LNA Probe Design: Comprehensive Overview in Molecular Biology
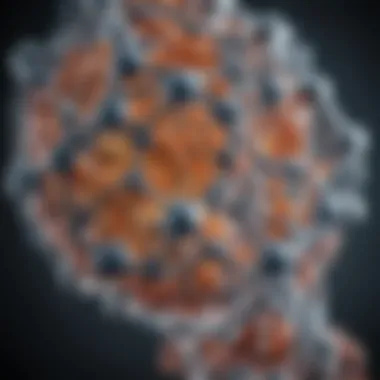
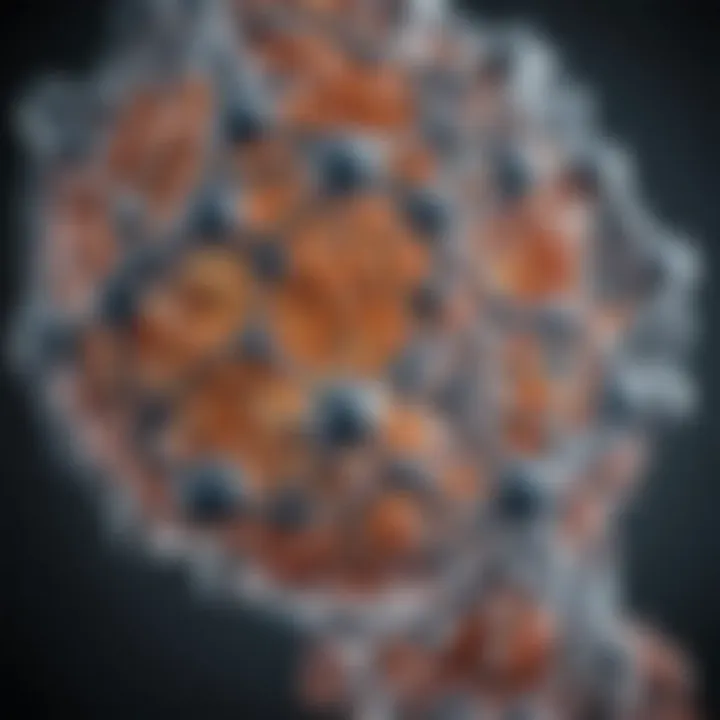
Intro
LNA, or Locked Nucleic Acids, have emerged as a pivotal component in molecular biology and biotechnology. Designed to enhance the binding affinity and specificity of nucleic acid probes, these molecules present significant advantages in various applications, particularly in diagnostics and therapeutics. This article aims to dissect the intricacies of LNA probe design, illustrating its relevance and importance in current research.
Research Overview
Research on LNA probes reveals their superior properties compared to traditional DNA and RNA probes. Several studies show that LNAs can bind to complementary nucleic acid sequences with greater strength and stability. This characteristic is critical in assays where precision is paramount, such as in detecting low-abundance targets in complex biological samples.
Key findings in the area include:
- Increased Binding Affinity: LNA probes can demonstrate up to five-fold enhanced binding over standard DNA or RNA probes.
- Enhanced Specificity: The unique structure of LNAs minimizes off-target hybridization, leading to more reliable results in diagnostic procedures.
- Versatility in Applications: LNAs are beneficial not only in diagnostics but also in research applications where precise nucleic acid recognition is essential.
The significance of this research extends beyond mere academic interest. LNAs bridge crucial gaps in genetic analysis, enabling advancements in personalized medicine and targeted therapy. The implications of these findings underscore the necessity for continued exploration in the field of LNA probe design.
Methodology
Examining the design and implementation of LNA probes involves a comprehensive approach that integrates various experimental and analytical techniques. Researchers typically employ several methodologies, such as:
- Synthesis of LNA Probes: The chemical synthesis of LNAs requires meticulous attention to detail.
- In Vitro Testing: This involves assessing the binding properties of LNA probes in controlled conditions to establish efficacy.
- Comparative Analysis: Researchers often compare LNA probes to traditional nucleic acid probes to quantify improvements in performance.
- Field Testing: Applications in real-world diagnostic scenarios are essential for validating laboratory findings.
Data collection techniques can include high-throughput sequencing to monitor probe interactions and binding studies using fluorescence or other detection methods. This multi-faceted approach ensures a robust understanding of LNA performance in various contexts.
By integrating insights from diverse studies, this article aims to consolidate knowledge on the design, optimization, and practical applications of LNA probes, ultimately guiding future research endeavors.
Understanding LNA Probes
Understanding locked nucleic acid (LNA) probes is crucial for anyone involved in molecular biology, diagnostics, or biotechnology. These probes are highly specialized tools designed to detect specific nucleic acid sequences with greater affinity and specificity than traditional DNA oligonucleotides. Their unique structure allows them to maintain stability under various experimental conditions, which significantly broadens their applications.
The significance of LNA probes lies in their ability to hybridize tightly with complementary RNA or DNA sequences. This characteristic enables them to outperform conventional probes in terms of binding strength. The increased binding affinity results in more reliable assays for detecting genetic material, such as in various forms of cancer, pathogens, or genetic disorders. The ability to distinguish between closely related sequences enhances specificity, reducing false positives and improving diagnostic accuracy.
When designing LNA probes, it is vital to consider several factors that affect their performance. This includes the length of the probe, the concentration used in experiments, and the nature of the target sequence. Probes that are too long may bind non-specifically, while those that are too short may not bind effectively, illustrating the need for careful optimization. Moreover, understanding the implications of splice variants and single nucleotide polymorphisms (SNPs) is essential in accurately targeting the intended sequences without interference from similar ones.
In summary, LNA probes represent a significant advancement in nucleic acid detection technologies. Their development has opened new avenues for research and diagnostics, making it essential for researchers and clinicians to understand their design, composition, and impact on modern science.
Definition and Composition
Locked nucleic acids are modified oligonucleotides where the ribose moiety is locked in a specific conformation. This modification enhances the stability and binding characteristics of the nucleic acid. The LNA structure consists of a ribose sugar molecule that is constrained by a methylene bridge connecting the 2' and 4' positions.
This unique design provides several important advantages:
- Increased affinity for complementary sequences
- Resistance to degradation by nucleases
- Enhanced specificity, enabling the detection of sequences that may differ by only a single nucleotide
The composition of LNA probes usually includes a blend of LNA nucleotides and standard DNA nucleotides. This combination allows for flexibility in designing probes that are tailored for specific applications, whether it be for genotyping, detecting expressional changes in RNA, or identifying mutations in DNA.
Historical Context
The concept of locked nucleic acids was introduced in the late 1990s. Initial research focused on their potential to improve the binding characteristics of nucleic acid probes. Over time, studies demonstrated that LNA-modified probes significantly outperformed traditional DNA and RNA probes, particularly in hybridization strength and stability. As research continued, applications expanded into various fields, including diagnostics, therapeutics, and genomics.
The growing interest in LNA probes has led to extensive research and development in their synthesis techniques. This development facilitated the production of LNA probes that could be used in practical applications, such as quantitative polymerase chain reaction (qPCR) and fluorescence in situ hybridization (FISH). The integration of LNA technology into existing methodologies has marked a turning point in nucleic acid detection, allowing for advancements in precision medicine and personalized therapies.
Structure of LNA Probes
The structure of LNA probes is a critical aspect that greatly influences their functionality in molecular biology. These probes are designed with a distinct locked nucleic acid structure. This feature is paramount because it enhances the stability and binding properties of the probes, making them suitable for various applications in diagnostics and therapeutics.
Nucleic Acid Modification
Locked Nucleic Acid probes have unique modifications in their backbone structure. The phosphodiester bond in conventional nucleic acids is replaced with a methylene bridge. This modification constrains the nucleotide, thus introducing a structural rigidity that significantly increases binding affinity for complementary RNA or DNA sequences.
The importance of nucleic acid modifications cannot be understated. They not only improve the thermal stability of the probes but also enhance their specificity. Probes with higher specificity reduce the likelihood of non-target binding, allowing for more accurate detection of specific nucleic acid sequences. Furthermore, these modifications allow LNA probes to effectively differentiate between similar sequences, making them valuable in applications where precision is essential, such as in the detection of single nucleotide polymorphisms.
Mechanism of Action
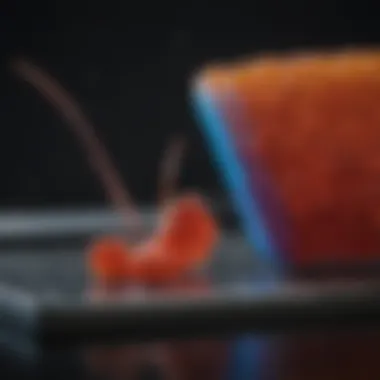
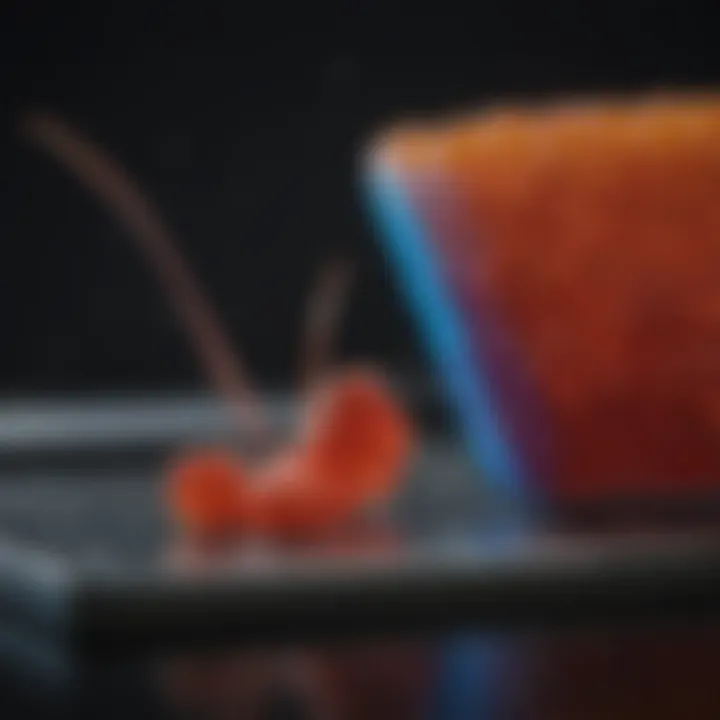
The mechanism of action of LNA probes is rooted in their ability to hybridize with target nucleic acids. When an LNA probe encounters its complementary sequence, it forms a stable duplex. This interaction is facilitated by the rigid conformation of the LNA units, which allows for optimal stacking and hydrogen bonding with the target strand.
The strong binding affinity is a result of the structural adaptation of LNA probes. Their modified backbone creates a higher melting temperature compared to standard nucleic acid probes. This means that LNA probes can remain bound to their targets under less favorable conditions, which further solidifies their position in sensitive assays.
The capability of LNA probes to hybridize at lower concentrations also plays a key role in enhancing the sensitivity of detection methods in various biological assays. Thus, understanding the mechanism of action allows researchers to better tailor probe design to meet specific experimental requirements.
The locked structure of LNA probes makes them particularly advantageous for high-accuracy applications, such as molecular diagnostics and biomarker discovery, where precision is critical.
In summary, the structure of LNA probes, marked by nucleic acid modifications and their mechanisms of action, highlights their importance in the advancement of molecular biology. These characteristics enable higher specificity, thermal stability, and binding affinity, crucial for both research and practical applications in the field.
Advantages of LNA Probes
LNA probes have gained significant attention in molecular biology and biotechnology. Their unique characteristics contribute to various advantages that enhance the effectiveness of molecular detection and analysis. Understanding these advantages helps in recognizing the critical role that LNA probes play in research and clinical applications.
Increased Binding Affinity
One of the most significant advantages of LNA probes is their increased binding affinity. Compared to traditional DNA or RNA probes, LNA probes can bind more tightly to their target sequences. This heightened affinity arises from the locked structure of the Nucleic Acid, preventing the formation of unwanted secondary structures. Consequently, LNA probes can achieve effective hybridization even in complex biological samples. This increased affinity translates to improved sensitivity in assays, which is essential for accurate detection of low-abundance targets.
Moreover, studies demonstrate that LNA probes can tolerate mismatches better than conventional nucleic acid probes. This quality allows researchers to use them in scenarios where targets may have point mutations or variations, ensuring reliable results.
Enhanced Specificity
Another essential benefit of LNA probes is enhanced specificity. The structural modifications in LNA enhance the discrimination between closely related sequences. This specificity is crucial in applications where distinguishing between similar sequences can have profound implications, such as in diagnostics and genotyping.
LNA's ability to maintain sequence specificity under varying conditions leads to fewer false positives. As a result, researchers and clinicians can trust the outcomes of their assays more fully, leading to more accurate diagnoses and targeted therapeutic approaches. This specificity is particularly valuable in distinguishing splice variants or analyzing single nucleotide polymorphisms (SNPs), where even a slight change in the sequence can impact biological outcomes significantly.
Thermal Stability
Thermal stability is another prominent advantage of LNA probes. The locked structure of LNA not only improves binding affinity and specificity but also contributes to the thermal stability of the probe-hybrid complex. This stability allows LNA probes to remain intact under conditions that might lead other probe types to lose integrity or function.
The improved thermal stability of LNA probes makes them suitable for assays that require stringent conditions. For instance, they can withstand higher temperatures during processes like PCR or in hybridization techniques, facilitating their use in diverse experimental contexts.
Design Considerations
Designing LNA probes requires meticulous attention to several critical factors. These considerations directly influence their effectiveness in hybridization, specificity, and overall utility across different applications. A comprehensive understanding of these design elements is essential for maximizing the performance of LNA probes within various experimental frameworks.
Probe Length and Concentration
The length of the LNA probe plays a decisive role in its binding characteristics. Shorter probes may lead to quicker hybridization but can also come with compromised specificity. Conversely, longer probes tend to provide enhanced specificity and stability in binding to target sequences. The optimal length, therefore, should balance these attributes, typically ranging from 18 to 25 nucleotides.
Concentration also significantly impacts the binding efficiency of LNA probes. An effective probe concentration must be determined for the specific assay conditions. A high concentration enhances detection sensitivity, yet it can also increase background noise. Hence, careful optimization based on experimental requirements is crucial.
Target RNA or DNA Sequence
Identifying the target RNA or DNA sequence is paramount in LNA probe design. The choice of target should consider the sequence's uniqueness to avoid nonspecific binding. For instance, target regions that are highly conserved will likely lead to unintended cross-hybridization with similar sequences.
Consideration should also extend to the GC content and melting temperature (Tm) of the target sequence. A balanced GC content favors stable binding, while understanding Tm ensures that the hybridization occurs at the appropriate temperatures during experiments. Thus, analyzing the target sequence comprehensively is vital to achieving optimal probe performance.
Splice Variants and Single Nucleotide Polymorphisms
In complex organisms, splice variants can pose significant challenges for probe design. Splice variants, arising from alternative splicing, can lead to different mRNA transcripts that may share high sequence similarity yet exhibit unique functional outcomes. Designing probes that distinguish between these variants is crucial.
When considering single nucleotide polymorphisms (SNPs), LNA probes can be tailored to selectively bind to specific alleles. Such specificity is integral for applications in genotyping and disease association studies. Understanding the context of these variations can influence probe design to enhance their efficacy in diagnostic applications.
Key Takeaway: The effectiveness of LNA probes depends heavily on the meticulous design considerations that involve probe length, target sequence characteristics, and the capability to distinguish between variants and SNPs. Each of these aspects must be thoughtfully integrated into the probe design process to ensure successful results in various applications.
Synthesis of LNA Probes
The synthesis of LNA probes is a critical process that lays the foundation for their application in molecular biology and biotechnology. Understanding this process is essential for researchers and professionals who aim to use LNA technology effectively. The significance of LNA probe synthesis lies in its ability to enable the production of highly specific and efficient probes, necessary for accurate nucleic acid detection and quantification. Through careful design and rigorous synthesis methods, it is possible to produce LNA probes that offer enhanced stability, higher binding affinity, and improved specificity compared to conventional DNA or RNA probes.
Chemical Synthesis Techniques
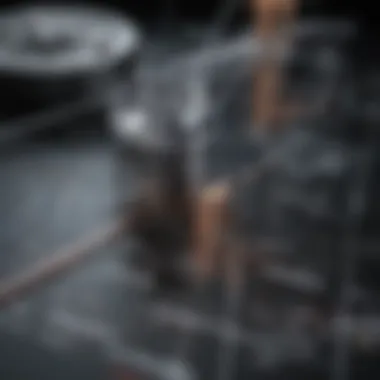
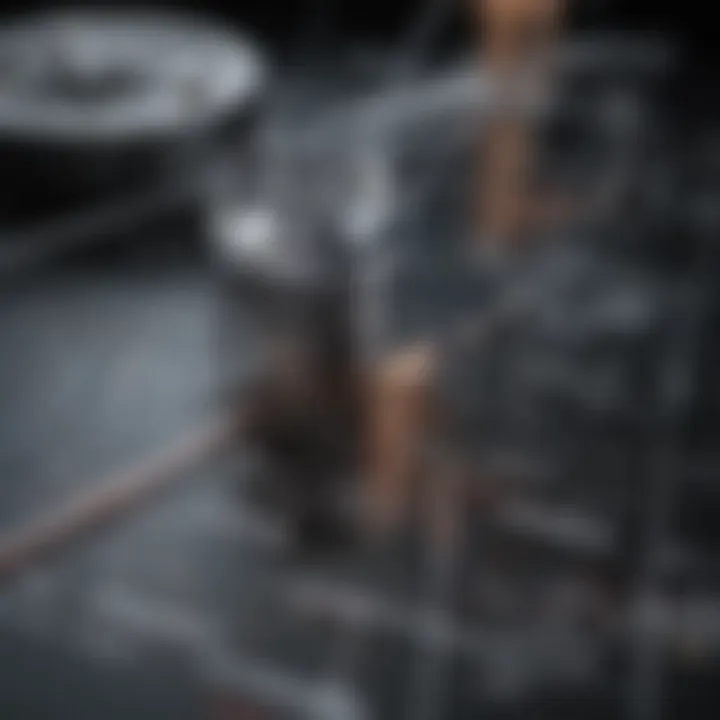
Chemical synthesis techniques form the core of LNA probe fabrication. The predominant method for synthesizing these probes is solid-phase synthesis. This approach allows for the sequential addition of nucleotides onto a solid support material. The solid-phase synthesis of LNA probes involves several key steps:
- Initial attachment: The first nucleotide is attached to a solid support, typically a silica or polystyrene resin.
- Nucleotide addition: Successive nucleotide units are added, following a phosphoramidite approach. Each addition involves a series of chemical reactions, including deprotection, coupling, and oxidation.
- Incorporation of LNA modifications: During nucleotide assembly, Locked Nucleic Acids are incorporated into the sequence. This modification enhances binding characteristics.
- Cleavage and purification: After completing the desired sequence, the probes are cleaved from the solid support and undergo purification to remove excess reagents and byproducts.
Through these techniques, synthesizing LNA probes can maintain the strict quality necessary for subsequent applications in diagnostics and therapeutics.
Quality Control Measures
Quality control measures are paramount when synthesizing LNA probes. These measures ensure that the final product meets the required standards of purity, yield, and functionality. The absence of rigorous quality control can lead to variability in probe performance, potentially affecting experimental outcomes. Here are several key aspects of the quality control process:
- Monitor synthesis progress: Real-time analytical methods, such as thin-layer chromatography or high-performance liquid chromatography, are employed to check the progress of the synthesis, identifying any issues early.
- Purity assessment: After synthesis and purification, the probes are analyzed for purity using techniques such as mass spectrometry and gel electrophoresis. This step confirms that the synthesized probes match the intended sequence and composition.
- Functional testing: The biological activity of the probes is validated through in vitro testing, measuring their binding affinity to target nucleic acids. This ensures that the probes can successfully perform their intended applications.
- Stability evaluations: Finally, stability tests are conducted to assess the longevity and reliability of the probes under various storage conditions. Stability is a critical factor in determining the shelf-life and usability of the LNA probes.
Effective quality control during the synthesis of LNA probes is essential for obtaining reliable results in subsequent applications.
Overall, the synthesis of LNA probes involves a detailed interplay of chemical techniques and quality assurance measures. Combining these ensures that the synthesized LNA probes are effective tools for precise molecular analysis and modular applications.
Validation of LNA Probes
The validation of LNA probes is a crucial component in ensuring their efficacy and reliability in various applications, especially in molecular diagnostics and therapeutic developments. This process not only confirms the performance of LNA probes but also provides insights into their specificity, sensitivity, and overall utility in biological research. A well-validated LNA probe guarantees that it binds accurately to its target sequence, leading to precise experimental outcomes. The subsequent sections discuss in detail the methods of validation, as well as the implications of these validations in both in vitro and in vivo contexts.
In Vitro Testing
In vitro testing plays a significant role in the assessment of the functionality of LNA probes. This evaluation occurs outside of a living organism, often conducted in controlled laboratory settings. The primary goal is to determine how well these probes can bind to their target nucleic acids.
A few elements are important here:
- Binding Affinity: It is critical to understand how strong the interaction is between the LNA probe and its target. High binding affinity often translates to more reliable results.
- Specificity: In vitro testing assesses whether the LNA probe can differentiate between closely related sequences, such as splice variants or mutations. A probe with high specificity minimizes cross-reactivity, which is vital in applications such as diagnostics.
- Temperature Stability: It is necessary to evaluate how temperature fluctuations affect the probeโs performance, as this can impact results during real-world applications.
"In vitro testing is often the first line of defense in ensuring that LNA probes perform as intended."
Such assessments help refine the probe design before proceeding to more complex in vivo validations. Additional techniques such as gel electrophoresis and quantitative PCR are commonly utilized to quantitatively measure these parameters, ensuring that the probe can accurately serve its intended purpose in real-world applications.
In Vivo Applications
The transition from in vitro to in vivo applications signifies a major step toward understanding how LNA probes behave within living organisms. Validating LNA probes in vivo presents unique challenges due to the complexity of biological systems. Nonetheless, this stage is essential for translating laboratory success into therapeutic potential.
Key considerations include:
- Efficacy: The probe must demonstrate that it can effectively target and bind to the intended nucleic acid within a biological environment.
- Safety: It is paramount to assess any adverse effects that may arise from the use of LNA probes in living organisms. Consequently, careful monitoring is essential.
- Bioavailability: This refers to how well the LNA probe can be distributed throughout the organism. Factors such as the probe's stability and its mechanism of action in physiological conditions play a crucial role in determining bioavailability.
In vivo testing often involves animal models for early-phase studies. Results from these experiments significantly influence further clinical developments. The insights gained help in refining probe formulations and optimizing delivery methods, ensuring that they can function as desired in pathway modulation or disease treatment.
Overall, both in vitro and in vivo validations are fundamental to the LNA probe design process. They provide necessary proof of performance, assuring researchers and clinicians that the probes can meet the demands of their applications in real-world environments.
Applications of LNA Probes
LNA probes play a crucial role in various fields, particularly in molecular diagnostics, therapeutic developments, and research applications. Their unique properties facilitate more accurate detection of nucleic acids, thereby enhancing the effectiveness of assays and treatments. It is essential to understand these applications to fully appreciate the implications of LNA probe design in modern biology and medicine.
Molecular Diagnostics
Molecular diagnostics encompasses a range of techniques to analyze genetic material. LNA probes are particularly valuable in this field due to their high specificity and binding affinity. They can detect low levels of target sequences, which is especially important in early disease detection. For instance, when screening for specific mutations or pathogens, LNA probes can provide reliable results that reduce false positives.
The integration of LNA probes into assays like quantitative PCR has become increasingly common. They improve sensitivity and specificity, facilitating the identification of rare genetic variants. Consequently, they help tailor treatments in personalized medicine, making applications in molecular diagnostics more effective.
- Advantages of LNA Probes in Diagnostics:
- High specificity reduces false positive rates.
- Enhanced binding affinity increases detection limits.
- Useful for low-abundance target detection.
Therapeutic Developments
In therapeutic applications, LNA probes are being explored for their potential in gene therapy and targeted treatment strategies. Their ability to selectively hybridize with specific RNA targets allows for precise modulation of gene expression. This feature holds promise in treating various diseases, including cancer and genetic disorders.
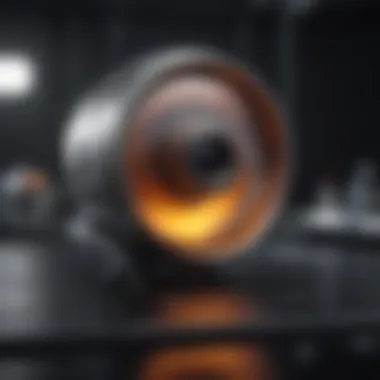
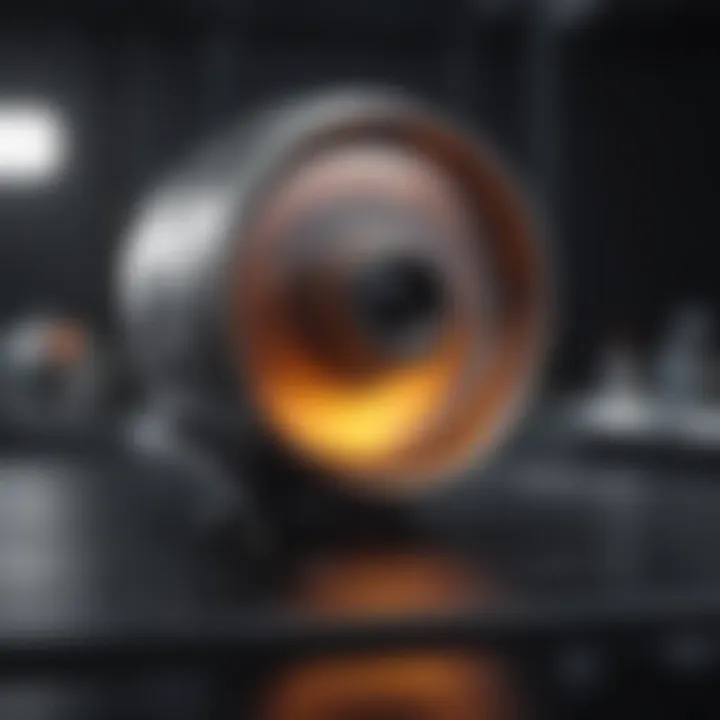
One significant application is the development of LNA-based antisense oligonucleotides that can inhibit mRNA translation. By binding to the mRNA, these probes can effectively block the synthesis of proteins associated with certain diseases. Moreover, since LNAs are chemically modified, they exhibit increased stability, which is beneficial for therapeutic use compared to traditional oligonucleotides.
- Key Benefits for Therapy:
- Targeted modulation of gene expression.
- Increased stability offers prolonged activity in vivo.
- Potential for less off-target effects.
Research Applications
Research in molecular biology benefits significantly from LNA probes. Their ability to provide insights into complex biological systems makes them essential tools in molecular studies. For instance, LNA probes are utilized in studies exploring gene function, expression levels, and interactions among various nucleic acids.
The versatility of LNA probes aids in various applications, such as in situ hybridization and fluorescence in situ hybridization (FISH). These techniques are utilized to visualize nucleic acid locations within cells or tissues, helping researchers understand cellular processes better. Additionally, the capacity to detect single nucleotide polymorphisms using LNA probes can contribute to genetic studies, enabling researchers to map genetic variations and their implications in disease.
- Applications in Research:
- Understanding gene expression patterns.
- Investigating nucleic acid interactions.
- Visualizing cellular structures using FISH.
Current Trends and Innovations
Current trends and innovations in LNA probe design reveal significant advancements in synthesis methods as well as the integration of LNA with other technologies. These elements shape the future of research and application in molecular biology, making it crucial for educators, students, researchers, and industry professionals to stay informed. Enhanced synthesis techniques not only improve the efficiency of probe production but also elevate the overall quality and functionality of the probes. The ongoing integration with other technologies indicates a multidisciplinary approach that can lead to groundbreaking developments in diagnostics and therapeutics.
New Synthesis Methods
The evolution of synthesis methods for LNA probes plays a key role in their efficiency and applicability. Recent methodologies have emerged, focusing on streamlined processes that reduce production time and costs. For example, the adoption of solid-phase synthesis has gained traction. This method allows for easier automation and scalability while minimizing the chances of error that can occur during manual synthesis.
Moreover, advancements in chemical techniques, such as phosphoramidite chemistry, have refined the assembly of LNA monomers. This enhancement directly correlates to the efficacy of the probes, optimizing factors like binding affinity and specificity. It is critical to understand these synthesis techniques, as they form the foundation on which high-quality probes are built, thus impacting their ultimate utility in research and clinical settings.
"Innovative synthesis methods can transform the landscape of molecular diagnostics, making tests faster and more accurate."
Integrating LNA with Other Technologies
The integration of LNA probes with other technologies creates unique and effective solutions for various applications. The fusion of LNA with CRISPR technology, for instance, has revolutionized genetic editing by enhancing the precision of target identification. This collaboration allows for more targeted interventions while reducing off-target effects, which is essential in therapeutic contexts.
Additionally, combining LNA probes with high-throughput sequencing technology enables researchers to analyze vast amounts of data more effectively. This synergy enhances our ability to detect and quantify specific nucleotide sequences, allowing for advanced molecular diagnostics and genomic studies. As LNA probes continue to integrate with other innovative technologies, the potential for new discoveries expands, paving the way for future advancements in healthcare and scientific research.
Challenges and Limitations
Understanding the challenges and limitations associated with LNA probe design is essential for researchers and practitioners in the field. Even though LNA probes offer numerous advantages like enhanced specificity and increased binding affinity, they do not come without significant challenges.
Cost Implications
The synthesis of LNA probes can be more expensive compared to traditional probes such as DNA or RNA. The chemical modifications in LNA increase both the material costs and the complexity of the synthesis process. This financial barrier can limit accessibility for some researchers, particularly in smaller laboratories or in developing regions. Institutions must weigh the advantages of using LNA probes against the budget constraints. It's important to consider how the increased cost can impact the scale of experimentation. Many projects may have to compromise or reduce sample sizes due to budget limitations. Additionally, when considering the long-term benefits of LNA probes, such as improved assay performance, the upfront cost may be justified. However, this rationale does not always alleviate budget pressure in practical settings.
Stability Issues
Although LNA probes exhibit higher thermal stability compared to conventional nucleic acid probes, they are not immune to stability issues. Environmental factors, such as temperature fluctuations and exposure to nucleases, can affect their integrity and functionality. This raises concerns particularly in long-term studies or storage conditions. Moreover, the stability of LNA probes during hybridization can vary depending on the target sequences and buffer systems used. For instance, high salt concentrations can sometimes lead to unexpected hybridization behavior.
Researchers must account for these stability concerns during the experimental design phase. Ensuring that the experimental conditions are optimized is crucial to mitigate any adverse effects on probe performance. Therefore, it is advisable to conduct thorough validation and optimization under various environmental conditions to ensure reproducibility and reliability of results.
In summary, while LNA probes provide significant benefits, awareness of their cost implications and stability challenges is key to leveraging their full potential in research and applications.
Future of LNA Probe Design
The future of LNA probe design is pivotal in continuing advancements in molecular biology and biotechnology. As research progresses, the demand for more accurate, efficient, and versatile tools in diagnostics and therapeutic research grows. LNA probes offer specific benefits that can be expanded with new technologies. This section will explore the emerging technologies and potential developments shaping the future of LNA probe design.
Emerging Technologies
Emerging technologies play a huge role in enhancing the capabilities of LNA probes. Here are some significant trends and their implications:
- Automation of Probe Synthesis: New automated systems will allow for faster and more efficient synthesis of LNA probes. This can lead to reduced costs and increase accessibility for research laboratories.
- CRISPR Integration: The combination of LNA probes with CRISPR technology opens up possibilities for enhanced gene editing. This partnership can facilitate precise targeting of genetic sequences, leading to successful edits and modifications in organisms.
- Nanotechnology Applications: Integrating nanotechnology can elevate the sensitivity and specificity of LNA probes. Nanoparticles functionalized with LNA can improve the detection limits in various applications.
Each of these technologies aims to enhance not only the performance of LNA probes but also their applicability across a wider range of fields and experiments.
Potential Developments in Research and Industry
The potential advancements in research and industry related to LNA probes are multifaceted:
- Personalized Medicine: As the shift towards personalized medicine continues, LNA probes will be essential in diagnostics. Their ability to precisely target specific biomarkers can lead to tailored treatment plans for patients.
- Increased Use in Agriculture: LNA probes could be employed to identify and modify genetic traits in crops. This could lead to higher yield and resistance to diseases, which is crucial for food security.
- Regulatory and Ethical Considerations: As usage grows, accompanying developments in regulation will be necessary. Ethical considerations in human genetic editing and agriculture must converge to ensure responsible applications.
Innovations in LNA probe design not only enhance existing methodologies but also pave the way for groundbreaking applications in diagnostics and therapeutics.