined in one discipline, they can be shared with others, thus improving overall measurement precision. For example, tools from physics can revolutionize how chemists evaluate reaction concentrations.
- Broadened Research Scope: When disciplines intersect, researchers can tackle broader questions concerning concentrations, leading to more comprehensive studies.
Impact on Policy and Regulation
Policy and regulation significantly shape the application of concentration research findings. As concentrations become more finely measured and understood, legal frameworks must evolve accordingly. Policymakers need to consider how scientific insights into concentration can inform regulations across various sectors. This includes environmental protection, public health, and industry standards.
Several aspects demonstrate the critical nature of developing sound policies based on concentration studies:
- Public Health: Accurate concentration data can guide regulations on pollutants and provide evidence for health recommendations. By understanding how concentrations affect human exposure to hazardous substances, better standards can be set to protect communities.
- Environmental Protection: Concentration studies inform regulations concerning emissions and waste management. They help assess the impact of harmful substances on ecosystems and dictate necessary actions for remediation.
- Industry Compliance: Companies must adhere to specific concentration limits as dictated by regulations. This compliance helps mitigate risks associated with chemical usage and helps maintain safety in industrial operations.
By fostering interdisciplinary research and ensuring that policies are informed by the latest concentration studies, science can play a transformative role in addressing some of the most pressing challenges of our time.
Closure
The conclusion section serves as a vital component for summarizing and synthesizing the insights gathered throughout this article. It not only encapsulates the key points discussed but also underscores the significance of ongoing inquiry in the field of concentration studies.
Summary of Key Points
- Understanding Concentration: The article began with a fundamental exploration of concentration, establishing its meaning and measurements across varied scientific disciplines. A clear grasp of this concept is essential for comprehending its applications.
- Types of Concentration: Different fields such as biology, chemistry, and physics have unique considerations for concentration levels. Recognizing these distinctions is critical for researchers and educators.
- Factors Influencing Concentration: A multitude of variables, including environmental conditions and interactions among biological or chemical entities, can affect concentration levels. This awareness aids in better understanding experimental outcomes or natural phenomena.
- Measuring Concentration: The methodologies discussed, like spectrophotometry and mass spectrometry, are tools essential for accurate assessment and analysis, influencing a wide range of scientific findings.
- Applications of Concentration Analysis: The real-world implications of concentration research reflect its relevance in environmental studies, pharmacology, and material science, among others, making it a cornerstone of scientific advancement.
- Challenges and Advancements: The article also highlighted accuracy issues and matrix effects while emphasizing the importance of new measurement techniques and computational methods in overcoming these hurdles.
The Importance of Ongoing Research
Ongoing research in concentration studies remains crucial due to several reasons. First, as science progresses, new challenges and questions arise, necessitating refreshed investigations. Advancements in technology continually reshape the methodologies used, which keeps the field dynamic.
Moreover, as global challenges mount in health and environmental sectors, understanding concentration at a deeper level can guide effective policy-making and practical applications. Continued exploration can lead to innovations and improvements that benefit society widely. By fostering interdisciplinary collaboration, future studies can bridge gaps in knowledge and enhance scientific literacy across various sectors.
In summary, the article aims to inspire continuous dialogue and inquiry within the scientific community regarding the concept of nearest concentration, promoting a deeper understanding and effective application of this essential aspect of science.