Exploring Resonance Scanners: Function and Future
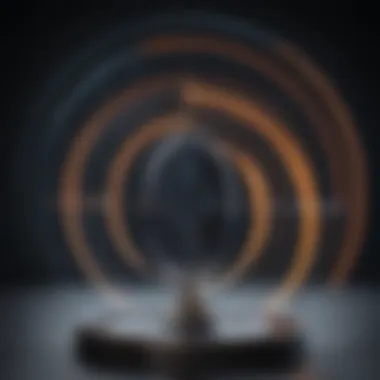
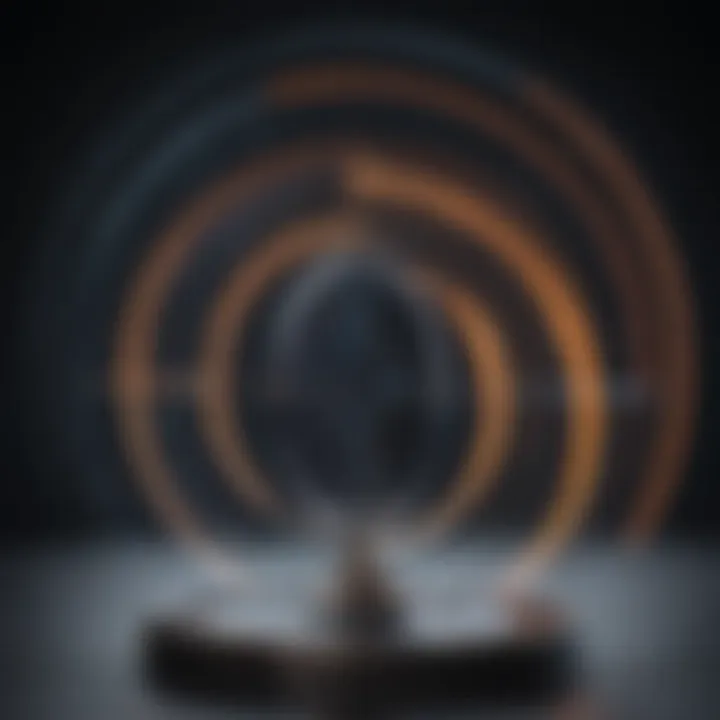
Intro
Resonance scanners play a crucial role in both medical and industrial applications. These advanced devices utilize the principles of resonance to provide detailed analysis and imaging. The significance of resonance scanners cannot be overstated, as they enhance diagnostic capabilities and optimize material evaluation processes. In this article, we will delve into the mechanics of resonance scanning, exploring its fundamental principles, operational mechanisms, and various applications.
Research Overview
Summary of Key Findings
Resonance scanners operate on the premise that certain materials resonate at specific frequencies when exposed to electromagnetic waves. This resonance can be finely tuned, allowing for precise imaging or analysis. Key findings reveal that resonance scanners improve the contrast and resolution of images significantly compared to conventional imaging methods. In addition, their non-invasive nature makes them particularly advantageous in medical diagnostics.
Importance of the Research in its Respective Field
The research surrounding resonance scanners is critical for advancing diagnostic imaging technology. As healthcare continues to evolve, the need for innovative solutions rises. Resonance scanners meet this demand through their ability to provide detailed insights into the internal structures of the body without invasive procedures. Furthermore, industry sectors that use these scanners for material analysis benefit from increased efficiency and accuracy, thus improving product quality and safety.
Methodology
Description of the Experimental or Analytical Methods Used
The methodology for evaluating resonance scanners includes both theoretical calculations and practical tests. Researchers often employ resonance frequency analysis to understand how different materials respond to specific frequencies. These methods include:
- Spectrum Analysis: A method used to determine the resonance frequencies of materials.
- Imaging Techniques: Various imaging modalities are applied to visualize the data collected from resonance scans.
Sampling Criteria and Data Collection Techniques
Sampling for resonance scanner studies is done meticulously to ensure diverse representation of materials. Typically, samples from various biological tissues or synthetic materials are selected. Data collection techniques integrate both qualitative and quantitative approaches, often using control samples to establish baseline resonance frequencies. This enhances the reliability of the overall findings.
Resonance scanning technology exemplifies the confluence of science and engineering, shaping the future of diagnostics and analysis.
Applications of Resonance Scanners
Resonance scanners serve multiple applications across sectors. In medical imaging, magnetic resonance imaging (MRI) is one of the most notable uses. It provides detailed images of internal organs and tissues without exposing patients to ionizing radiation. In industrial settings, resonance scanning is invaluable for assessing material properties, identifying defects, and ensuring structural integrity. Such applications demonstrate the versatility and essential nature of this technology.
Prologue to Resonance Scanners
Resonance scanners have emerged as a significant technological advancement in diagnostic imaging and material evaluation. Their ability to provide detailed information without invasive procedures makes them invaluable across various sectors. This introduction serves as a foundation for understanding resonance scanners, highlighting their mechanics and diverse applications. The importance of this topic lies in its broad implications, including their impact on medical diagnostics, industrial processes, and future innovations.
Defining Resonance Scanners
A resonance scanner is a specialized device designed to detect and analyze resonance frequencies in materials, whether biological or synthetic. These scanners utilize principles of resonance to achieve precise imaging and characterization. They can identify subtle differences in material properties, which serves crucial roles in numerous fields. In medicine, resonance scanners facilitate techniques such as Magnetic Resonance Imaging (MRI), allowing for unprecedented views inside the human body. In industry, they improve quality control and help in materials characterization.
The scanner operates based on the interaction between the external stimulus and the natural frequencies of the object being examined. This interaction provides information about the structural and chemical properties, leading to a comprehensive understanding of the material's condition.
Historical Context
The development of resonance scanning technology is rooted in the early 20th century when scientists began to comprehend the principles of resonance. Early applications were primarily in physics and chemistry, focusing on spectral analysis. However, it wasn't until advances in magnetic resonance technology in the 1970s that real progress was made in imaging applications. The introduction of MRI revolutionized medical diagnostics, leading to a non-invasive method for internal examination.
Since then, resonance scanners have evolved significantly. They now incorporate advanced sensor technologies and sophisticated algorithms, enhancing their accuracy and versatility. The integration of digital computing has further transformed how resonance scanning is applied in fields ranging from biomedicine to materials science. Understanding this history underlines how past innovations shape current practices and future developments in resonance scanning technology.
Fundamentals of Resonance Frequency
Understanding the fundamentals of resonance frequency is crucial to grasping the inner workings of resonance scanners. This concept forms the bedrock for various applications, especially in medical imaging and material analysis. Resonance frequency refers to the frequency at which a system naturally oscillates when disturbed. The ability to determine this frequency allows technicians to gather specific information about an object's composition, structure, and mechanical properties.
The importance of resonance frequency lies in its implications for sensitivity and accuracy in scanning technology. By tuning a resonance scanner to the appropriate frequency, practitioners can enhance image clarity. This resonance condition ensures optimal reception of signals emitted from the scanned object, thereby increasing the effectiveness of the diagnostic or analytical process.
Moreover, understanding resonance frequency helps in mitigating the effects of noise, which can often interfere with data accuracy. Techniques to isolate and amplify relevant signals are adopted based on resonance principles, leading to significant advancements in both medical and industrial applications.
Basic Principles of Resonance
The basic principles of resonance involve the interaction between an external force and the natural frequency of a system. When an object is subjected to an external oscillating force at its resonance frequency, it experiences heightened vibrations. This effect has practical applications in various fields, allowing for the precise detection of materials during scanning.
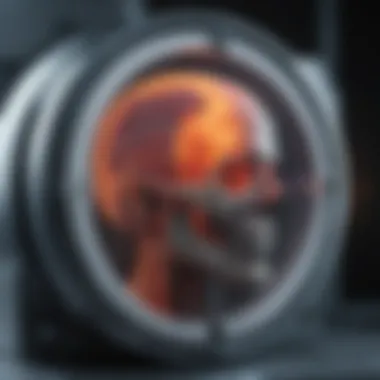
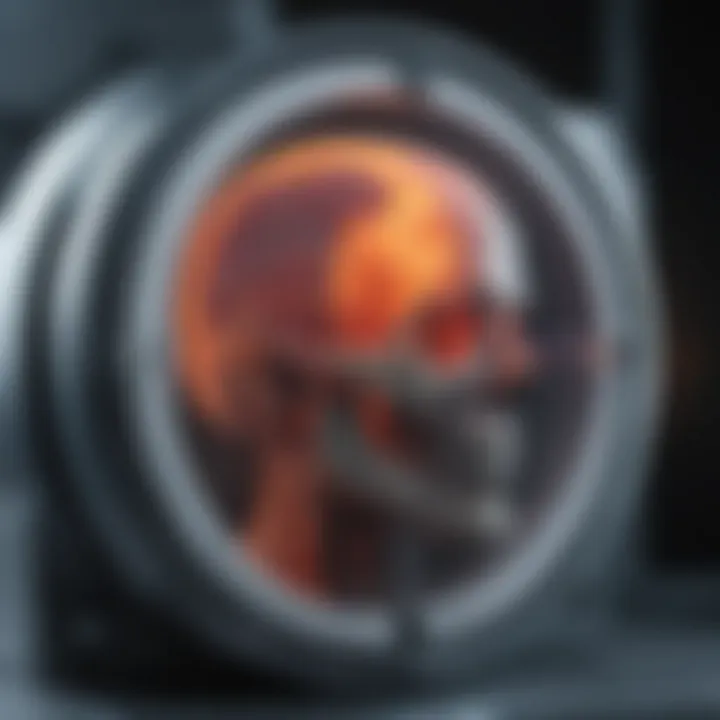
In the context of resonance scanners, these principles dictate how signals are generated and received. An external source emits waves targeting the specific resonance frequency of the object being examined. As the object vibrates, it emits secondary signals that are captured by sensors. This process elucidates valuable information regarding the internal structure and composition.
Understanding these principles also allows for the design of resonant systems that can operate efficiently under different conditions. Factors such as temperature, material composition, and environmental influences can alter resonance properties, and these variations must be accounted for in scanner design.
Mathematical Models of Resonance
Mathematical models play a significant role in articulating the behavior of resonance systems. Various equations describe how objects react under resonance conditions. For instance, the relationship between frequency, mass, and stiffness is fundamental to these models. The basic formula can be expressed as:
- f: Frequency
- k: Stiffness constant
- m: Mass of the object
This equation illustrates that frequency is directly influenced by the physical characteristics of the material. Adjusting either the mass or stiffness alters the system's behavior, hence affecting how resonance scanners operate.
Other mathematical principles, including Fourier analysis, are key to understanding and interpreting the signals captured by resonance scanners. This technique allows for the decomposition of complex waveforms into their constituent frequencies, facilitating the extraction of relevant information regarding the scanned object.
Components of a Resonance Scanner
Understanding the components of a resonance scanner is crucial for grasping how these devices operate and their various applications. Each part plays a significant role in ensuring that the scanner functions effectively and produces accurate results. The fundamental elements include the scanning mechanism, sensor technologies, and control algorithms. Each component serves its purpose, contributing to the overall efficiency and reliability of resonance scanning in both medical and industrial settings.
Scanning Mechanism Overview
The scanning mechanism is the heart of a resonance scanner. It determines how the system gathers data and generates images or readings. It typically consists of a source that produces the necessary energy (like electromagnetic radiation or sound waves), which interacts with the subject being scanned.
- Energy Source: This could be radiofrequency waves in Magnetic Resonance Imaging (MRI) or ultrasonic waves in acoustic resonance techniques.
- Signal Detection: After the energy interacts with the object, the scanner often requires a sophisticated detection system to capture the signals emitted or reflected.
- Data Processing Unit: This second part interprets the received signals, converting them into meaningful data that can generate images or provide analytical results.
The mechanical design must allow for precision and accuracy in achieving effective scans. Proper calibration and alignment are necessary to minimize errors in the collected data.
Sensor Technologies
Sensor technologies used in resonance scanners vary widely depending on the application. The choice of sensors impacts the quality of data collected and the effectiveness of the scanning process. Some common sensor types include:
- RF Coils: In MRI, radiofrequency coils are essential. They help to transmit and receive signals, playing a vital role in image quality.
- Piezoelectric Sensors: Utilized in acoustic scanning, these sensors convert mechanical stress into electrical signals. They are crucial for capturing high-fidelity acoustic information.
- Optical Sensors: Though less common in traditional resonance scanning, they are used in newer technologies that leverage optical coherence for imaging.
Selecting appropriate sensor technology can enhance the effectiveness of resonance scanning, making it essential to understand these tools as part of the overall system.
Control Algorithms
Control algorithms are the brain of the resonance scanner. They enable the machine to operate autonomously, processing real-time data and making adjustments as necessary for optimal performance. Important aspects include:
- Signal Processing: Algorithms process incoming signals to filter out noise and enhance the quality of the data collected. This step is crucial for obtaining clear images or accurate analyses.
- Image Reconstruction: In medical imaging, algorithms reconstruct the captured signals into visual representations, aiding medical professionals in diagnoses.
- Feedback Mechanism: Control algorithms often include feedback loops, adjusting parameters dynamically based on real-time measurements to maintain precision and accuracy.
The efficiency of resonance scanners depends significantly on how well these algorithms are designed and implemented.
Understanding these components helps to appreciate how resonance scanners work and why they hold importance in modern science and industry. They are more than just machines; they exemplify precision engineering combined with innovative technology.
Technology Behind Resonance Scanning
The field of resonance scanning encompasses technologies that revolutionize both diagnostic imaging and material analysis. Understanding the technology behind resonance scanning is crucial for grasping its multifaceted applications and benefits. Such technologies employ unique principles that allow practitioners to visualize internal structures and assess material properties with remarkable accuracy.
Magnetic Resonance Imaging (MRI)
Magnetic Resonance Imaging is one of the most recognized applications of resonance scanning. MRI operates based on the principles of nuclear magnetic resonance. It is a non-invasive imaging technique that uses strong magnetic fields and radio waves to generate detailed images of structures inside the body.
- Operational Mechanisms: When a patient is placed inside the MRI scanner, the magnetic field aligns the protons in hydrogen atoms. Radiofrequency pulses then disturb this alignment, causing the protons to emit signals as they return to their original state. These emitted signals are processed to create images.
- Clinical Significance: MRI has significant advantages in soft tissue imaging, such as the brain, muscles, and organs. It provides high-resolution images, making it invaluable in diagnosing conditions like tumors, brain disorders, and joint injuries.
- Limitations: Despite its benefits, MRI does have limitations. The procedure can be lengthy, and not all patients can undergo MRI due to conditions such as claustrophobia or metal implants. Furthermore, MRI machines are costly, which can lead to accessibility issues in some healthcare settings.
Acoustic Resonance Techniques
Acoustic resonance techniques are another set of technologies that utilize the principles of resonance in unique ways. These methods employ sound waves to analyze materials and detect structural variations at microscopic levels.
- How It Works: In acoustic resonance techniques, transducers emit ultrasound waves that interact with the material being tested. The response of these waves is measured, providing information about the internal structure, density, and composition of the material.
- Applications in Industry: Such techniques have applications in various industries, including construction, aerospace, and manufacturing. They are used for quality control, allowing for non-destructive testing of materials to ensure they meet safety and performance standards.
- Benefits and Challenges: Acoustic resonance is beneficial due to its non-invasive nature and ability to assess materials in real-time. However, it may face challenges such as the requirement for precise calibration and possible interference from environmental factors.
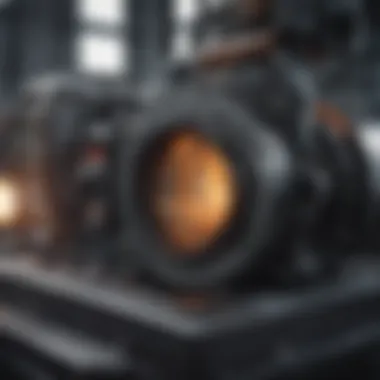
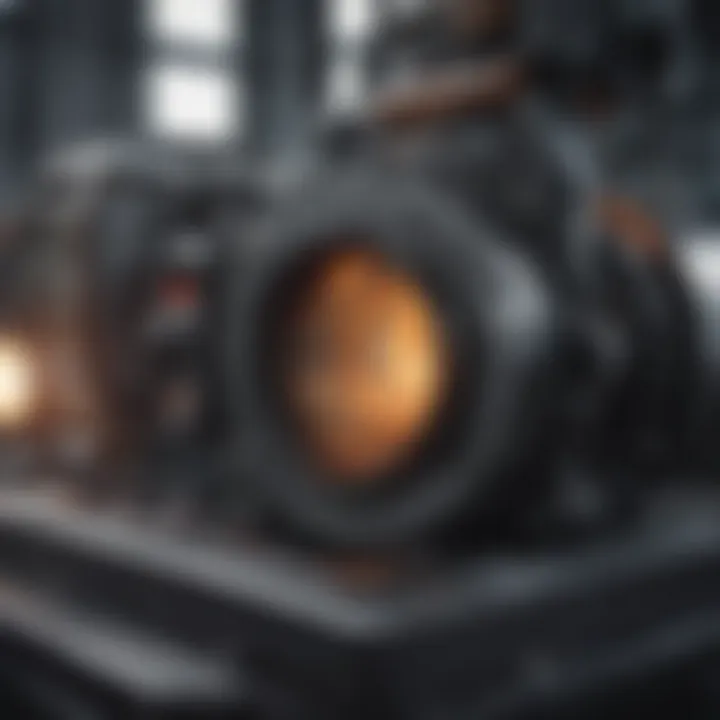
The integration of resonance scanning technologies like MRI and acoustic techniques reflects a significant step forward in both medical and industrial applications. Their ability to provide detailed imaging and analysis is unparalleled in many fields.
Applications of Resonance Scanners
The widespread applications of resonance scanners demonstrate their crucial role across various sectors, significantly in medical diagnostics and industrial uses. Each application showcases how resonance scanning technology facilitates detailed analysis, supporting critical decision-making processes. This section elaborates on the diverse applications and the intrinsic benefits and considerations tied to them.
Medical Diagnostics
Medical diagnostics utilizing resonance scanners represent a cornerstone of contemporary health care. These scanners provide non-invasive imaging techniques that yield high-resolution images, allowing for the precise identification of anomalies. Their use spans multiple domains, including oncology, neurology, and cardiology, largely benefiting patient outcomes by their ability to detect diseases at early stages.
Limitations and Challenges
Despite the advantages, resonance scans are not without limitations and challenges. An important aspect to consider is the cost of acquiring and maintaining the technology. Many healthcare facilities face significant financial implications, making it difficult to implement these systems universally. The limitations also extend to patient factors, such as claustrophobia and contraindications for individuals with certain implants.
Additionally, there are challenges associated with image interpretation. Radiologists must undergo rigorous training to understand intricate imaging results, and misinterpretation can lead to misdiagnosis. These factors collectively highlight why the exploration of limitations and challenges is critical for understanding resonance scanners in medical diagnostics.
Future Directions
Looking forward, the future directions of resonance scanning technology promise exciting advancements. One of the most significant developments is the integration of artificial intelligence to enhance image processing and analysis. By applying machine learning algorithms, radiologists can quickly identify and classify abnormalities, potentially reducing diagnosis time and increasing accuracy.
Moreover, efforts to improve the designs of resonance scanners to address patient comfort could help mitigate challenges such as claustrophobia. Continued research into contrast agents and methodologies will likely enhance imaging capabilities. These attributes collectively illustrate the promising future directions and their potential benefits in the context of enhancing medical diagnostic processes.
Industrial Uses
In the realm of industry, resonance scanners supply vital insights for quality assurance and materials characterization. Their applications help to ensure that products meet specifications and function reliably in their intended environments.
Quality Control
Within quality control processes, resonance scanners are invaluable tools for detecting imperfections or inconsistencies in materials. They often provide real-time feedback, allowing manufacturers to rectify issues before they become systemic. The key characteristic of using resonance scanners in this domain is their ability to perform thorough inspections without damaging the products. This non-destructive approach makes them a popular choice for industries where product integrity is paramount.
The benefits of utilizing resonance scanners for quality control include improved process efficiency and lower rejection rates of defective products. This effectiveness enhances overall operational productivity and profitability, making it an essential tool in manufacturing.
Materials Characterization
Materials characterization is another critical area where resonance scanners make a substantial impact. They provide detailed information about material properties and structures, which is essential for engineering applications. The unique feature of resonance scanning technology is its ability to analyze various materials at different scales, informing decisions on material selection and usage.
While the advantages of materials characterization include precise data that guides product development, the complexities involved in interpreting data can present challenges. Understanding the insights provided by resonance scanners requires expertise, underscoring the necessity for skilled personnel in analyzing results.
"Resonance scanners are redefining the standards of accuracy and efficiency in both medical and industrial fields, marking a significant evolution in diagnostic and analytical technology."
Advantages of Using Resonance Scanners
The increasing reliance on resonance scanners in both medical and industrial fields underscores their vital importance in advancing diagnostic technology. The advantages of these devices are notable, particularly in terms of their precision and non-invasive nature. Understanding these elements helps recognize the significant role resonance scanners play in the modern landscape of imaging and analysis.
Precision and Accuracy
One of the foremost advantages of resonance scanners is their ability to provide high precision and accuracy in measurements. These devices operate based on the principles of resonance frequency, which allows for detailed observation of sample properties. For instance, in Magnetic Resonance Imaging (MRI), the fine-tuned resonance frequencies help create detailed images of internal structures within the human body. This ability to achieve precise imaging is crucial for making accurate diagnoses.
Moreover, resonance scanners can detect minute variations in properties, leading to more reliable results. In industrial applications, such as quality control, the precision of these scanners ensures that materials meet stringent standards. This reliability significantly reduces risks associated with material defects and potential failures in products.
"Precision in resonance scanning leads to advancements in both healthcare and industrial safety."
Non-invasive Procedures
Another significant advantage of resonance scanners is their non-invasive nature. In medical settings, procedures that do not require incisions or insertion improve patient comfort and reduce recovery time. MRI, for instance, allows for comprehensive imaging of soft tissues without needing any form of surgical intervention. This approach minimizes the risks often associated with invasive diagnostic techniques.
In industrial contexts, non-invasive techniques allow for the characterization of materials without altering or damaging them. Non-destructive testing using acoustic resonance techniques exemplifies this. This method not only ensures integrity but also saves costs associated with sample destruction or extensive repairs.
The adoption of non-invasive procedures is progressively becoming a standard due to their benefits, enhancing both patient care and product assessment.
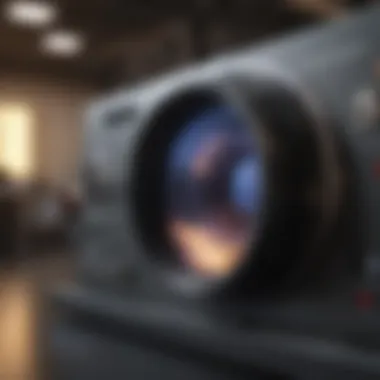
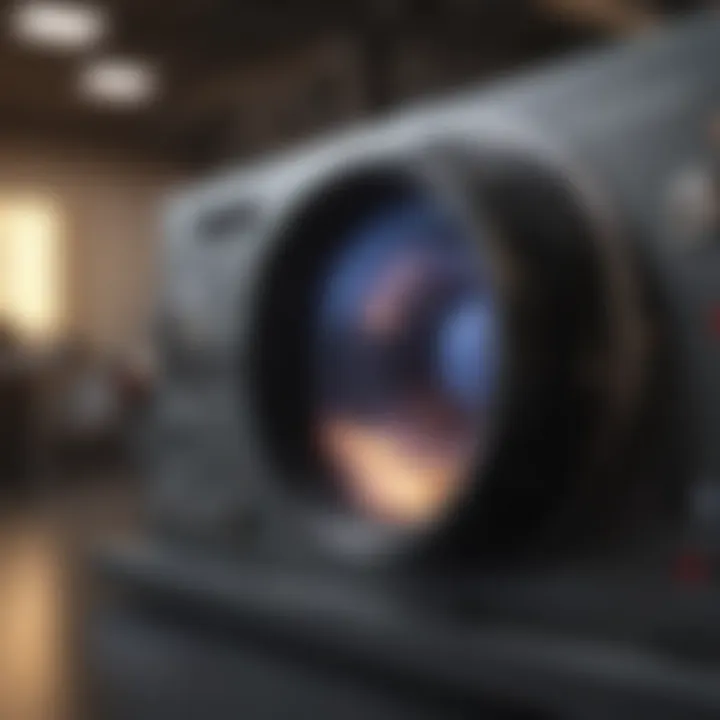
Through precision and non-invasive procedures, resonance scanners offer advantages that affect various sectors significantly. These aspects make them indispensable tools in advancing our understanding and capabilities in diagnostics and material analysis.
Challenges in Resonance Scanning Technology
The domain of resonance scanning technology presents numerous challenges that must be addressed for optimal utilization. While this technology has brought significant advancements in fields like medical diagnostics and industrial applications, it is essential to critically examine the limitations and implications surrounding its use. Understanding these challenges is crucial for researchers, practitioners, and stakeholders aiming to harness the full potential of resonance scanners.
Technical Limitations
One of the primary technical limitations in resonance scanning revolves around the sensitivity and resolution of the scanner. The effectiveness of these scanners largely depends on their ability to detect weak signals amidst noise. Factors like environmental disturbances can significantly affect the accuracy of readings. Furthermore, the complex algorithms required for interpreting resonance data often necessitate precise calibration and tuning.
Some resonance scanners, particularly Magnetic Resonance Imaging (MRI) devices, may require substantial tuning to achieve optimal performance. The physical limitations of the equipment, such as the size of the magnet or the coils used, can restrict the type of materials and biological tissues that can be analyzed. In certain cases, this confines the scanning capabilities to specific wavelengths, subsequently impacting the versatility of the technology across various applications.
Moreover, the time taken for scans can lead to patient discomfort in medical settings. Long scanning durations not only reduce patient compliance but also increase the chances of motion artifacts, further complicating data interpretation. Addressing these technical hurdles is paramount for improving the reliability and efficiency of resonance scanning technologies.
Cost Implications
The cost implications of resonance scanning technology represent another critical challenge. The initial investment for resonance scanners, especially advanced models like MRI machines, can be prohibitively high. Institutions are often required to secure substantial funding to cover equipment purchase, installation, and maintenance costs.
Beyond the purchase price, operational expenses can escalate based on the requirement for trained personnel and regular maintenance. Scanners typically require specialized technicians to ensure correct operation and interpret results accurately. This ongoing financial commitment can strain budgets, particularly for smaller healthcare facilities or research institutions.
Moreover, the complexity of resonance scanning can lead to unexpected expenses. Technical malfunctions may necessitate costly repairs or lead to long downtimes, further impacting service delivery. Therefore, careful cost analysis and budgeting become essential for any organization intending to implement resonance scanning technology.
Future of Resonance Scanning Technologies
The future of resonance scanning technologies is critical for multiple reasons. As industries, particularly healthcare and manufacturing, increasingly rely on precision and efficiency, advancements in resonance scanning become paramount. These technologies not only enhance the capabilities of existing systems but also enable entirely new applications, reflecting the evolving landscape of both science and industry.
Advancements in Sensor Technologies
Significant developments in sensor technologies are on the horizon. Innovations in materials science lead to sensors that can detect finer differences in resonance frequencies. For instance, the introduction of graphene-based sensors can potentially improve the sensitivity of resonance scanning, allowing for more accurate results in medical diagnostics.
Continued research in nanotechnology is also promising. Enhanced miniaturization of sensors may allow them to be integrated into portable devices. This integration will facilitate point-of-care testing, bringing sophisticated scanning capabilities to remote or underserved regions.
Key advancements include:
- Higher Sensitivity: Improved sensors will capture minute changes in resonance frequency, increasing diagnostic accuracy.
- Real-Time Data Processing: Faster processing will allow for almost instant results, crucial in emergency medical situations.
- Interoperability: New sensors will likely enhance compatibility with existing imaging systems, making it easier to incorporate them into current workflows.
Emerging Applications
The landscape of resonance scanning is set for transformation as new applications are discovered. One notable area is the potential integration with telemedicine. As healthcare systems adapt to a digital-first approach, remote diagnostics using resonance scanning could redefine patient engagement.
In industrial contexts, resonance scanners will play a role in predictive maintenance. By utilizing resonance frequency data, companies can monitor machinery health in real time and predict failures before they occur.
Emerging applications include:
- Telemedicine Integration: Remote resonance scanning can facilitate urgent care, improving access to healthcare.
- Predictive Maintenance: Industry can utilize resonance data to minimize equipment downtime and ensure operational efficiency.
- Material Inspection and Quality Control: Enhanced resonance technology can lead to better detection of flaws in materials, crucial for aerospace and construction industries.
The advancements in resonance scanning technologies are set to redefine precision in diagnostics and industrial applications, bringing a significant impact on how we approach health and safety.
Through continual evolution and research, resonance scanning stands poised to influence a plethora of fields, heightening efficiency and accuracy while potentially transforming practices across the board. The trajectory of this technology not only hints at improvements in performance but also a commitment to understanding complex systems and enhancing human capabilities.
Ending
In summary, resonance scanners embody a significant advancement in the realms of diagnostic imaging and industrial evaluation. This technology's ability to capture detailed information through resonance frequencies has transformed several fields. It not only provides a non-invasive method for medical diagnostics but also enhances quality control processes in manufacturing. The implications of these scanners extend far beyond mere imaging; they facilitate a deeper understanding of materials, biological systems, and various physical properties.
Summary of Key Points
- Definition and Mechanism: Resonance scanners function on the principle of resonance, allowing for the detailed scanning of both living tissues and materials. This technology hinges on the accurate measurement of frequencies that correspond to different substances.
- Applications: Major applications include Magnetic Resonance Imaging (MRI) in healthcare and advanced material analysis in various industries. The versatility highlights the scanners' potential in diverse environments.
- Advantages and Limitations: While the advantages, including precision and non-invasiveness, are significant, challenges exist such as technical limitations and cost implications which may hinder widespread adoption.
- Future Directions: Emerging developments in sensor technology indicate a robust future for resonance scanners. Ongoing innovations promise enhanced accuracy and new applications, which may redefine current methodologies.
The Impact of Resonance Scanners on Future Research
The evolution of resonance scanning technology stands to significantly influence future research across multiple disciplines. With continuous enhancements in sensor capabilities, researchers can expect to achieve even higher sensitivity and specificity in their measurements. This can lead to breakthroughs in medical diagnostics, such as earlier disease detection and more accurate imaging results. Moreover, in industrial contexts, improved resonance scanning methods can offer better insights into material properties, leading to innovation in product development.
Furthermore, interdisciplinary collaborations are likely to emerge, fostering advancements in both technology and application. As researchers explore novel uses of resonance scanning in areas such as nanotechnology and environmental science, the potential for transformative research is immense. The interplay of these developments not only promises to advance scientific inquiry but also to generate practical applications that can benefit society as a whole.
"The future of resonance scanners is not just about improvement in technology but also about expanding their application across varied fields, demonstrating their repertoire of capabilities."