Exploring the Structural Dynamics of Platelets
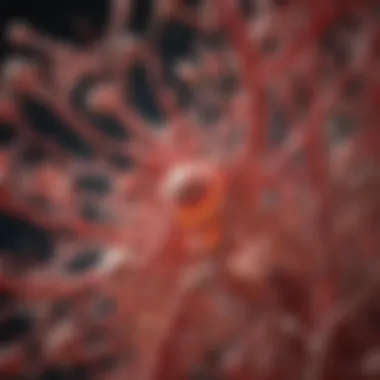
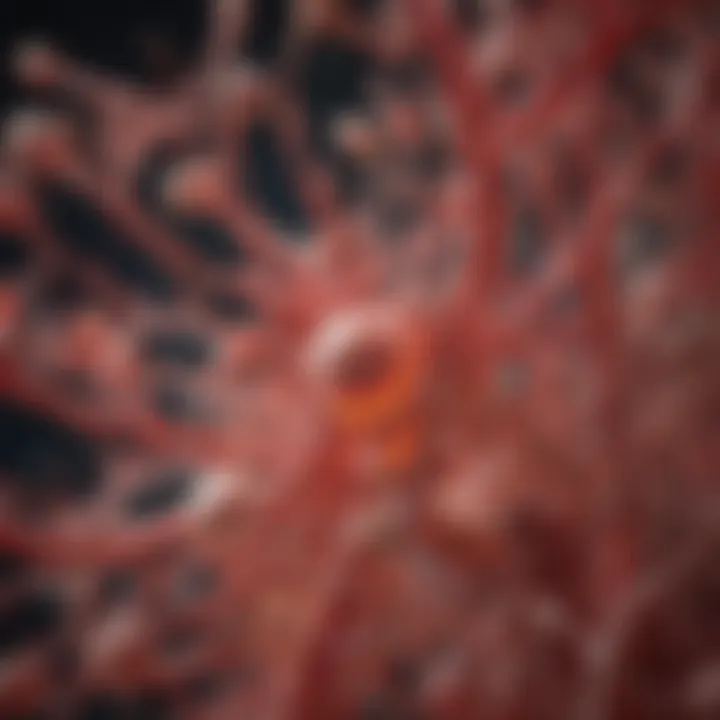
Intro
Platelets are a vital component of the hemostatic system. Despite their small size, they play a critical role in stopping bleeding and maintaining vascular integrity. Understanding their structure is essential for comprehending how they function. This examination allows for insights into the complexity of platelet dynamics and their involvement in various physiological processes.
The intricate architecture of platelets includes several organelles and cytoskeletal elements that contribute to their functionality. This dynamic structure not only supports their primary role in hemostasis but also informs us about the conditions under which they operate. By dissecting the various aspects of platelet composition and activation, this article aims to provide clarity on their role in vascular biology and pathology.
Furthermore, recent advancements in imaging techniques have enhanced our ability to study platelet morphology. This exploration into the microanatomy of platelets enriches our understanding of how structural changes can impact their functionality and may inform future therapeutic strategies.
Research Overview
Summary of key findings
This article explores the various elements that make up platelets, including:
- The origin and development of platelets from megakaryocytes.
- The composition of platelet granules and membranes.
- Cytoskeletal components that facilitate shape change and movement.
- Mechanisms that trigger platelet activation and aggregation.
By understanding these key areas, we can appreciate the complex relationships within platelets that enable their roles in hemostasis.
Importance of the research in its respective field
Methodology
Description of experimental or analytical methods used
- Electron Microscopy (EM): Provides high-resolution images of platelet ultrastructure.
- Fluorescence Microscopy: Allows visualization of specific proteins within platelets during activation.
Sampling criteria and data collection techniques
- Samples are typically derived from healthy volunteers, with strict adherence to ethical guidelines.
- Data from patients with known platelet disorders are also utilized to compare structural differences.
With these methods, the complexities of platelet structure and function can be examined comprehensively. By exploring the points of activation and the implications of dysfunction, the conversation around platelets can significantly advance.
"Understanding the fine structure of platelets reveals not just the mechanisms of hemostasis, but also potential therapeutic avenues for treating platelet-related disorders."
Intro to Platelet Structure
Understanding the structure of platelets is a significant aspect in the study of vascular biology and medicine. Platelets, or thrombocytes, are small, disc-shaped cell fragments crucial for blood coagulation and wound healing. Their structural components directly influence how they function. Insights into platelet structure provide a clearer view of their roles in hemostasis and their activation mechanisms. This section lays the groundwork for the subsequent discussions in the article, paving the way for a comprehensive comprehension of how platelets operate within the circulatory system and respond to injury.
Definition and Importance of Platelets
Platelets are an essential element in maintaining hemostasis—the process that prevents and stops bleeding. They originate from megakaryocytes in the bone marrow and are vital for blood clot formation. The normal platelet count ranges from 150,000 to 450,000 platelets per microliter of blood.
The importance of platelets cannot be overstated. They play a primary role in hemostatic control, responding rapidly to vascular injury. When a vessel is damaged, platelets adhere to the exposed site and aggregate to form a platelet plug. This reaction is the first step in the coagulation cascade, which ultimately leads to clot formation.
Additionally, platelets secrete various chemical signals that further modulate the healing response. This includes the release of growth factors that aid in tissue repair. Therefore, the structure and functionality of platelets are closely interconnected, making their study crucial for understanding both normal physiology and pathological conditions.
Overview of Hemostasis
Hemostasis is a complex process consisting of three main phases: vascular spasm, primary hemostasis, and secondary hemostasis.
- Vascular spasm occurs immediately after vascular injury, resulting in constriction of the blood vessel.
- Primary hemostasis involves the rapid accumulation of platelets at the injury site, where they adhere to exposed collagen and each other, forming a loose plug. This step also initiates the release of various signaling molecules.
- Secondary hemostasis stabilizes the temporary platelet plug through a cascade of coagulation factors that culminate in fibrin formation.
The interaction between platelets and these phases of hemostasis emphasizes their critical role in the prevention of excessive bleeding. Notably, abnormalities in platelet function or number can lead to conditions such as thrombocytopenia or thrombosis, reinforcing the importance of understanding platelet structure and function.
Platelet Origins and Development
Understanding the origins and development of platelets is crucial to grasp their full functional potential and roles in hemostasis. This aspect of platelet physiology emphasizes how they are formed, their lifecycle, and how these features contribute to vascular biology and medical applications. The knowledge of platelet development can inform clinical strategies for managing bleeding disorders and thrombosis, making this an important area of study for students, researchers, and professionals.
Thrombopoiesis and Megakaryocytes
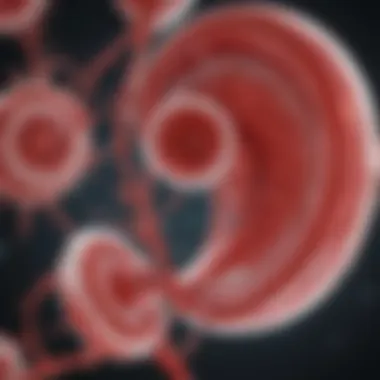
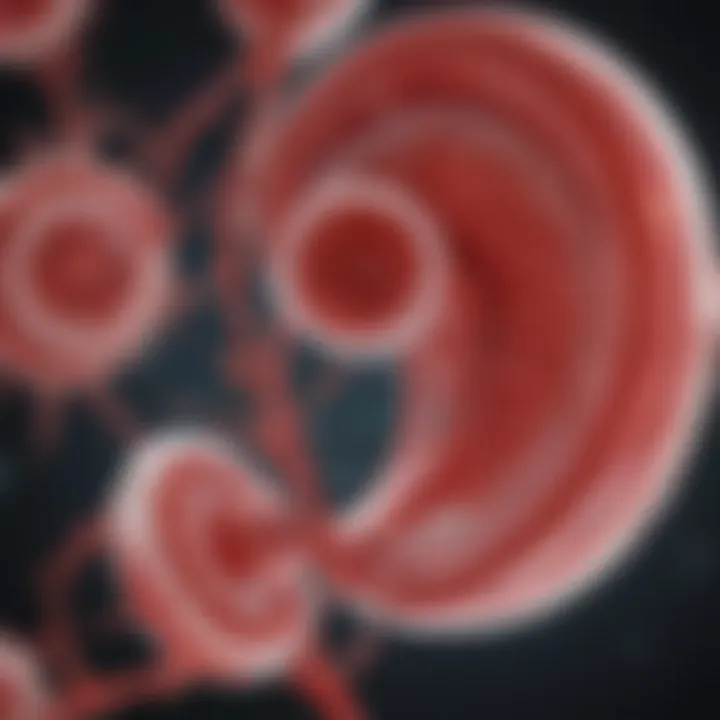
Platelets originate from specialized bone marrow cells known as megakaryocytes. This process, termed thrombopoiesis, involves the differentiation of hematopoietic stem cells in response to specific growth factors. One of the key factors involved is thrombopoietin, which stimulates the production and maturation of megakaryocytes.
Megakaryocytes are unique cells characterized by their large size and polyploidy. They can undergo a process called endomitosis, where the nucleus replicates without cell division, resulting in a multinucleated cell. This allows megakaryocytes to produce a significant number of platelets. The cytoplasmic projections of these megakaryocytes, known as pro-platelets, extend into the bloodstream, breaking off to form individual platelets.
The development of megakaryocytes is influenced by the microenvironment within the bone marrow, including interactions with stromal cells. Any dysfunction in this process may lead to either an insufficient supply of platelets or excessive production, both conditions being relevant in clinical contexts.
Lifecycle of a Platelet
Once formed, platelets have a relatively short lifespan, usually around 7 to 10 days. Their lifecycle can be divided into several distinct phases:
- Circulation: After being released from megakaryocytes, platelets enter the bloodstream, where they perform their key functions in hemostasis by monitoring vascular integrity.
- Activation and Aggregation: In response to vascular injury, platelets undergo a process of activation wherein they change shape and release granule contents. Factors such as collagen exposure or thrombin signaling can trigger these changes.
- Functionality: Activated platelets contribute to forming a platelet plug, a necessary step in stopping bleeding. They interact with other platelets and the vessel wall, reinforcing the aggregation through various receptors and signaling pathways.
- Clearance: After fulfilling their function, aged platelets are removed from circulation primarily by the spleen and liver. This clearance is vital for maintaining homeostasis and preventing the risks associated with excessive platelet accumulation, which could lead to clotting disorders.
The balance in the production and clearance of platelets is paramount. An imbalance might lead to either thrombocytopenia (low platelet count) or thrombocytosis (high platelet count), both harmful conditions.
Composition of Platelets
The composition of platelets is crucial for their functioning in hemostasis and overall vascular health. Understanding this composition offers important insights into how platelets respond to injuries within the body. Platelets, also known as thrombocytes, are not merely small cells; their structure houses various components that play distinct roles in their biological activities. The nuanced interactions among these elements determine the efficiency of the hemostatic process.
Cytoplasmic Components
The cytoplasm of a platelet contains various organelles and proteins essential for its function. One of the most significant constituents is the cytoskeleton, comprising actin filaments and microtubules. This structure provides shape and stability, allowing platelets to change their form during activation. Additionally, the cytoplasm contains granules that store vital proteins, such as clotting factors, which are released during the platelet activation process.
Importantly, the energy requirements of platelets necessitate the presence of mitochondria. While platelets have a limited lifespan, approximately 7-10 days, the rapid production of energy is sustained by mitochondria. This ensures that platelets can quickly respond to vascular injury by activating and aggregating.
Membrane Structure and Composition
The membrane of platelets is another fundamental aspect of their composition. It is primarily composed of a lipid bilayer that serves to encapsulate the cytoplasmic contents. This membrane is embedded with various glycoproteins and receptors that are essential for platelet adhesion and activation. For example, the glycoprotein IIb/IIIa complex binds to fibrinogen, facilitating platelet aggregation.
The lipid composition of the membrane also plays a role in cell signaling. Phospholipids, such as phosphatidylcholine and phosphatidylserine, are involved in signaling pathways that activate platelets upon vessel injury. Alterations in these lipid components can affect platelet reactivity, making the membrane and its composition a vital area of study in platelet research.
Granules and Their Contents
Platelets contain two main types of granules: alpha granules and dense granules. Alpha granules are larger and house a range of proteins, which include growth factors like platelet-derived growth factor (PDGF) and fibrinogen. These proteins are pivotal in the wound healing process and influence vascular repair.
Dense granules, on the other hand, are smaller and contain small molecules such as ADP, ATP, calcium ions, and serotonin. Upon activation, these substances are released into the surrounding area, promoting further platelet activation and aggregation. The coordinated release of these granule contents is essential for the amplification of hemostatic responses.
"The intricate composition of platelets enables them to play a multi-faceted role in hemostasis, influencing repair mechanisms and maintaining vascular integrity."
In summary, the composition of platelets, from their cytoplasmic components to their membrane structure and granule contents, underlines their complex functionality and importance in hemostasis. Understanding these elements facilitates further exploration into how they may be targetted in therapeutic strategies for disorders related to platelet function.
Cytoskeletal Architecture
The cytoskeletal architecture of platelets plays a crucial role in their functionality and response to environmental signals. This intricate network provides structural support, determines platelet shape, and facilitates essential processes such as activation and aggregation. Understanding the various components within the cytoskeleton helps to explain the dynamic nature of platelets and their pivotal role in hemostasis.
Role of Microtubules
Microtubules act like railways within the platelet's cytoskeleton. These tubular structures are composed of tubulin proteins, enabling them to provide rigidity and shape to the platelets. Their significance lies in several functional aspects:
- Shape maintenance: Microtubules help maintain the disc-like shape of resting platelets, which is essential for their proper functioning in血液的流動.
- Movement facilitation: During platelet activation, microtubules reorganize, allowing the platelets to change shape and extend pseudopodia, which are essential for interaction with other platelets and vessel walls.
- Activation support: Microtubules also play a role in the signaling pathways that trigger platelet activation, responding to stimuli such as collagen and thrombin.
As a result, alterations in microtubule dynamics can lead to impaired platelet function, affecting hemostatic responses.
Actin Filaments in Platelet Function
Actin filaments are perhaps the most critical elements of the platelet cytoskeleton. These filaments provide tensile strength and enable changeable morphology, which is vital during activation. The role of actin filaments can be summarized as follows:
- Shape remodeling: Upon activation, the polymerization of actin filaments leads to a rapid change in the platelet shape, transforming them from a resting state to a more active form suitable for aggregation.
- Granule secretion: Actin assists in the movement and secretion of granules filled with clotting factors, which are vital for promoting hemostasis.
- Integration with receptors: Actin interacts with integrin receptors on the platelet surface, facilitating effective adhesion to sites of vascular injury.
In summary, the cytoskeletal architecture, particularly the interplay of microtubules and actin filaments, ensures that platelets can respond swiftly and effectively during hemostatic events, highlighting the critical balance between structural integrity and dynamic function in these essential cells.
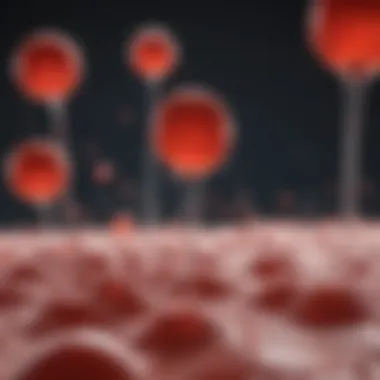
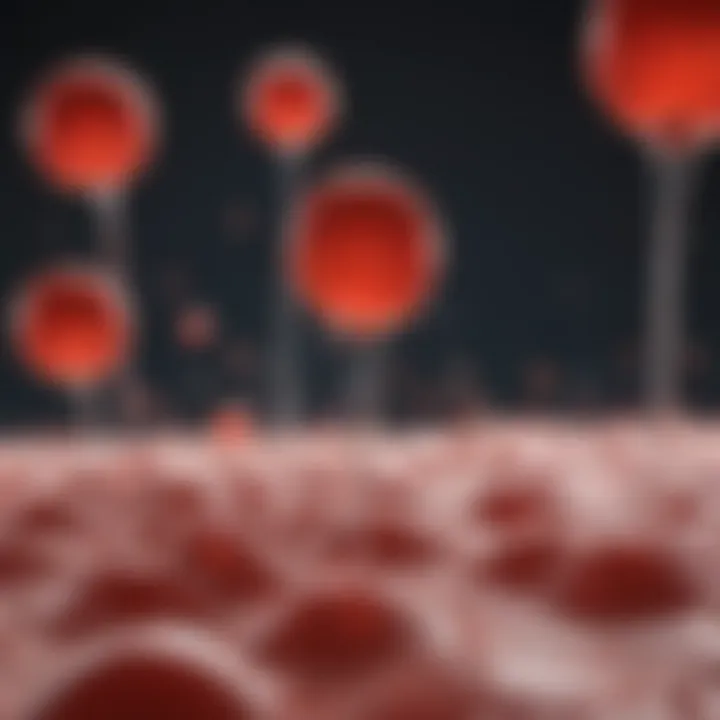
"The design of platelets allows them to be flexible yet strong, adapting to the ever-changing environment of the vascular system."
Understanding these elements is key for researchers and practitioners alike, as abnormalities in cytoskeletal components may underpin various platelet disorders.
Platelet Activation Mechanisms
Platelet activation is a crucial step in hemostasis, the process that prevents bleeding. This section details the triggers of platelet activation, the signaling pathways involved, and the consequent changes in platelet shape. Understanding these mechanisms shines light on how platelets respond rapidly to vascular injury, thereby underscoring their essential role in maintaining vascular integrity.
Triggers of Activation
Platelet activation begins with the recognition of traumatic events, primarily the rupture of blood vessels. Various triggers can initiate this process:
- Collagen: When collagen in the extracellular matrix is exposed due to injury, platelets adhere to it, starting the activation process.
- ADP (Adenosine Diphosphate): Released from damaged cells, ADP is a potent activator of platelets. It binds to specific receptors on platelets, amplifying the activation signal.
- Thrombin: Generated during the coagulation cascade, thrombin is a powerful platelet activator that not only promotes aggregation but also initiates further signaling pathways.
- Other stimuli: Numerous factors, like epinephrine and thromboxane A2, also play roles in platelet activation.
Recognizing these triggers is essential for understanding how platelets behave in both healthy and pathological conditions.
Signaling Pathways Involved
Once activated, platelets undergo a series of complex intracellular signaling events. Key pathways include:
- Integrin Activation: This process involves the activation of integrins such as αIIbβ3, leading to stable adhesion and aggregation.
- Calcium Mobilization: Activated platelets release calcium ions, which are crucial for various cellular functions, including shape change and aggregation.
- Phospholipase C Pathway: This pathway orchestrates diverse cellular responses leading to the production of inositol trisphosphate (IP3) and diacylglycerol (DAG), influencing platelet shape and granule release.
These signaling pathways illustrate the coordination of cellular events that occur rapidly during platelet activation, ultimately ensuring a swift response to injury.
Changes in Platelet Shape
Upon activation, platelets undergo notable morphological changes. This shape transformation is integral for their function. The main alterations include:
- Discoid to Sphere: Initially disc-shaped, platelets assume a spherical shape, providing more surface area for interaction with other platelets and the vascular wall.
- Filopodia Formation: Platelets extend pseudopodia, or filopodia, which enhances surface contact area. This extension fosters the aggregation of platelets at the injury site.
- Granule Exocytosis: Shape changes also trigger the release of granule contents, such as clotting factors. This further promotes aggregation and reinforces hemostatic function.
The shape changes of platelets serve as a fundamental aspect of their activation process, demonstrating the dynamism inherent in these cellular structures.
Understanding platelet activation is crucial for developing therapies targeting thrombotic conditions. The ability of platelets to rapidly change shape and aggregate is central to their function.
In summary, platelet activation mechanisms reveal the intricate processes that ensure effective hemostatic responses. By uncovering the triggers, pathways, and changes in shape, we gain insights into both normal physiology and disease states, emphasizing the vital role of platelets in health and medicine.
Platelet Aggregation Processes
Platelet aggregation is a critical phenomenon in hemostasis, where platelets clump together to form a plug that seals wounds in damaged blood vessels. This process is complex and involves various pathways and interactions. Understanding platelet aggregation processes is essential, as dysregulation in this mechanism can lead to both bleeding disorders and thrombotic diseases.
Mechanisms of Aggregation
Platelet aggregation occurs primarily through the interaction of specific receptors on the platelet membrane and soluble factors in the circulation. Key mechanisms include:
- Receptor Activation: The binding of agonists such as ADP, thrombin, and collagen to platelet receptors induces changes in the platelets. GPIIb/IIIa (an integrin) is particularly important as it undergoes a conformational change that allows binding between platelets.
- Cytoskeletal Rearrangement: Once activated, platelets undergo shape changes that contribute to aggregation. Actin filaments reorganize, resulting in the formation of pseudopodia, which extend and facilitate closer contact with other platelets.
- Release of Granule Contents: Activated platelets release contents from their granules including ADP and fibrinogen. These substances amplify the aggregation process and promote further platelet activation.
This aggregation process is a tightly regulated sequence of events. Disruption at any step can have considerable consequences, leading to insufficient clot formation or excessive clotting.
Implications of Aggregation in Hemostasis
The implications of platelet aggregation in hemostasis are profound. Successful aggregation is necessary for effective clot formation, which prevents excessive blood loss from injuries. Some important considerations include:
- Balance of Activation and Inhibition: Hemostasis relies on a delicate balance. While platelet aggregation is essential to stop bleeding, excessive aggregation can result in thrombosis, which may cause cardiovascular events like heart attacks or strokes.
- Role in Vascular Health: The ability of platelets to aggregate properly is also a reflection of vascular health. In pathological states, such as atherosclerosis, the composition of plaque can alter platelet behavior, leading to inappropriate aggregation responses.
- Therapeutic Targets: Understanding these processes allows for better therapeutic strategies. Drugs like aspirin or clopidogrel target specific pathways in platelet activation or aggregation, thus reducing the risk of thrombotic events.
"Platelet aggregation is not just a response to injury; it is a finely-tuned process essential for maintaining vascular integrity."
In summary, the study of platelet aggregation processes provides insights into how hemostasis is achieved and the potential for therapeutic interventions to manipulate these vital responses when necessary.
Pathological Aspects of Platelet Function
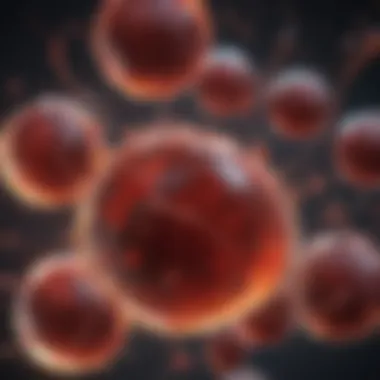
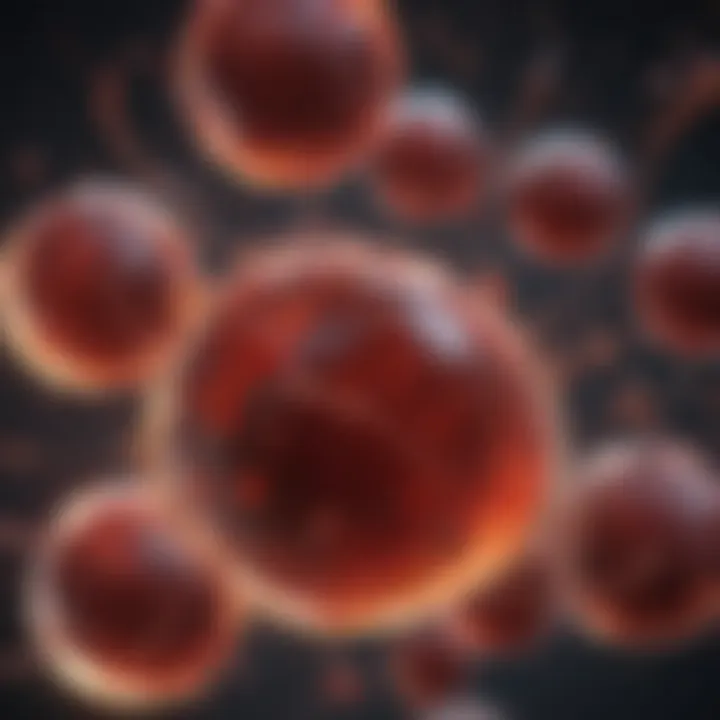
Understanding the pathological aspects of platelet function is crucial for grasping how these cells contribute to various diseases. Platelets are not just passive responders in the hemostatic process; they play active roles in several conditions, such as thrombosis and bleeding disorders. These conditions arise due to dysfunction or overactivity of platelets. Consequently, research in this area not only advances our knowledge of platelet biology but also informs therapeutic strategies.
Platelet Dysfunction and Diseases
Platelet dysfunction can manifest in several forms, leading to diseases characterized by abnormal bleeding. Common conditions associated with platelet dysfunction include:
- Bernard-Soulier Syndrome: This genetic disorder results from a deficiency in glycoprotein Ib, affecting the platelet's ability to adhere to the vessel wall.
- Glanzmann's Thrombasthenia: This condition arises from the absence of glycoprotein IIb/IIIa, which is essential for platelet aggregation. Patients experience prolonged bleeding due to impaired clot formation.
- Aspirin-Related Dysfunction: Long-term use of aspirin affects platelet function by inhibiting cyclooxygenase enzymes, which are necessary for thromboxane A2 synthesis. This can lead to an increased bleeding risk, particularly in surgical settings.
Platelet dysfunction can lead to potentially life-threatening conditions when left unaddressed, emphasizing the importance of early diagnosis.
The study of such diseases helps to delineate the mechanisms at play. Various tests can identify platelet function abnormalities, guiding clinicians in treatment choices. The integration of genetic testing is becoming more common, providing insight into the heritable nature of some platelet dysfunctions.
Impact of Platelet Hyperactivity
On the other end of the spectrum is platelet hyperactivity, which is implicated in thromboembolic disorders. Conditions such as myocardial infarction, stroke, and deep vein thrombosis often involve platelet hyperactivity, leading to excessive clotting. Factors contributing to this overactivity include:
- High Cholesterol: Elevated lipid levels can alter platelet function, making them more reactive.
- Diabetes: Increased glucose can enhance platelet activation, leading to a higher risk of cardiovascular events.
- Inflammation: Chronic inflammation can boost platelet activation through various signaling pathways.
Management of conditions related to platelet hyperactivity often involves antiplatelet medications such as clopidogrel or aspirin. These drugs inhibit specific pathways that drive platelet activation, reducing the likelihood of inappropriate clot formation. Understanding the biochemical underpinnings of platelet hyperactivity has led to advancements in targeted therapies, improving patient outcomes.
Advancements in Imaging Techniques
The importance of advanced imaging techniques lies in their ability to provide real-time analysis and multi-dimensional visualization of platelets. This leads to improved comprehension of their dynamics, interactions, and responses under various physiological conditions. Multiple methodologies exist, each offering a unique perspective and contributing to a holistic understanding of platelet behavior.
Fluorescent Microscopy Applications
Fluorescent microscopy has revolutionized our capability to visualize cellular components, including platelets. This technique leverages specific fluorescent dyes that bind to particular molecules or structures within platelets, enabling selective visualization. This approach enhances our understanding of platelet activation and the localization of functional proteins.
Key applications of fluorescent microscopy in platelet research include:
- Visualization of Activated Platelets: By using fluorescently labeled antibodies specific to activation markers, researchers can identify and quantify activated platelets in a sample.
- Tracking Platelet Interactions: Fluorescent dyes can be used to label platelets, allowing real-time tracking of their interactions with other cells or surfaces under flow conditions.
- Molecular Dynamics: Fluorescent techniques like Förster Resonance Energy Transfer (FRET) allow researchers to study the molecular dynamics within platelets, providing insights into signaling events during activation.
The advantages of these applications are profound. They provide a dynamic view of platelets in action, permitting the capture of critical moments during hemostatic processes.
Electron Microscopy Insights
Electron microscopy (EM) offers another level of detail that complements fluorescent microscopy. By using a beam of electrons, EM can achieve resolutions far greater than light microscopy. This quality makes it indispensable for studying the ultrastructure of platelets.
Two primary forms of electron microscopy are particularly useful in platelet study:
- Transmission Electron Microscopy (TEM): TEM provides detailed images of thin sections of platelets, allowing for the examination of organelles and cytoskeletal structures at nanometer resolution.
- Scanning Electron Microscopy (SEM): SEM offers three-dimensional images of platelet surfaces and can reveal morphological changes during activation and aggregation processes.
Applications of EM in platelet research include:
- Detailed Structural Analysis: Researchers can investigate the intricate details of platelet granules, membranes, and cytoskeletal arrangements.
- Characterizing Activation Changes: EM allows for the observation of morphological changes in platelets upon activation, providing insights into their functional state.
In summary, advancements in imaging techniques, including fluorescent and electron microscopy, have vastly improved our understanding of platelet structure and function. These tools enable researchers to explore the complex behavior of platelets, offering vital information that could impact clinical practices in vascular biology and medicine.
"An advanced understanding of platelet dynamics through imaging techniques is crucial for developing targeted therapeutic strategies in various diseases."
As research progresses, these innovative methodologies will continue to enhance our comprehension of the pivotal role platelets play in hemostasis and disease states.
Endings and Future Directions
The exploration of platelet structure and function is crucial in understanding various biological processes and potential medical issues. Conclusively, the insights shed light on the dynamic roles platelets play in hemostasis, as well as their potential implications in various diseases. Continued research on platelets ensures that we grasp their structural complexities and the factors influencing their behavior under physiological and pathological conditions.
"Advancements in our understanding of platelets can pave the way for innovations in treating vascular diseases."
Summary of Key Findings
- Platelet Structure: The architecture of platelets includes a well-organized cytoskeleton and various granules, which are essential for their functionality.
- Activation Mechanisms: Several mechanisms trigger platelet activation, including biochemical signals and mechanical forces.
- Aggregation Processes: The aggregation of platelets is a multi-step process significant for successful hemostasis.
- Pathological Insights: Platelet dysfunction is linked to numerous diseases, highlighting the need for comprehensive research on their behavior in altered states.
- Imaging Advancements: New imaging techniques provide deeper insights into platelet morphology, aiding in research and clinical applications.
Potential Areas for Research
Research continues to evolve, opening avenues worth investigating:
- Novel Imaging Techniques: Exploring new methods in fluorescence and electron microscopy can enhance our understanding of platelet dynamics.
- Genomic Studies: Investigating the genetic basis for platelet disorders can yield insights into personalized medicine approaches.
- Therapeutic Targeting: Identifying specific molecular targets within platelets could lead to innovative treatments for cardiovascular diseases.
- Interactions with Other Cells: Studying how platelets interact with endothelial cells and leukocytes may reveal important information about inflammation and thrombosis.
- Platelet Bioengineering: Advancements in bioprinting and synthetic biology can lead to the generation of engineered platelets for therapeutic applications.