Understanding the LNP Manufacturing Process: A Comprehensive Guide
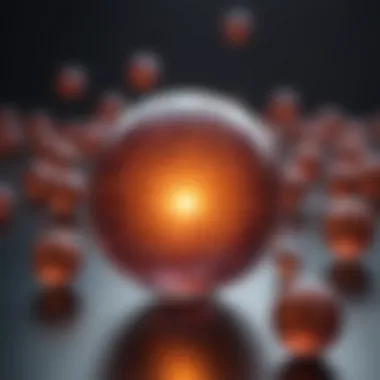
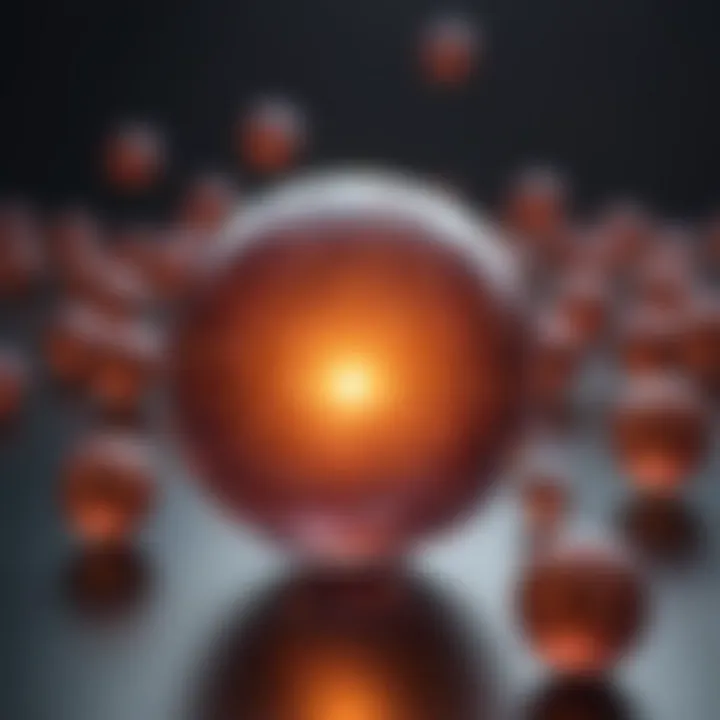
Intro
Lipid nanoparticles (LNPs) have become pivotal in today's pharmaceutical advancements, especially in drug delivery mechanisms. As we delve into the LNP manufacturing process, we will explore the fundamental principles of formulation, the variety of techniques employed in production, and the essential quality control measures. These aspects not only ensure efficacy but also safeguard patient safety. Understanding this process is key for students, researchers, educators, and professionals in pharmaceuticals and biotechnology.
Research Overview
Summary of Key Findings
The research related to LNP manufacturing uncovers various important themes. First, the choice of lipid materials significantly impacts the physical and chemical properties of the nanoparticles. Next, optimizing production methods leads to better scalability and uniformity in drug delivery systems. Furthermore, quality control processes play a crucial role in maintaining the integrity of LNP formulations throughout production.
Importance of the Research in Its Respective Field
The implications of LNP research stretch across various domains. In pharmaceuticals, LNPs enhance the stability and bioavailability of drugs, leading to improved therapeutic outcomes. In biotechnology, they open up new avenues for vaccine delivery and gene therapy. The growing interest in mRNA vaccines during the COVID-19 pandemic has brought LNP technology into the spotlight, highlighting its significance in contemporary medicine.
Methodology
Description of the Experimental or Analytical Methods Used
The manufacturing process of LNPs typically employs methods such as microfluidics, lipid film hydration, and solvent evaporation. Each technique has unique advantages and is selected based on the desired properties of the final product. Microfluidics, for instance, enables precise control over mixing dynamics, which is crucial for achieving desired particle sizes and distribution.
Sampling Criteria and Data Collection Techniques
During the production of LNPs, various sampling criteria are established to optimize formulations. Researchers often analyze particle size, polydispersity index, encapsulation efficiency, and release kinetics. Data collection techniques include dynamic light scattering for size measurements and high-performance liquid chromatography for assessing drug loading.
Effective quality control is integral to successful LNP manufacturing, influencing both the safety and efficacy of drug delivery systems.
Closure
In summary, understanding the LNP manufacturing process is essential for leveraging its full potential in drug delivery. The insights from this research guide future innovations in pharmaceuticals and biotechnology.
Prelims to LNPs
Lipid nanoparticles (LNPs) are becoming increasingly significant in the pharmaceutical landscape. Their importance lies in their role as carriers for various therapeutic agents, particularly in the context of modern drug delivery systems. LNPs facilitate the safe and effective transport of nucleic acids and drugs within the body, ensuring the stability and bioavailability of these substances. Understanding the fundamentals of LNPs is essential for those involved in biopharmaceutical research and development.
Definition of Lipid Nanoparticles
Lipid nanoparticles are nanoscale structures composed primarily of lipids. They can encapsulate therapeutic molecules, such as RNA, DNA, or proteins, and protect them from degradation. This encapsulation allows for targeted delivery, enhancing therapeutic efficacy while minimizing side effects. LNPs can vary in composition, size, and method of production, which impacts their performance and application in drug delivery.
LNPs typically consist of four main components:
- Lipids: These form the core structure and consist of cationic, anionic, or neutral lipids, each serving different roles in encapsulation and delivery.
- Nucleic Acids: These are the active therapeutic agents targeted for delivery.
- Solvents: Commonly used solvents must be biocompatible and non-toxic to ensure patient safety.
- Stabilizers: These help maintain nanoparticle structure and stabilize the formulation during storage and transport.
Historical Context
The concept of using lipid-based systems for drug delivery originated in the 1970s. Early research focused on liposomes, which are spherical vesicles that can carry both hydrophilic and hydrophobic drugs. However, liposomes faced limitations regarding stability and cellular uptake. This led to investigations into lipid nanoparticles.
In the 1990s, scientists began to refine the LNP manufacturing process to improve the delivery of genetic materials. The breakthrough came with the development of mRNA vaccines, especially during the COVID-19 pandemic. The Pfizer-BioNTech and Moderna vaccines, both based on LNP technology, demonstrated the capability of lipid nanoparticles to efficiently deliver mRNA into cells, thus revolutionizing vaccine development.
"Lipid nanoparticles have emerged as the cornerstone of modern biopharmaceutical delivery systems, particularly post-2020 with the surge in mRNA vaccine technologies."
Today, LNPs are at the forefront of biopharmaceutical research. They are used not only in vaccines but also in gene therapy and treatment for various diseases such as cancer. As the field continues to evolve, ongoing research into LNP formulation techniques, stability, and targeting mechanisms remains vital.
Importance of LNPs in Drug Delivery
Lipid nanoparticles (LNPs) have emerged as a pivotal mechanism in modern drug delivery systems. Their significance lies in their versatile capabilities to encapsulate various therapeutic agents, especially nucleotides like mRNA and DNA. This section elucidates the fundamental role LNPs play across multiple facets of drug delivery, exploring their advantages and some considerations surrounding their use.
One of the primary advantages of LNPs is their ability to protect sensitive biomolecules from degradation. Common therapeutic agents, such as mRNA, are quite fragile. They require a stable delivery system to reach their target cells intact. LNPs serve this purpose excellently, ensuring that the therapeutic payload is shielded from environmental factors until it can be delivered to the appropriate site of action. This targeted delivery is crucial in maximizing therapeutic efficacy and minimizing off-target effects, a common challenge in drug administration.
Additionally, LNPs enhance cellular uptake through endocytosis. The lipid composition of LNPs enables them to fuse with the cellular membrane, facilitating the entry of their payload into the cells. The efficiency of this process has made LNPs extremely important in vaccine development, particularly for the administration of mRNA vaccines.
"LNPs represent a breakthrough in drug delivery, enabling novel therapies that were previously challenging to realize."
Role in mRNA Vaccines
The role of LNPs in mRNA vaccines cannot be overstated. Recent developments during the COVID-19 pandemic have showcased this technology's potential and efficacy. Companies like Pfizer-BioNTech and Moderna have utilized LNPs to deliver their mRNA vaccines effectively. In these formulations, LNPs encapsulate the mRNA, ensuring stability and protection against degradation while promoting efficient cellular uptake upon administration.
These LNPs not only protect the mRNA but also help in determining the immunogenicity of the vaccine. Tailoring the lipid composition can significantly influence the vaccine's performance, adjusting critical properties like size, charge, and surface characteristics. This optimization is vital for achieving the desired immune response while ensuring safety.
In summary, the effectiveness of mRNA vaccines heavily relies on the adaptability and efficiency of LNPs as carriers. Without this innovative technology, the rapid development of effective vaccines like those for COVID-19 would not have been possible.
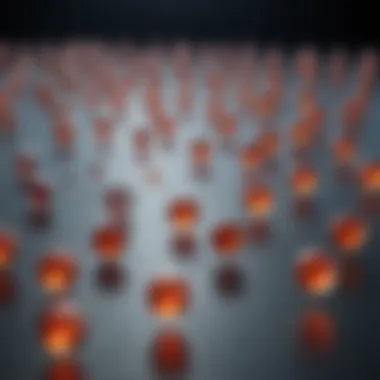
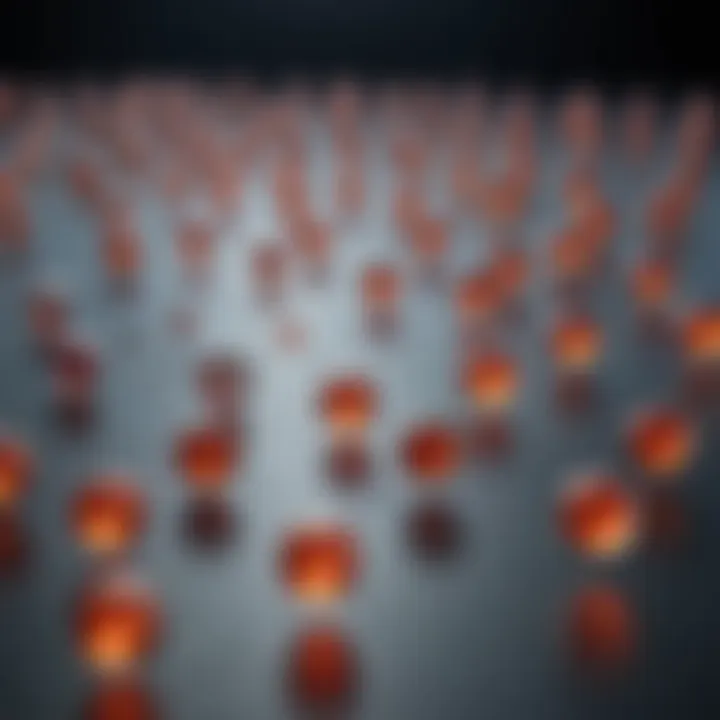
Applications in Gene Therapy
Beyond vaccines, LNPs play an essential role in gene therapy, another groundbreaking area in medicine. Gene therapies often require the delivery of large or negatively charged nucleic acids, which present challenges in traditional delivery methods. LNPs facilitate the delivery of these entities by encapsulating them within a lipid matrix, which can traverse cellular membranes more effectively.
The versatility of LNPs allows for the targeting of various tissues and organs, which is crucial for conditions like genetic disorders and cancers. Moreover, recent research has shown that LNPs can be designed for specific tissues, enhancing their ability to deliver therapeutic genes precisely where they are needed.
In summary, LNPs represent a significant advancement in drug delivery. Their ability to provide a protective, efficient means of delivery for sensitive therapeutic agents, particularly in mRNA vaccines and gene therapies, highlights their crucial role in the ever-evolving landscape of medicine.
Lipid Selection for LNPs
The selection of lipids in lipid nanoparticle (LNP) formulation plays a pivotal role in the effectiveness of drug delivery systems. The properties of lipids influence how well the drug interacts with cells, how stable the delivery system is, and ultimately how efficacious the therapy can be. Understanding lipid selection is critical for researchers and professionals who are involved in the production of LNPs, especially given the diverse range of available lipid types and their unique functionalities.
In LNP formulation, lipids serve multiple purposes:
- They encapsulate the active pharmaceutical ingredient (API).
- They assist in cellular uptake following administration.
- They contribute to the stability of the LNP during storage and delivery.
Selecting the right lipid involves evaluating its physicochemical properties, biocompatibility, and its ability to stabilize the API. Factors like the fatty acid chain length, degree of saturation, and functional groups can determine the overall performance of the lipid in LNPs. Therefore, a deep understanding of various types of lipids and their interactions with the encapsulated payload is essential.
Types of Lipids Used
LNP formulations typically utilize a few types of lipids: phospholipids, cholesterol derivatives, and cationic lipids are among the most common. Each type serves distinct purposes during the formulation process.
- Phospholipids: They are the primary building blocks of cell membranes. Phospholipids like 1,2-Dioleoyl-sn-glycero-3-phosphocholine (DOPC) are often used due to their ability to form stable lipid bilayers. They enhance biocompatibility and help in the effective encapsulation of nucleotides.
- Cholesterol: Often included in formulations to modulate membrane fluidity. Cholesterol improves the stability of the liposomes under physiological conditions. This makes it more likely that the LNP will maintain its integrity until it reaches the target cells.
- Cationic lipids: These lipids can interact with negatively charged nucleic acids, allowing for better encapsulation. Cationic lipids, such as N-(1-(2,3-dioleoyloxy)propyl)-N,N,N-trimethylammonium chloride (DOTMA), promote cellular uptake through electrostatic interactions.
Different applications may require different lipid compositions. Therefore, the choice of lipids should align closely with the intended therapeutic application of the LNP.
Impact on Stability and Efficacy
The selected lipids directly impact both the stability and efficacy of the LNPs. Stability is crucial for maintaining the integrity of the nanoparticle throughout its storage and transport. If the lipids are not selected carefully, the LNPs may aggregate or degrade, resulting in loss of function.
The efficacy of LNPs is tied to their ability to deliver the encapsulated API effectively. Lipid properties, such as chain length and saturation, can influence how well the LNPs can interact with cell membranes for successful delivery. Longer fatty acid chains may enhance membrane fusion, which is vital for cellular uptake.
In summary, lipid selection is not simply a matter of preference, but rather it is a fundamental aspect of LNP manufacturing that affects both the performance and the applicability of drug delivery systems. Careful consideration of lipid types and their interactions can lead to significant advancements in the field of pharmaceuticals and biotechnology.
"The choice of lipid can determine the success or failure of a lipid nanoparticle formulation."
By selecting appropriate lipids, researchers can enhance stability, biocompatibility, and biodistribution, all of which are critical for effective therapeutic outcomes.
Formulation Techniques in LNP Manufacturing
The formulation techniques employed in lipid nanoparticle (LNP) manufacturing are crucial for achieving efficient drug delivery. These methods directly influence the characteristics of the LNPs, such as size, stability, and encapsulation efficiency. Understanding these techniques is essential for optimizing LNP design, enhancing therapeutic efficacy, and ensuring a swift transition from laboratory research to clinical applications.
Microfluidic Mixing
Microfluidic mixing is a cutting-edge technique that allows for precise control over the formulation parameters during LNP assembly. This method involves the manipulation of liquids at the microscale, which enables efficient mixing of lipids and nucleotides. One of the prominent advantages of microfluidic mixing is its ability to create uniform LNPs with controlled size and polydispersity.
- Efficiency: Microfluidic systems can rapidly produce high-quality LNPs with minimal waste, compared to traditional methods. The continuous flow design enhances mass transfer, leading to better encapsulation of therapeutic agents.
- Reproducibility: The controlled environment ensures consistent results, a vital aspect for scalability in LNP manufacturing. This consistency leads to more reliable therapeutic outcomes in clinical settings.
- Customization: By adjusting flow rates and channel dimensions, researchers can tailor the properties of LNPs to meet specific needs, thus broadening their potential applications.
While microfluidic mixing presents numerous benefits, there are also challenges. Manufacturing equipment can be costly and may require specialized knowledge.
Reverse Phase Evaporation
Reverse phase evaporation is another important technique used in the formulation of LNPs. This method involves creating a water-in-oil emulsion where the lipids and aqueous phase containing nucleotides are mixed. Following this, the solvent is evaporated, resulting in the formation of lipid nanoparticles.
- Encapsulation: This technique allows for high encapsulation efficiency of hydrophilic compounds, making it suitable for delivering mRNA or siRNA molecules.
- Control: By varying the solvent evaporation rate, researchers can influence the size and stability of LNPs. This adaptability aids in optimizing formulations for specific therapeutic applications.
- Versatility: Reverse phase evaporation can accommodate different lipid compositions, which is critical in exploring new formulations for various medical treatments.
However, this technique can also introduce complexities, particularly in the handling of organic solvents, which necessitate cautious management to ensure safety and compliance with regulatory standards.
These formulation techniques are pivotal in advancing LNP technology. Understanding their intricacies and implications for drug delivery systems fosters innovation in pharmaceutical research.
Characterization of LNPs
The characterization of lipid nanoparticles (LNPs) is crucial in the realm of drug delivery systems. It involves a detailed examination of their physical and chemical properties, allowing researchers to assess their functionality and effectiveness. Precise characterization ensures that these nanoparticles meet the specific criteria needed for safe and efficacious delivery of therapeutic agents.
Size and Morphology Analysis
Size and morphology of LNPs play a significant role in determining their biological interactions and delivery efficiency. Typically, the size of LNPs ranges from 50 to 200 nanometers, which is optimal for cellular uptake. Various techniques are used for size analysis, including dynamic light scattering (DLS) and transmission electron microscopy (TEM). These methods provide insights into the average size, size distribution, and shape of the nanoparticles.
A well-defined size distribution improves uptake by cells. Moreover, the morphology influences how LNPs interact with biological membranes. For instance, spherical shapes often favor endocytosis, thereby enhancing delivery efficiency. Smaller LNPs may show improved circulation time, while larger ones may be more likely to aggregate or be cleared by the immune system. Therefore, ongoing analysis is essential for determining the best formulations for specific applications.
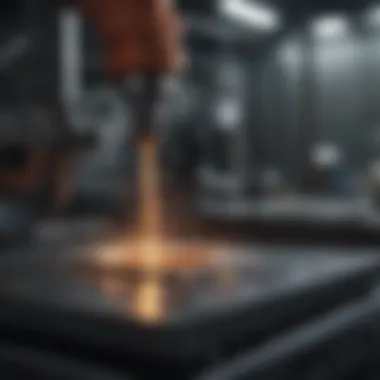
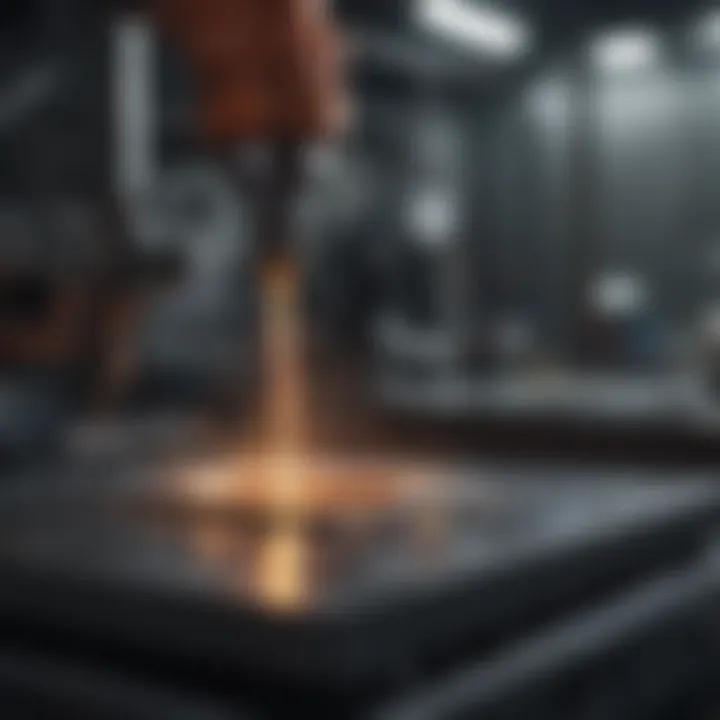
Encapsulation Efficiency
Encapsulation efficiency refers to the percentage of the active pharmaceutical ingredient (API) that is successfully enclosed within the lipid nanoparticle structure. This metric is critical to evaluating the performance of LNP formulations. High encapsulation efficiency is desired not only to maximize the therapeutic payload but also to minimize wastage of valuable drug materials.
Several factors influence encapsulation efficiency, including lipid composition, method of preparation, and the physicochemical properties of the API. Typically, more hydrophobic drugs show higher encapsulation efficiency within LNPs.
"The evaluation of encapsulation efficiency provides insights into the ability of LNPs to protect and deliver the therapeutic materials to the target site."
Techniques to assess encapsulation include centrifugation followed by quantification of free drug through various analytical methods, such as HPLC or mass spectrometry. Improving encapsulation efficiency can lead to enhanced therapeutic outcomes and reduced side effects, making it a central consideration during LNP formulation development.
Quality Control in LNP Manufacturing
Quality control is a critical aspect of lipid nanoparticle (LNP) manufacturing. It ensures that the final product meets safety, efficacy, and performance standards. In the field of pharmaceuticals, where LNPs are increasingly utilized, maintaining quality is not just beneficial, it is a necessity. The significance of robust quality control cannot be overstated. It provides assurance to regulators, healthcare providers, and ultimately, to patients who rely on these advanced drug delivery systems.
Regulatory Standards
Adherence to regulatory standards establishes a framework within which LNP manufacturing must operate. Organizations like the Food and Drug Administration (FDA) and the European Medicines Agency (EMA) outline specific guidelines that manufacturers must follow. These regulations cover various aspects of LNP production, including formulation, testing, and final product evaluation.
Key regulatory standards relevant to LNP manufacturing include:
- Good Manufacturing Practice (GMP): Ensures that products are consistently produced and controlled according to quality standards.
- Quality by Design (QbD): Encourages a deep understanding of the production process to build quality into the final product from the outset.
- Preclinical Guidelines: These guide the evaluation of LNPs before clinical trials, assessing their safety and biological properties.
Following these standards minimizes risks related to contamination, variability, and suboptimal performance in LNPs.
Testing Methods
A variety of testing methods are employed to ascertain the quality of LNPs during and after manufacturing. Rigorous testing helps detect any deviations in formulation and performance metrics. Some commonly used methods include:
- Dynamic Light Scattering (DLS): Used for assessing the size distribution and stability of LNPs in solution.
- High-Performance Liquid Chromatography (HPLC): Commonly applied to measure encapsulation efficiency and quantify lipids and nucleotides.
- Transmission Electron Microscopy (TEM): Offers insights into the morphology of LNPs, providing information on their shape and structural integrity.
Implementing diverse testing methods not only verifies compliance with regulatory standards but also serves as an assurance to stakeholders regarding the product's reliability.
In summary, quality control in LNP manufacturing cannot be regarded as an afterthought. It permeates every stage of production from the selection of raw materials through to final testing. A rigorous quality control framework facilitates the safe introduction of innovative drugs to the market, ultimately leading to enhanced therapeutic outcomes and improved patient care.
"Quality is never an accident; it is always the result of intelligent effort." - John Ruskin
Maintaining high-quality standards is especially crucial in a rapidly evolving field such as biopharmaceuticals. Manufacturers must remain agile in adapting to new regulations while fostering a culture of quality that prioritizes patient safety.
Challenges in LNP Production
The production of lipid nanoparticles (LNPs) presents significant challenges that must be addressed to ensure optimal performance and applicability in various therapeutic areas. Understanding these challenges is crucial for researchers and manufacturers aiming to refine their processes. The issues encountered in LNP production can impact efficiency, cost effectiveness, and ultimately, the success of drug delivery systems. This section focuses on two main challenges: scalability issues and cost considerations.
Scalability Issues
Scalability is a prominent challenge for LNP manufacturing. In early-stage research and development, LNPs are often produced in small batches for experimental purposes. However, transitioning from small-scale to large-scale production is not straightforward.
Key factors contributing to scalability issues include:
- Reproducibility: Maintaining consistent quality and performance in LNPs across varying batch sizes can become problematic. Variations in equipment, environment, and raw materials can introduce significant discrepancies.
- Process Optimization: Existing methods may not efficiently scale. Specific techniques may function well on a lab scale but fail to produce LNPs effectively when adjusted for larger volumes.
- Material Availability: Sourcing materials in larger quantities while ensuring quality standards may pose logistical challenges. Not all suppliers can meet the rigorous demands of large-scale applications.
The inability to efficiently scale up production can hinder the timely delivery of critical therapies, potentially affecting patient care.
Cost Considerations
Cost is another significant concern in LNP production. The synthesis and manufacturing of lipid nanoparticles can be expensive, driven by a range of factors:
- Raw Material Costs: High-quality lipids and other components necessary for LNP formation can contribute considerably to overall production costs. Determining cost-effective alternatives without compromising performance is vital.
- Technology and Equipment Investment: Advanced technologies and specialized equipment for LNP manufacturing require significant upfront investment. Startups and smaller companies may find this prohibitive.
- Regulatory Compliance: Navigating the regulatory landscape incurs additional costs. Meeting the standards set by agencies such as the FDA or EMA often requires extensive documentation, testing, and validation.
Balancing these costs with the need for high-quality LNPs is essential. Companies unable to manage expenses may struggle to sustain their operations, hindering innovation in this field.
"The efficiency of LNP production determines whether promising therapies will reach the market and patients."
Recent Advances in LNP Technology
Recent advances in lipid nanoparticle (LNP) technology have played a crucial role in the evolution of drug delivery systems. These innovations enhance the delivery mechanism of therapeutic agents, particularly for complex molecules like mRNA. The importance of this topic cannot be overstated, as the developments contribute not only to improved efficacy and safety but also to the broader acceptance and application of LNPs in clinical settings.
One of the key advancements in this field is the formulation strategies that allow for better stability and biodistribution of LNPs. As researchers discover new lipid formulations, they can tailor the physical and chemical properties of nanoparticles. This tailoring directly influences the release profiles, cellular uptake, and ultimately, the therapeutic outcomes. The integration of novel excipients and lipid-like materials has opened new avenues for improving the performance of existing treatments.
"Recent advances in LNP technology are transforming the field of biopharmaceuticals, promoting more effective and safer therapeutic strategies."
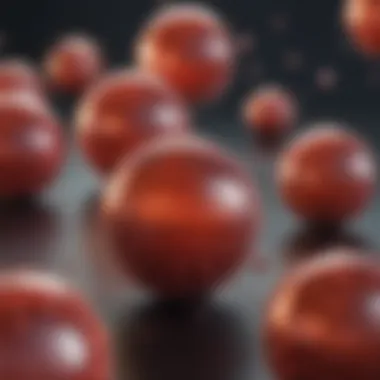
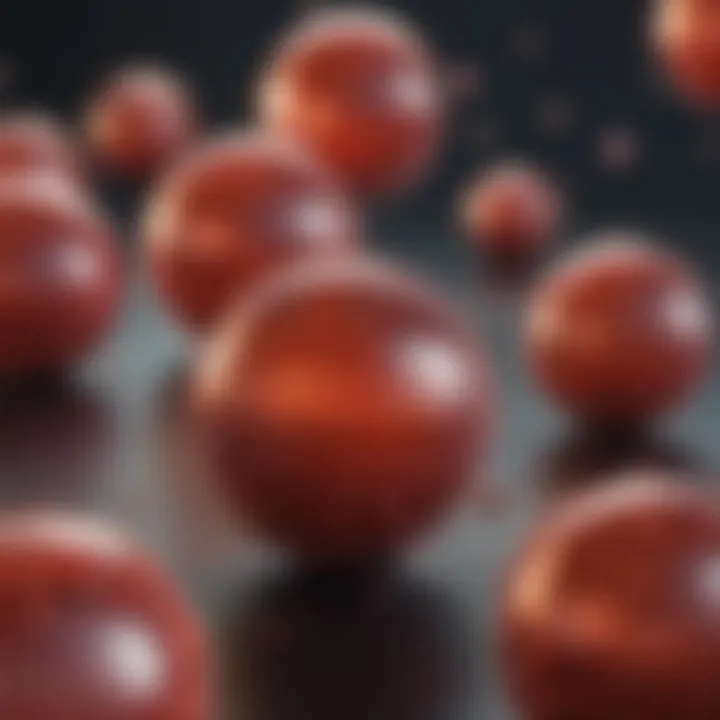
Innovative Formulation Strategies
Innovative formulation strategies focus on refining the composition of lipid nanoparticles to better suit specific therapeutic needs. For instance, the design of LNPs that can evade the immune system can significantly improve their clinical applications. Using stealth lipids or pegylated lipids enhances circulation time in the bloodstream and reduces recognition by the immune system.
In addition, using different ratios of lipids can optimize LNP formulation for desired functions. It can lead to improvements in encapsulation efficiency and stability in biological environments. Various mixing techniques, such as microfluidic mixing, have also been explored to achieve a more uniform particle size, contributing to controlled drug release and enhanced bioavailability.
New Drug Candidates Utilizing LNPs
With the maturity of LNP technology, new drug candidates are increasingly utilizing these systems. For instance, many companies are now developing LNPs to deliver nucleic acid therapeutics beyond mRNA vaccines. RNA interference (RNAi) therapies and gene editing applications are frontiers where LNPs display significant potential.
Without LNP technology, many of these drug candidates could not achieve the required efficacy. The capability of LNPs to encapsulate large and complex molecules opens the door for treating rare diseases and cancers that previously lacked effective options. Thus, the incorporation of LNPs in clinical trials for various drug candidates demonstrates the ongoing promise and innovation within this technology.
Case Studies in LNP Applications
Lipid nanoparticles (LNPs) have become a focal point in modern biopharmaceutical applications due to their versatility and effectiveness. Understanding practical cases where LNPs have been applied can provide invaluable insights into their impact on drug delivery systems. These case studies illuminate not only the benefits of LNP technology but also the specific challenges that researchers and developers face. By analyzing real-world applications, we can appreciate the progress made and identify future directions for research and implementation.
LNPs in COVID-19 Vaccination
One of the most significant applications of LNPs has been in the development of COVID-19 vaccines. Notably, the Pfizer-BioNTech and Moderna vaccines utilize LNPs to encapsulate and deliver mRNA. This method enhances the stability of the mRNA and facilitates its delivery into human cells. The lipid nanoparticles protect the mRNA from degradation while ensuring effective cellular uptake, a critical factor in the vaccines' success.
The importance of LNPs in these vaccines can be understood through several key points:
- Encapsulation Efficiency: The ability of LNPs to encapsulate mRNA is crucial. Effective encapsulation leads to higher vaccine potency and a robust immune response.
- Safety Profiles: By carefully selecting lipid components, researchers can minimize the potential for adverse reactions, ensuring that LNPs are safe for human use.
- Scalability: The production techniques for LNPs used in vaccines have proven scalable, allowing for rapid and widespread distribution amid a global health crisis.
Through these studies, it is clear that LNPs play a crucial role in mRNA vaccine technology. Their ability to enhance stability and deliver genetic materials is a fundamental advancement in modern immunology.
LNP-Mediated Cancer Therapies
Another promising application of lipid nanoparticles is in cancer therapy. LNPs can effectively deliver chemotherapeutic agents while minimizing systemic side effects. By using targeted delivery systems, LNPs can concentrate drug action at the tumor site, reducing the impact on healthy tissues.
Key aspects to consider in LNP-mediated cancer therapies include:
- Targeted Delivery: LNPs can be engineered to recognize specific tumor markers, allowing for precision medicine approaches in oncology.
- Reduced Toxicity: The controlled release of chemotherapeutics through LNPs leads to lower concentrations of drugs in the bloodstream, thereby decreasing side effects commonly associated with traditional chemotherapy.
- Combination Therapies: By co-delivering multiple therapeutic agents, LNPs enhance the efficacy of treatment protocols, allowing for synergistic effects that improve patient outcomes.
Overall, LNP-mediated cancer therapies showcase the potential of lipid nanoparticles in revolutionizing how we approach treatment. As research progresses, these case studies reveal vital lessons that can shape future innovations in drug delivery systems.
Future Directions in LNP Research
The future of lipid nanoparticles (LNPs) research holds significant prospects for advancements in drug delivery systems. As scientists refine these technologies, the integration of LNPs into diverse therapeutic areas will likely increase. Understanding these developments is essential for unlocking their full potential in the biopharmaceutical sector.
Potential for Personalized Medicine
Personalized medicine derives its strength from the ability to tailor treatments to individual patients. LNPs offer unique opportunities in this space. Their formulation can be adjusted to accommodate distinct biological characteristics, allowing more effective drug delivery. By targeting specific cells or tissues, LNPs can enhance therapeutic outcomes while minimizing adverse effects.
For example, cancers present heterogeneity, where tumors of the same type may respond differently to treatments. With personalized LNP formulations, oncologists can deliver chemotherapeutic agents specifically to tumor cells, depending on each patientโs unique tumor profile. This targeted delivery not only improves efficacy but also reduces systemic toxicity, a significant hurdle in traditional therapies.
In essence, as LNP technology advances, it paves the way for developing specialized medications that are better matched to individual patients. This not only enhances treatment effectiveness but could also lead to better patient adherence and outcomes over time.
Collaborative Research Opportunities
Collaborative research initiatives are critical to advancing LNP technologies and their applications. Different institutions can bring varied expertise, fostering innovation in lipid nanoparticle systems. Researchers can explore new lipid formulations, mixing methods, and targeting mechanisms by pooling resources and knowledge.
Partnerships between academic institutions, pharmaceutical companies, and biotech firms can catalyze breakthroughs. For instance, an academic lab might develop a novel lipid composition, while a biotech partner can provide the necessary tools for clinical testing.
Moreover, multidisciplinary collaborations enhance the research framework. They can involve chemists, biologists, and medical professionals, ensuring comprehensive approaches to problem-solving. Such collaborations can also open doors for funding opportunities and access to specialized equipment.
"The future of lipid nanoparticles is intricately linked to collaborative efforts that foster innovation, experimentation, and the practical application of research discoveries."
Closure
The conclusion serves as a crucial summation of the insights presented throughout this article. It reflects the overarching themes regarding lipid nanoparticle (LNP) manufacturing processes, particularly emphasizing the intricate balance between formulation, production, and quality control. The importance of LNPs in modern biopharmaceutical contexts cannot be overstated, as they represent a cutting-edge approach to drug delivery innovation.
Summary of Key Points
In summary, the article elucidates significant aspects of LNP manufacturing:
- Detailed understanding of lipid selection and its impact on drug efficacy.
- Explanation of formulation techniques, such as microfluidic mixing and reverse phase evaporation, that enhance LNP quality.
- Characterization methods used for assessing size, morphology, and encapsulation efficiency.
- Quality control measures that follow regulatory standards, ensuring safety and compliance.
- Challenges faced in scaling LNP production and considerations related to cost efficiency.
- Recent technological advances and case studies, highlighting LNP applications in crucial areas like vaccine development.
- Future directions inclined towards personalized medicine, advocating for collaborative research in the field.
Summarizing these key points allows the reader to appreciate the multifaceted nature of LNP technology and its revolutionary role in drug delivery systems.
Implications for the Future of Biopharmaceuticals
The implications stemming from the insights provided in this article are significant. LNPs stand at the forefront of biopharmaceutical advancement, especially in fields such as gene therapy and vaccine development. Emphasis on personalized medicine increases the potential for tailored therapies that align closely with patient-specific needs, further propelling research in this direction.
Collaboration among academic institutions, industry stakeholders, and regulatory bodies is equally vital. By fostering an environment of shared knowledge, the progress of LNP technologies can expedite innovations that follow stringent safety protocols and afford broader access to life-saving therapies. The journey of LNP manufacturing is not just about technology but also about creating value for patients and healthcare systems worldwide.
"The future of LNP technology holds immense benefits for biopharmaceuticals, facilitating advancements in drug delivery, safety, and efficacy."