Protein Purification Techniques in E. coli
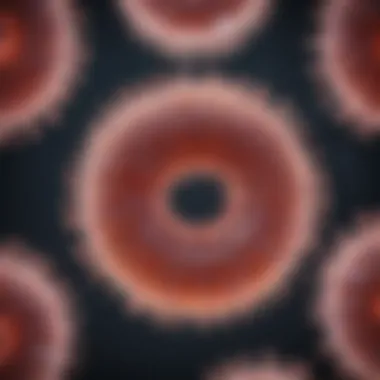
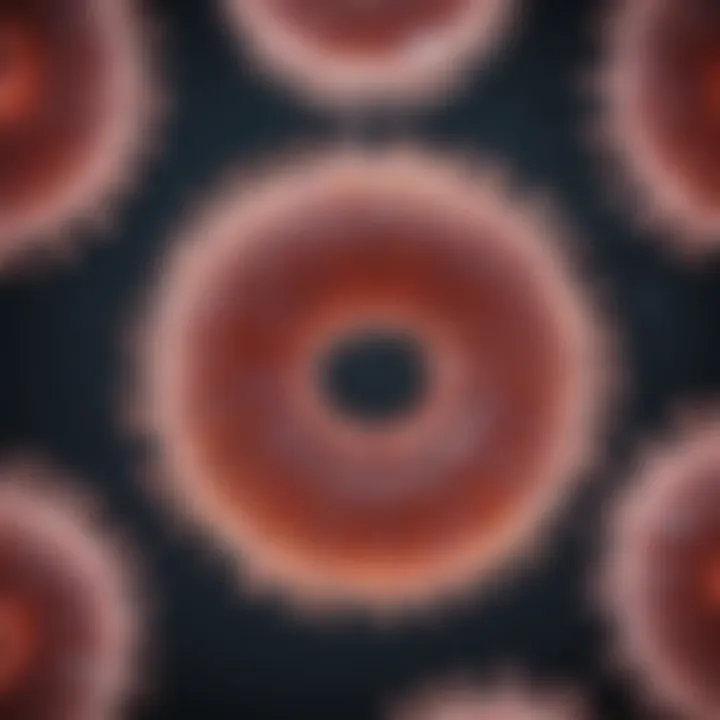
Intro
Protein purification is a cornerstone of molecular biology and biotechnology. Using Escherichia coli (E. coli) as a host organism for the production and purification of proteins has become increasingly popular due to its rapid growth and genetic tractability. This article delves into various techniques and applications related to protein purification in E. coli, offering a comprehensive guide suitable for both seasoned researchers and newcomers.
Research Overview
Summary of Key Findings
One of the key findings in protein purification is the importance of selecting the appropriate purification strategy. Techniques like affinity chromatography, ion exchange, and gel filtration play pivotal roles. Each method has unique characteristics that can significantly affect the yield and purity of the target protein. Furthermore, optimizing these processes often involves tweaking variables such as pH, temperature, and salt concentration, to achieve the best results.
Importance of the Research in Its Respective Field
The research into effective protein purification methods is crucial due to the diverse applications of purified proteins. In therapeutic development, purified proteins can be used as drugs or vaccine components. In industrial settings, enzymes modified for specific tasks can enhance production processes. Thus, advancements in purification techniques not only bolster scientific understanding but also drive innovation across different fields.
Methodology
Description of the Experimental or Analytical Methods Used
Protein purification in E. coli often begins with the culture of transformed bacterial cells expressing the target protein. Common methods deployed include:
- Cell Lysis: Mechanical or chemical means are used to break open bacterial cells and release intracellular proteins.
- Clarification: Centrifugation is employed to remove cell debris, allowing for the supernatant containing the soluble proteins to be collected for further purification.
- Chromatographic Techniques: This encompasses various forms including:
- Affinity Chromatography: Utilizes specific interactions between the target protein and a ligand attached to a solid support.
- Ion Exchange Chromatography: Exploits the charge properties of proteins to achieve separation.
- Size Exclusion Chromatography: Separates proteins based on their size as they pass through a porous material.
Sampling Criteria and Data Collection Techniques
When purifying proteins, careful selection criteria must be established to ensure the purity and functionality of the end product. The initial sample collected from cell lysis should be representative of the entire culture. Throughout the purification process, fractions are analyzed using methods like SDS-PAGE to assess purity levels and protein yield.
"Optimizing protein purification can lead to significant improvements in yield and purity that have a strong impact on the downstream applications."
As a result, controlled sampling and precise techniques ensure that the data collected reflects the effectiveness of the purification strategy used.
This focus on methodology not only illustrates the steps involved but also underscores the importance of rigorous experimental design in the field of protein purification.
Prelude to Protein Purification
Protein purification is a critical aspect of biochemistry that enables the study and application of proteins in various fields. This process involves isolating a specific protein from a complex mixture, which often includes other proteins, nucleic acids, and various cellular components. In the context of using Escherichia coli as a host organism, the purification process becomes both intriguing and essential. E. coli's rapid growth rate and well-understood genetics make it an ideal candidate for protein expression, allowing researchers to produce larger quantities of proteins for analysis.
The significance of protein purification extends beyond merely isolating proteins. It serves as a gateway to understanding protein structure, function, and interaction, which are vital for advancements in drug development, molecular biology, and biotechnology. The ability to purify proteins can lead to the development of therapeutic agents, industrial enzymes, and diagnostic tools. Therefore, this article provides a detailed examination of the methodologies and techniques involved in protein purification using E. coli, shedding light on their applications in both research and industry.
Overview of Protein Purification
Protein purification can be described as a multi-step process designed to attain a protein in its purest form. This process often begins with cell lysis, where the cells are broken open to release their content. Common methods for cell lysis include sonication, freeze-thaw cycles, and enzymatic digestion. Once the cells are lysed, scientists use various purification techniques to separate the target protein from other cellular components.
Common techniques employed in protein purification include:
- Affinity chromatography
This method leverages specific binding interactions between the protein of interest and a ligand attached to a stationary phase. It allows for high specificity and yield. - Ion exchange chromatography
In this technique, proteins are separated based on their charge. The protein mixture is applied to a column containing charged resin, facilitating elution based on the protein's isoelectric point. - Size exclusion chromatography
Also known as gel filtration, this method separates proteins according to their size. Smaller proteins pass through the column more slowly, whereas larger ones elute first, allowing for easy separation. - Precipitation techniques
These include methods such as ammonium sulfate precipitation, which helps to concentrate proteins by selectively precipitating them out of solution.
By using these methods sequentially, researchers can achieve a high level of purity while maintaining protein activity. The choice of purification technique or combination of techniques may depend on the specific properties of the target protein.
Importance in Research and Industry
The importance of protein purification cannot be overstated. In research, purified proteins are essential for elucidating biochemical pathways and conducting experiments aimed at understanding cellular processes. For example, studying enzyme kinetics requires purified enzymes to evaluate their activity under various conditions. Without purification, researchers may be unable to draw clear conclusions about the function of the protein in question.
In the industrial realm, purified proteins are used in developing therapeutic agents, including monoclonal antibodies and vaccines. Biopharmaceutical companies rely on the ability to produce and purify proteins efficiently to meet regulatory standards and patient needs. The enzymes produced through purification processes are also valuable in various industries, such as food and biofuel production.
"Purified proteins are the building blocks of future therapies and innovations in biotechnology."
In summary, protein purification is not just a technical necessity; it is the bedrock upon which scientific inquiry and innovation are built. This article will explore various techniques, factors affecting purification, and the applications of purified proteins in unbeatable depth.
Fundamentals of E. coli as a Host System
Understanding the fundamentals of Escherichia coli as a host system is crucial in the context of protein purification. The organism serves as a valuable tool in molecular biology and biotechnology, precisely due to its unique properties and the advantages it offers for protein expression and purification. The ease of genetic manipulation in E. coli, alongside its rapid growth rate, makes it an ideal candidate for producing recombinant proteins. This section focuses on both the characteristics that define E. coli and the processes that enable efficient manipulation for research and industrial applications.
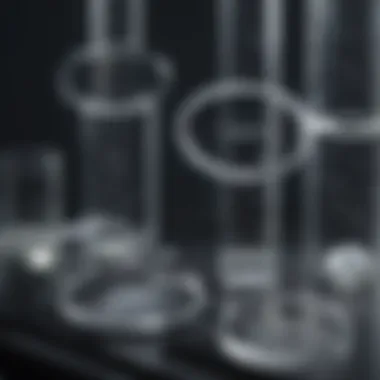
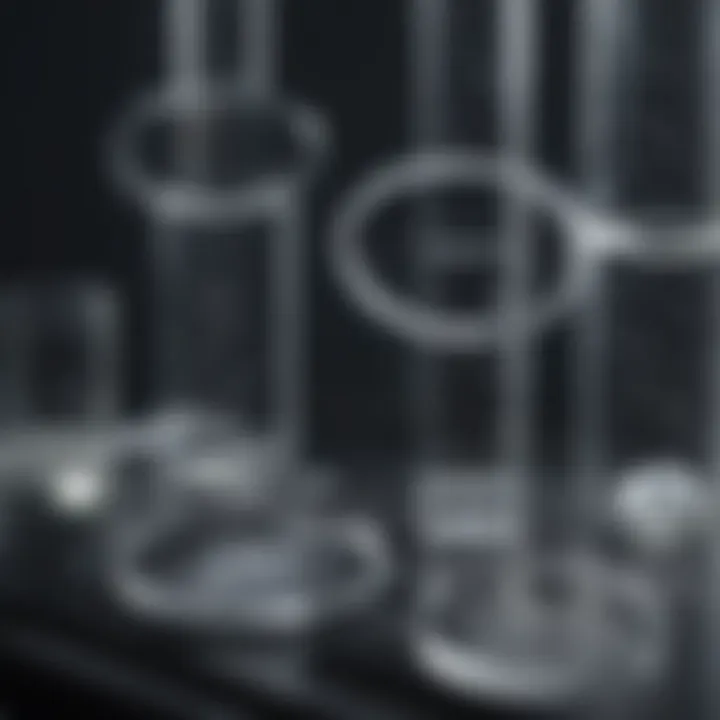
Characteristics of E. coli
E. coli is a gram-negative bacterium known primarily for its role in several fundamental biological processes. One of its most significant characteristics is its uncomplicated genetic structure. Its genome has been sequenced, and the bacterium exhibits a well-understood metabolic profile. The following points encapsulate its essential features:
- Rapid Growth: E. coli can double in number every 20 minutes under optimal conditions, facilitating quick experiments and analysis.
- Genetic Simplicity: The manageable size of its genome allows for straightforward genetic manipulation.
- Versatility: E. coli can grow in various media and conditions, permitting flexibility in protein expression platforms.
- Protein Folding: The presence of chaperon proteins aids in proper protein folding, an important aspect for functional recombinant proteins.
- Safety: Laboratory strains, such as E. coli K-12, are non-pathogenic, which reduces biohazard risks in research environments.
Overall, these characteristics underlie E. coli’s significant role in protein purification processes.
Genetic Manipulation in E. coli
The potential for genetic manipulation is perhaps the most critical factor that establishes E. coli as a prime host for protein purification. Advances in techniques allow researchers to modify the genetic code easily, thus creating strains engineered for specific tasks. Here are some relevant considerations:
- Plasmid Vectors: The use of plasmids enables the introduction of foreign genes into E. coli, greatly facilitating the expression of target proteins. Commonly used plasmids include pUC19 and pGEX series, which help in cloning and protein expression, respectively.
- Gene Expression Control: Various promoters can be utilized to control gene expression levels. For instance, the T7 promoter combined with the E. coli T7 RNA polymerase allows for high-level expression of recombinant proteins.
- Selectable Markers: Antibiotic resistance genes often accompany plasmids, allowing for the selection of transformed cells effectively. This aspect streamlines the isolation of bacteria harboring the desired genetic material.
- CRISPR/Cas9 Technologies: Recent advancements in editing technologies enable targeted genomic modifications. This precision opens pathways for more complex protein engineering tasks.
In summary, genetic manipulation in E. coli is both accessible and versatile, which significantly enhances the efficiency of protein purification efforts.
The combination of E. coli characteristics and the ease of genetic manipulation positions this bacterium as a leading choice in protein purification methodologies.
Common Techniques for Protein Purification
Protein purification is essential in the field of molecular biology and biotechnology. It involves separating specific proteins from a mixture, to enable further analysis or application. Proper understanding of these techniques is crucial for many research and industrial applications. In this section, we discuss four commonly used techniques in E. coli, highlighting interesting aspects, their advantages, and important considerations.
Affinity Chromatography
Affinity chromatography is a popular protein purification technique based on specific binding properties of proteins. In this method, a target protein is separated from other cell lysates by exploiting its unique affinity for a certain ligand attached to a stationary phase. This can be a receptor, an enzyme substrate, or an antibody.
This method's major advantage is its high specificity, leading to a high purity level of the target protein. However, some aspects require consideration. The effectiveness of this technique depends largely on the choice of the affinity tag and the ligand used. Moreover, optimization of binding and elution conditions is critical to achieve the desired results.
Ion Exchange Chromatography
Ion exchange chromatography is another widely used method for protein purification. This technique separates proteins based on their net charge at a specific pH. In this method, charged groups on a resin interact with oppositely charged proteins. Proteins bind to the resin while others flow through, allowing for selective retention.
This technique is valuable due to its ability to generate high resolution separations. It can be easily scaled to handle large sample volumes, making it suitable for both research and industrial purposes. Nevertheless, one must carefully monitor pH and ionic strength. Any deviations can lead to unwanted protein loss or co-elution of undesired proteins.
Size Exclusion Chromatography
Size exclusion chromatography (SEC) operates on the principle of molecular size. In this process, the resin has pores that allow smaller molecules to enter, whereas larger proteins are excluded, allowing them to elute first. This technique is critical when one aims to separate proteins while conserving their native conformation.
One significant advantage of SEC is that it does not require harsh conditions, thus minimizing protein denaturation. However, it typically does not provide the high resolution that other methods offer. As such, it is often used as a polishing step after other purification techniques to enhance purity further.
Precipitation Techniques
Precipitation techniques involve the addition of a specific reagent to induce the proteins to aggregate and precipitate out of solution. Common reagents include ammonium sulfate and organic solvents. It's a straightforward and comparatively inexpensive method for protein purification.
These techniques work well for concentrating proteins while removing some contaminants. However, the yield and purity can sometimes be variable. Conditions such as temperature and ionic strength need to be strictly monitored. Though easy to use, one must approach precipitation with caution to avoid protein degradation or loss of activity.
Ultimately, selecting the appropriate technique or combination of techniques for protein purification in E. coli is vital. Each method presents distinct advantages and challenges that can be leveraged depending on the specific goals of a research or industrial project.
Factors Affecting Protein Purification
Protein purification is a critical aspect of biochemical research and application. Several factors can significantly influence the effectiveness and efficiency of this process. Understanding these factors allows researchers to optimize their methods and achieve better outcomes in isolating desired proteins from E. coli. This section discusses key elements that are crucial for successful protein purification, including protein characteristics and expression systems.
Protein Characteristics
The unique properties of each protein play a vital role in how effectively it can be purified. Characteristics such as solubility, size, and charge are particularly important. Here are several points to consider:
- Solubility: The solubility of a protein in a solvent affects its stability and behavior during purification. Some proteins may require specific conditions, like pH adjustment or the presence of certain salts, to remain soluble.
- Size: The size of a protein influences the choice of purification methodologies. Larger proteins may be separated via techniques like size exclusion chromatography, while smaller ones necessitate different approaches.
- Charge: Proteins carry different charges at varying pH levels. This characteristic can be exploited in ion exchange chromatography, allowing for effective separation based on charge.
In summary, understanding protein characteristics enables researchers to select appropriate purification techniques and conditions tailored to specific proteins, thereby enhancing purity and yield.
Expression Systems
The expression system used for producing the target protein directly affects the purification process. E. coli is a widely used host organism due to its rapid growth and ease of genetic manipulation. Nonetheless, several systems are worth considering:
- Bacterial Systems: In addition to E. coli, other bacteria can be used, but these may produce proteins that are less soluble or more prone to degradation.
- Yeast and Insect Cells: These systems can provide post-translational modifications that may be necessary for the functionality of certain eukaryotic proteins, impacting purification strategies.
- Mammalian Cells: For complex proteins that require specific folding or modifications, mammalian cell lines might be more effective. However, they complicate the purification due to additional cellular components.
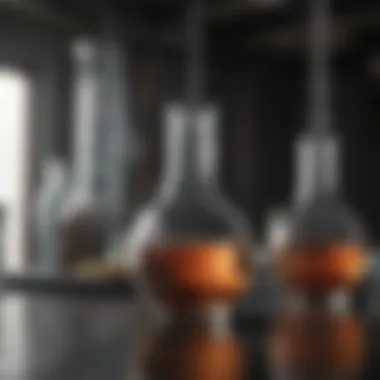
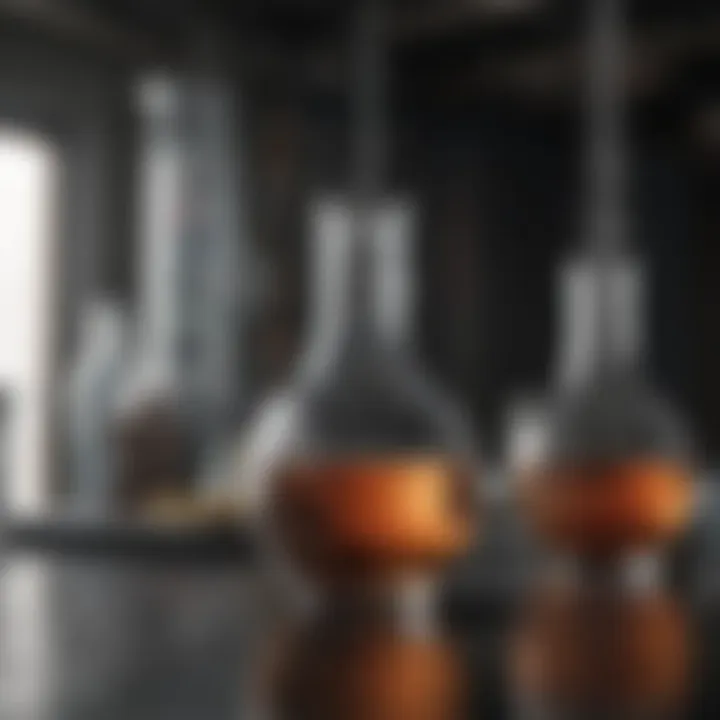
"The choice of expression system can determine the success or failure of the entire protein purification process."
Advanced Protein Purification Techniques
Advanced protein purification techniques are crucial in the field of molecular biology. They enhance purity and yield, allowing researchers to obtain high-quality proteins necessary for various applications. These methods refine the processes described previously. They facilitate more efficient separation and refinement of target proteins from complex mixtures. Understanding these techniques provides insights into the optimal approaches for different proteins, especially when aiming for high specificity or yield.
FPLC and HPLC
Fast protein liquid chromatography (FPLC) and high-performance liquid chromatography (HPLC) represent sophisticated methods for protein purification. FPLC distinguishes itself by being specially designed for protein applications, allowing gentle separation without denaturing biomolecules. This technique utilizes various adsorption columns and media, which are often adjusted based on the target protein's properties, such as size or charge. FPLC is effective for large-scale purification in research contexts or industrial applications, ensuring that proteins retain their functional and structural integrity.
On the other hand, HPLC is widely used due to its versatility across different types of samples. This technique excels in resolving mixtures into their components with high precision. HPLC typically incorporates a wide array of detectors, which can monitor various properties of eluting compounds. When applied to protein purification, HPLC can analyze minute quantities of sample, making it suitable for sensitive studies. Researchers often combine FPLC and HPLC techniques strategically, maximizing the benefits of both methods.
Affinity Tags and Their Use
Affinity tags are engineered peptide sequences or proteins that facilitate the purification process. These tags bind specifically to a ligand on a solid support, enabling the target protein to be separated from unwanted proteins during purification. Common affinity tags include His-tags, GST-tags, and Strep-tags, among others. Each tag has unique binding properties, which can influence the efficiency of purification.
The selection of an affinity tag can depend on multiple factors, including the downstream application of the purified protein and the compatibility with the indicator assays. Researchers must be mindful of potential impacts on protein function, as certain tags can alter the protein's stability or binding characteristics. The use of cleavable tags allows for the removal of the tag after purification, ensuring the protein is in its native form.
Overall, advanced techniques such as FPLC, HPLC, and the use of affinity tags have transformed protein purification. Employing these methods enhances the efficiency and specificity of obtaining high-purity proteins. This becomes even more significant as researchers push the boundaries of science and industry.
Assessing Purification Efficacy
In the realm of protein purification, assessing the efficacy of the purification process is paramount. It ensures that the resulting proteins are not only pure but also functional for their intended applications. High purity is critical, especially when the proteins are used in molecular biology experiments, structural studies, or pharmaceutical applications. Thus, methods used to evaluate the success of these purification strategies must be both reliable and informative.
SDS-PAGE Analysis
SDS-PAGE, or Sodium Dodecyl Sulfate Polyacrylamide Gel Electrophoresis, is one of the most widely employed methods for assessing protein purity. This technique separates proteins based on their molecular weight. During SDS-PAGE, proteins are denatured and negatively charged, allowing them to migrate through a polyacrylamide gel in response to an electric field. The distance a protein travels is inversely proportional to its size.
Benefits of SDS-PAGE analysis include:
- Visualization of Purity: Protein bands can be visualized, showing distinct bands for each protein component present.
- Molecular Weight Estimation: By comparing the migration of the sample to that of known standards, it's possible to estimate the molecular weight of the protein of interest.
- Detection of Contaminants: Any unexpected bands indicate the presence of contaminants or degradation products, prompting further purification if necessary.
SDS-PAGE results are often expressed quantitatively using densitometry, which analyzes the intensity of bands. This can help in determining the relative concentrations of proteins in various fractions throughout the purification process.
Western Blotting
Western blotting is another technique that serves as a powerful tool to assess the efficacy of protein purification. After SDS-PAGE, proteins are transferred to a membrane, typically made from nitrocellulose or PVDF. The membrane is then probed with specific antibodies against the protein of interest, allowing for targeted detection.
Key features of Western blotting include:
- Specificity: This method allows researchers to specifically confirm the presence of a target protein, distinguishing it from other proteins present in the sample.
- Sensitivity: Western blotting can detect low abundance proteins, making it possible to confirm the presence of a protein even when it is present in minute quantities.
- Quantitative Analysis: The intensity of the protein bands that react with the specific antibodies can be quantified, providing an estimate of the protein concentration in the sample.
To summarize, both SDS-PAGE and Western blotting are essential in the assessment of protein purification efficacy. They provide critical insights into the purity and presence of the target protein, ensuring that the purification has been successful and that the proteins are suitable for subsequent applications in research or industry.
"The credibility of any molecular biology experiment hinges on the quality of the reagents used, making the assessment of protein purification a cornerstone of successful research."
These methods help safeguard the integrity of scientific work, enabling researchers to advance the understanding of protein functions and their applications.
Applications of Purified Proteins
The process of protein purification is not merely an academic exercise but is essential for a multitude of applications across various fields. Understanding how purified proteins can be utilized allows researchers and industry professionals to appreciate the broader implications of their work. Purified proteins serve as invaluable resources for myriad tasks, including research, clinical applications, and industrial processes. Each application carries its own set of benefits and considerations, which are crucial for optimizing outcomes in both laboratory and commercial settings.
Research Applications
In the research domain, purified proteins are pivotal in advancing our understanding of biological functions and mechanisms. They provide clarity about cellular processes and enable experimental manipulation. Common uses include:
- Structural Biology: Purified proteins allow for crystallography and NMR studies. Determining the three-dimensional structures of proteins reveals insights into their functions and interactions.
- Enzyme Studies: Investigating the kinetics and mechanisms of enzymatic reactions requires pure enzymes. This knowledge can lead to the design of inhibitors or activators, crucial for therapeutic developments.
- Immunology: Antibodies, often purified, are central for studying immune responses. They can be used in various assays to detect and quantify proteins of interest.
- Biophysics: Techniques like fluorescence or surface plasmon resonance involve purified proteins to study interactions and conformational changes in real time.
Furthermore, purified proteins are essential for developing new technologies, such as biosensors and diagnostic tools. Researchers can engineer proteins to possess desired functionalities, thus enhancing the technological landscape.
Industrial Applications
The industrial sector also benefits significantly from protein purification. Several industries rely on specific proteins to develop products, streamline processes, and ensure quality. Some primary applications are:
- Pharmaceutical Industry: Purified proteins are central to the production of vaccines, enzymes used in drug formulation, and monoclonal antibodies for therapies, making their role absolutely critical.
- Food Industry: Enzymes from purified proteins are employed in various food processing techniques, enhancing flavors and textures or improving nutritional availability in food products.
- Biotechnology and Bioremediation: Purified proteins play a role in environmental cleanup processes and the development of biofuels. Their use in degradation pathways can facilitate waste treatment and resource recovery.
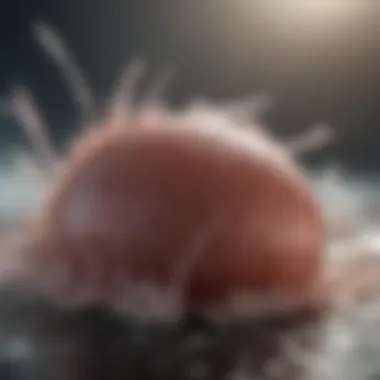
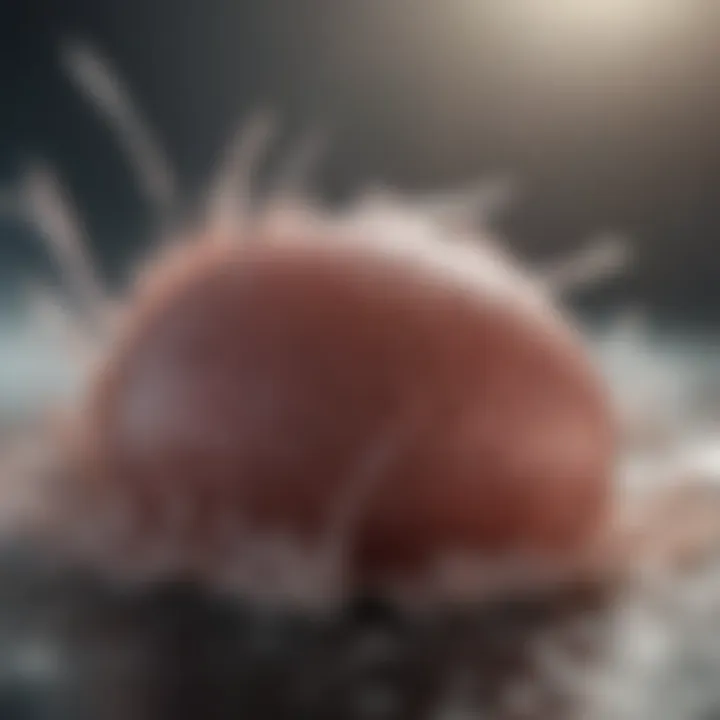
Purified proteins are not only essential for fundamental research, but they also drive innovations in biotechnology and pharmaceuticals.
Challenges in Protein Purification
Protein purification is a complex process and comes with various challenges. Addressing these challenges is vital to enhance the overall effectiveness of purification procedures. Failing to resolve issues such as yield and purity can compromise the reliability of research findings. Furthermore, understanding scalability issues is essential for industrial applications where large quantities of proteins are required. This section delves into these critical challenges, offering insights into overcoming them.
Yield and Purity Issues
The yield of purified protein is a key metric in any purification process. It refers to the amount of target protein obtained after the purification steps relative to the initial amount expressed. Low yields can arise from different issues, including loss during extraction, binding inefficiencies, and suboptimal conditions during chromatography. Moreover, purity is equally important, as contaminants can lead to erroneous results in downstream applications. High purity levels are necessary for critical experiments, such as structural studies or functional assays.
"Achieving both high yield and high purity is often a balancing act. Improvements in one area can sometimes detract from the other, necessitating rigorous optimization of protocols."
To address these challenges, researchers often employ multiple purification techniques sequentially. For instance, starting with a rough purification technique, such as ammonium sulfate precipitation, can help eliminate bulk contaminants before moving to more refined methods like affinity chromatography. This approach can increase the yield while refining the purity progressively. Additionally, modifying the conditions throughout the process, such as pH, ionic strength, and temperature, may lead to improvements in both yield and purity.
Scalability of Processes
Scalability is another prominent challenge in protein purification, especially when transitioning from lab-scale to industrial-scale processes. What works effectively in small volumes may not translate directly when working with larger samples. Here, the equipment and methods used must be capable of handling increased volumes while maintaining efficiency and product integrity. Factors influencing scalability include the type of chromatography, the nature of the protein, and the required throughput.
As the demand for purified proteins grows, advancements in technology must also keep pace to enable larger scale purification without compromising on standards.
A few approaches to enhance scalability are:
- Automation of Processes: Integrating automated systems can improve reproducibility and reduce manual errors during scaling.
- Development of Continuous Flow Systems: Continuous processes can help achieve higher efficiency than batch processes, facilitating larger production scales.
- Utilization of Multi-column Systems: This technique allows simultaneous processing of multiple samples, enhancing throughput.
In summary, the challenges in protein purification require a combination of careful planning and innovative strategies to address issues of yield, purity, and scalability. Addressing these elements will enhance reliability in both research and industrial applications.
Future Directions in Protein Purification
The field of protein purification is continuously evolving. This evolution is significant because successful protein purification is foundational to both research and industrial applications. As scientists seek more efficient and effective methods, identifying future directions becomes crucial. Innovations and trends will shape the landscape of protein purification, ensuring its adaptability to new scientific challenges and opportunities.
Innovations in Techniques
Innovation drives the progress in protein purification methods. New technologies are emerging that enhance both the efficiency and effectiveness of protein purification. For instance, advancements in microfluidics technology are allowing for miniaturized purification methods. This leads to faster processing times and reduced use of expensive reagents.
Another notable innovation is the development of automated purification systems. Automation streamlines processes, decreases human error, and increases reproducibility in workflows. Moreover, these systems can be integrated with real-time monitoring to optimize conditions dynamically.
Recent advancements in chromatography techniques such as monolith chromatography offer improved resolution and throughput. The ability to purify proteins with high specificity while maintaining activity is essential in biopharmaceutical production.
Ultimately, these innovations not only simplify complex procedures but also expand the range of applications for purified proteins, particularly in therapeutic development and structural biology.
Trends in Biotechnology
The biotechnology landscape significantly influences protein purification methods. As the demand for biopharmaceuticals continues to grow, the protein purification field must keep pace. There is an increasing trend toward sustainable and eco-friendly purification methods. This involves using fewer harmful chemicals and reducing waste.
Biotechnology also pushes for more personalized medicine approaches. This means purifying proteins that are specifically tailored to individual patient needs, requiring methods that are flexible and adaptable.
Furthermore, the integration of artificial intelligence in bioprocessing is gaining traction. AI can help predict the best purification strategies based on previous data. This might reduce trial-and-error experiments, accelerating the timeline from research to market.
Future directions in protein purification are shaping the way we approach scientific challenges. The continuous integration of new technologies and sustainable practices holds great promise.
The synergy between innovations and current trends ensures that protein purification is not merely a set of techniques but a dynamic field poised for advancement.
Culmination
In the realm of protein purification, the significance of the Conclusion lays in its ability to tie together the diverse techniques and applications discussed within this article. It serves as a succinct recap of the essential themes that have been explored, highlighting both the complexities and the methodologies associated with purifying proteins in Escherichia coli. The overarching narrative reinforces the idea that while the techniques may be varied, they all converge towards a common goal of achieving high purity and yield in protein extraction.
In summarizing the key points, the Conclusion not only brings closure to the discussion but also emphasizes the relevance of each method presented. It points out that successful protein purification is crucial for advancing research and application in various fields, including pharmaceuticals, biotechnology, and molecular biology. By recognizing the interconnections between the methodologies, readers can better appreciate the importance of selecting the appropriate technique depending on the specifics of their research or industrial objectives.
Summary of Key Points
- Importance of Purification: Efficient protein purification is vital for both research and industrial applications.
- Diverse Techniques: Various methodologies exist, each suited to specific protein characteristics and requirements.
- Impact of Factors: Different factors, including protein structure and the expression system, influence purification outcomes significantly.
- Evolving Approaches: New technologies and innovations continue to emerge, enhancing purification protocols and techniques.
The Conclusion underscores how understanding these key aspects is essential for researchers and practitioners aiming to harness the potential of purified proteins in innovative ways.
Importance of Continued Research
Continued research in protein purification holds paramount significance for the advancement of biotechnology and various scientific disciplines. As the methods of purification evolve, there are numerous benefits to investing time and resources into this area of study.
Research may lead to:
- Refinement of Techniques: Ongoing exploration can improve existing methodologies, enhancing yield and purity.
- Emerging Technologies: New technologies, such as automated high-throughput purification systems, may transform how proteins are purified.
- Broader Applications: As researchers uncover new techniques, the scope of protein application in medicine, agriculture, and industry broadens considerably.
- Cross-Disciplinary Insights: Protein purification research often intermarries with other fields like bioinformatics, leading to novel approaches and insights.