Understanding Antibody Specificity in Immunology
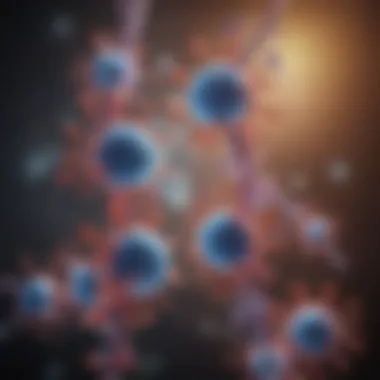
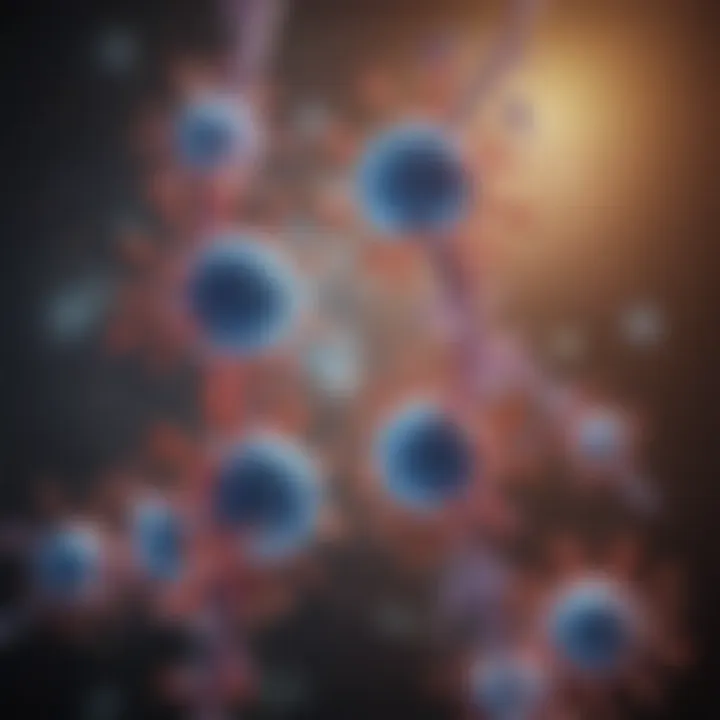
Intro
Antibody specificity is a cornerstone concept in immunology, reflecting the precise interactions between antibodies and their target antigens. Antibodies are proteins produced by the immune system to identify and neutralize foreign objects such as bacteria, viruses, and toxins. The specific binding affinity of an antibody for its antigen is fundamental to an effective immune response.
Understanding this specificity is crucial for developing vaccines and therapeutics. As we delve deeper into the mechanisms of antibody-antigen interactions, we uncover the intricacies of the immune response and its potential applications in medical science. This article aims to clarify the nature of antibody specificity and its importance in contemporary immunology.
Research Overview
Antibody specificity has been rigorously studied in recent years, leading to significant findings. The key takeaways from this research include:
- The structural diversity of antibodies and how it supports their binding capabilities.
- The role of epitope mapping in understanding how antibodies recognize specific antigens.
- The implications of antibody specificity in vaccine development and disease treatment.
The importance of this research extends beyond theoretical understanding. It influences practical applications in immunotherapy for cancers, autoimmune disorders, and infectious diseases. Advances in this field hold promise for improving patient outcomes and creating more effective treatment regimens.
Methodology
To understand antibody specificity, researchers often employ various experimental methods. Key methodologies include:
- X-ray crystallography: This technique helps visualize the structure of antibody-antigen complexes, revealing how antibodies bind to their targets.
- Enzyme-linked immunosorbent assay (ELISA): A common technique that quantifies the interaction between antibodies and antigens, providing insights into binding affinity and specificity.
- Surface plasmon resonance (SPR): This method measures real-time interactions between antibodies and antigens, allowing for the assessment of binding kinetics.
Sampling criteria typically involve the selection of antibodies with known specificity and the corresponding antigens. Data collection combines qualitative and quantitative approaches to generate a comprehensive view of antibody behavior.
Prelude to Antibody Specificity
Antibody specificity is a foundational concept in immunology, encompassing how the immune system identifies and responds to a myriad of antigens. Understanding this specificity is critical for developing therapeutic strategies, vaccines, and diagnostic tools. By delving into this topic, one can appreciate how antibodies interact with antigens at a molecular level, potentially leading to breakthroughs in disease prevention and treatment.
This section sets the stage for a rich exploration of antibody specificity, addressing various facets that will be covered in this article. We aim to discuss the intricate details of how antibodies are formed, how their specificity is determined, and why this specificity is vital for a functional immune response. Gaining insight into these elements benefits not only researchers but also healthcare professionals who rely on immunological principles to design effective interventions.
Definition of Antibody Specificity
Antibody specificity refers to the ability of an antibody to recognize a specific antigen or a closely related group of antigens. This means that each antibody has a unique structure that allows it to bind only to particular molecules, typically found on pathogens, such as viruses or bacteria. In practical terms, if an antibody is designed to target a specific virus, it will not effectively bind to another, unrelated virus. This precision is what makes antibodies technically powerful in therapeutic settings. Here, we can see the first glimpse of the complex relationship that dictates successful immune responses.
Historical Context
The study of antibody specificity has evolved significantly. Initial experiments date back to the late 19th century when Emil von Behring and Shibasaburo Kitasato discovered the effectiveness of serum from immunized animals in treating disease. This was the dawn of serology. As research progressed, Paul Ehrlich introduced the concept of the "magic bullet," positing that specific antibodies could target specific antigens, laying groundwork for modern immunology. Advances in technology, such as hybridoma techniques developed in the 1970s, have further advanced research, allowing scientists to create monoclonal antibodies with distinct specificities. These developments provide context for today's investigative research in antibody specificity.
Importance in Immunology
The importance of antibody specificity cannot be understated. It is essential for effective immune responses, which protect the body from pathogens while ensuring tolerance to self. Specificity directly contributes to the efficacy of vaccines. Vaccines train the immune system to recognize pathogens without causing disease, and this training hinges on the development of specific antibodies.
Moreover, specificity plays a crucial role in therapeutic applications. Monoclonal antibodies, for instance, are utilized in treatments for conditions like cancer, autoimmune diseases, and infectious diseases. Their effectiveness relies heavily on their capacity to bind precisely to targets, minimizing off-target effects and enhancing efficacy. In essence, understanding antibody specificity aids both fundamental research and clinical applications, emphasizing its integral role in immunology.
Mechanisms Behind Antibody Specificity
Antibody specificity is fundamental to the immune system's ability to identify and neutralize pathogens. Understanding the mechanisms behind this specificity enhances our insights into immunity, disease resistance, and the development of antibody-based therapies. There are several key components that explain how antibodies recognize specific antigens and maintain their effectiveness in immune responses.
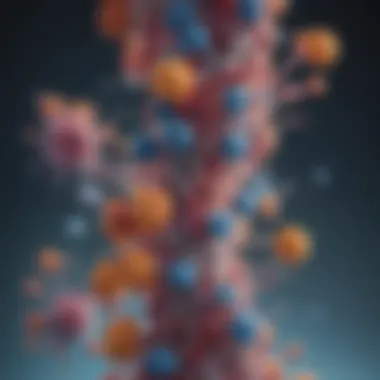
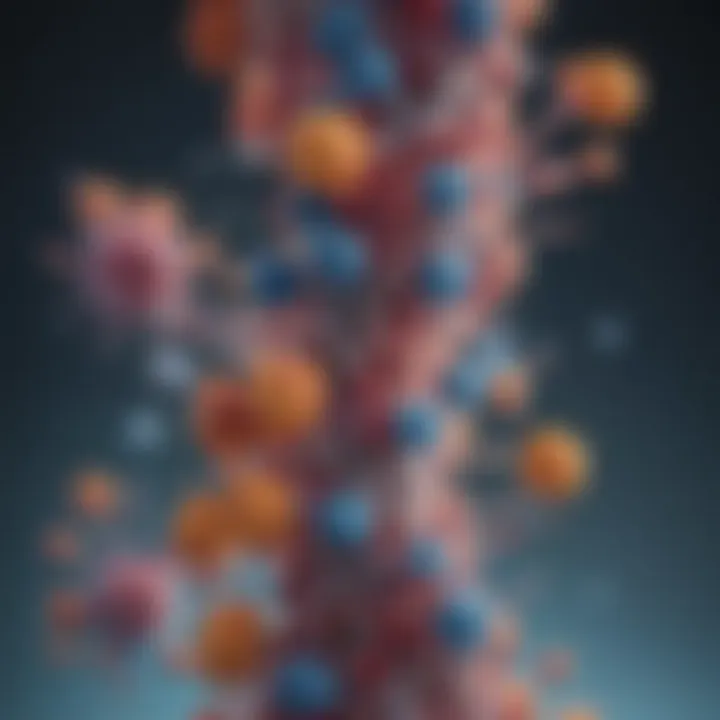
Molecular Structure of Antibodies
Antibodies, or immunoglobulins, are complex proteins with a distinct Y-shaped structure. This structure consists of two heavy chains and two light chains. Each antibody has a variable region and a constant region. The variable region is crucial for binding to antigens, while the constant region determines the antibody's class and its role in immune response. The specificity of an antibody is dictated by its unique variable region.
The variable region contains the antigen-binding sites that allow antibodies to latch onto specific epitopes on antigens. This specific binding capability is due to the precise arrangement of amino acids within the region, which creates a unique three-dimensional structure. Thus, the structural characteristics and variations in the molecular structure are essential for antibody specificity.
Antigen Recognition
Antigen recognition is a critical process that involves the binding of antibodies to specific antigens. This recognition is primarily mediated through non-covalent interactions, including hydrogen bonds, ionic bonds, and hydrophobic interactions. Each antibody can recognize a unique epitope, which is a specific part of the antigen.
During the immune response, B cells produce antibodies that display high specificity towards a distinct epitope. The binding of an antibody to an antigen triggers various immune mechanisms that lead to the neutralization or destruction of the pathogen. Because of this highly selective interaction, understanding how antibodies recognize antigens is vital for vaccine design and immunotherapy.
Clonal Selection Theory
Clonal selection theory explains how B cells gain specificity for diverse antigens. This theory proposes that each B cell has receptors that can bind to specific antigens. When a B cell encounters its corresponding antigen, it binds and gets activated. This activation results in the proliferation of that particular B cell, leading to the production of B cell clones that produce identical antibodies.
The clonal selection process ensures that the immune system generates a targeted response to pathogens effectively. Moreover, memory B cells are formed during this process, which ensures long-term immunity and a rapid response to subsequent exposures to the same antigen. The implications of clonal selection theory are profound, influencing the approaches taken in developing vaccines and antibody therapies.
Factors Influencing Antibody Specificity
Understanding the factors that influence antibody specificity is essential in immunology. These factors determine how effectively antibodies can recognize and react with different antigens. Moreover, they play a crucial role in vaccine development and therapeutic applications. The interplay of genetic information and environmental influences greatly affects antibody production. The following sections will delve deeper into these key aspects.
Genetic Diversity and Somatic Hypermutation
Genetic diversity is a fundamental element in the immune response. It allows the body to produce a wide array of antibodies that can recognize various pathogens. B cells, a type of white blood cell, are responsible for producing antibodies. These cells undergo a process called somatic hypermutation. During this process, the genes encoding the antibody are altered, resulting in a diverse population of antibodies.
Somatic hypermutation occurs primarily in the germinal centers of lymphoid tissues. Here, B cells experience rapid division and mutation, leading to variations in their antibodies. Some of these mutations enhance the binding affinity of antibodies to antigens, while others may be detrimental. The B cells that produce the most effective antibodies are selected for proliferation, enabling a robust immune response.
Affinity Maturation
Affinity maturation is the process by which antibodies become more effective at binding to their specific antigens. This occurs after the initial exposure to an antigen, and it is a vital aspect of the adaptive immune response.
In affinity maturation, B cells that produce antibodies with higher affinity for an antigen will survive and expand. The process continues as these B cells mutate further, and the best candidates with the highest affinity thrive. This leads to a population of antibodies that are finely tuned for their target, thus enhancing the overall effectiveness of the immune response.
The significance of affinity maturation cannot be overstated. It ensures that the immune system can respond more effectively upon re-exposure to the same pathogen. In the context of vaccine development, understanding how to promote this process is key to creating more effective vaccines.
Epitope Mapping and Analysis
Epitope mapping is the technique used to identify which parts of an antigen are recognized by antibodies. Understanding epitopes is crucial for developing vaccines and therapeutics. Epitopes can be linear or conformational, and identifying them aids in predicting how antibodies will interact with pathogens.
Through various advanced techniques like X-ray crystallography, mass spectrometry, and ELISA, researchers can analyze the specific interactions between antibodies and their cognate epitopes. This information helps in designing more precise therapeutics and improving vaccine strategies. By knowing which epitopes elicit the strongest and most protective antibody responses, researchers can create targeted interventions that enhance immunogenicity.
Mapping the epitope can significantly enhance the design of vaccines and therapeutic antibodies, leading to more effective treatments.
Applications of Antibody Specificity
The applications of antibody specificity are critical to multiple domains within immunology and biotechnology. The ability to precisely target antigens is fundamental not only for therapeutic interventions but also for preventative measures such as vaccines. This specificity ensures that antibodies can be engineered to treat various diseases, facilitate effective vaccination strategies, and serve as reliable diagnostic tools. By accentuating these applications, one can grasp their far-reaching benefits across healthcare.
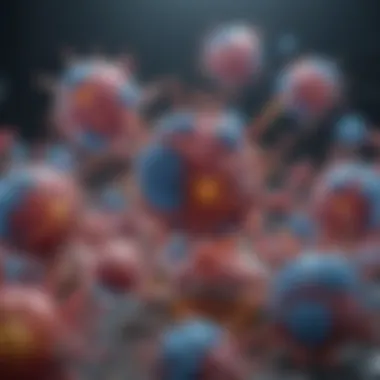
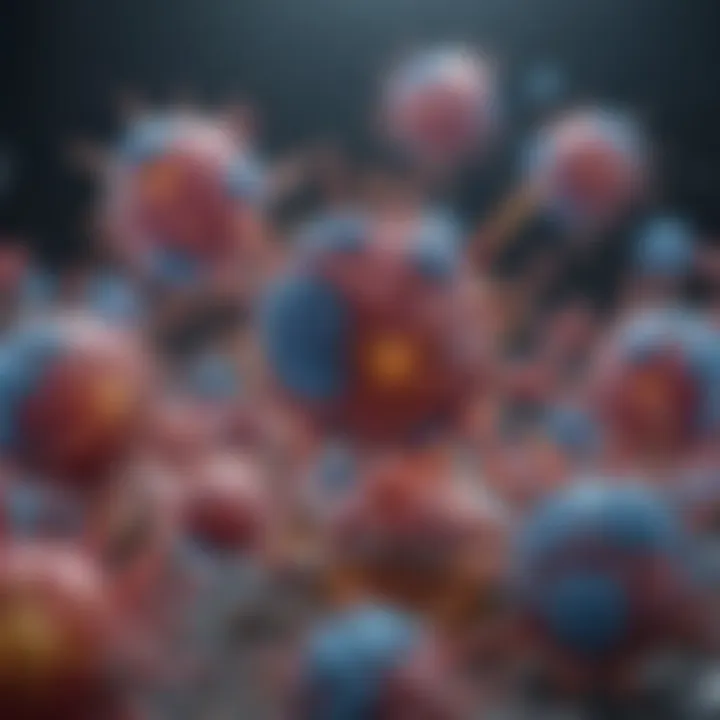
Therapeutic Antibodies
Therapeutic antibodies have revolutionized the treatment landscape for numerous conditions, including cancer, autoimmune disorders, and infectious diseases. These antibodies act by specifically binding to target antigens on the surface of cells, enhancing the immune response or blocking disease progression.
- Monoclonal Antibodies (mAbs): These are identical antibodies cloned from a single parent cell. Their specificity allows them to target only the intended antigen, minimizing impact on healthy cells. Examples include Rituximab for lymphoma and Trastuzumab for breast cancer.
- Mechanisms of Action: Therapeutic antibodies can neutralize toxins, activate immune pathways, or deliver cytotoxic agents directly to target cells. This targeted approach often leads to fewer side effects compared to traditional therapies.
- Emerging Therapies: The field of therapeutic antibodies is expanding, with advancements such as bispecific antibodies that can bind to two different antigens. This innovation holds potential for treating complex diseases where multiple pathways are involved.
Vaccination Strategies
Vaccination fundamentally relies on the concept of antibody specificity. The aim is to elicit an immune response that generates long-lasting resistance against specific pathogens. Through careful design, vaccines use either inactivated pathogens, live attenuated strains, or subunit components to create a tailored immune response.
- Mechanisms of Vaccination: Upon administration, antigens stimulate the immune system, leading to the production of specific antibodies. These antibodies remain in the system, providing immunity upon future exposures.
- Current Practices: Vaccines like the Influenza Vaccine and the COVID-19 Vaccines utilize engineered antibodies that target specific viral proteins. The clarity with which these antibodies can identify pathogens is central to vaccine success, impacting their efficacy and safety.
- Future Vaccination Approaches: Next-generation vaccines are focusing on broader specificity, enabling them to provide protection against varied strains of viruses, as seen with mRNA vaccine technology. Such innovations highlight the role of antibody specificity in developing robust vaccination strategies.
Diagnostic Tools
Specificity in antibodies extends to diagnostic applications, where they are employed to detect the presence of pathogens or biomarkers associated with diseases. Accurate diagnostics are vital for effective patient care and disease management.
- Enzyme-Linked Immunosorbent Assay (ELISA): This test employs antibodies to detect antigens in samples. Its specificity allows for reliable detection of diseases such as HIV and various allergens.
- Immunohistochemistry: This technique uses antibodies that bind to specific proteins in tissue sections. It assists pathologists in accurately identifying disease processes, especially cancers.
- Point-of-Care Testing: Rapid tests, particularly in infectious disease management, rely on antibody specificity for quick diagnosis. Tests for conditions like Malaria or COVID-19 use highly specific antibodies to deliver fast results.
"The precision with which antibodies can interact with their targets explains their vast applications in therapy, vaccination, and diagnostics."
In sum, understanding the applications of antibody specificity is essential for advancing medical science. Therapies, vaccines, and diagnostics all benefit from tailored antibody interactions. As research continues to progress, these applications will likely evolve to address new health challenges.
Challenges in Understanding Antibody Specificity
Antibody specificity is a pivotal concept in immunology. However, understanding this specificity is not without its challenges. Various intricacies affect how antibodies react with antigens. Addressing these challenges is crucial for advancing research and therapeutic applications.
Cross-Reactivity Issues
Cross-reactivity occurs when an antibody binds to multiple antigens, not just the one it was designed to target. This can lead to false positives in diagnostic tests and reduced effectiveness in therapeutic applications. Several factors contribute to cross-reactivity. For example, structural similarities between different antigens can confuse the immune system. Consequently, understanding these issues can help in developing more precise antibodies.
Research in this area is aimed at identifying how and why cross-reactivity occurs. It is essential to focus on how different protein structures can lead to varying degrees of antibody binding. This knowledge may lead to improved antibody design, with fewer side effects or unintended actions.
Antibody Engineering Complexity
Engineering specific antibodies for targeted therapies presents its own set of challenges. The process involves modifying antibody structures to enhance their specificity for a chosen antigen. This process is complex because the immune system is highly adaptable and can alter its responses based on changing conditions.
Designing antibodies that maintain high specificity requires intricate understanding of both antigens and antibodies. Additionally, the engineering process must consider factors such as stability, affinity, and bioavailability. The multifaceted nature of antibody engineering means that collaboration across disciplines is often necessary.
Challenges exist in assuring that engineered antibodies do not lose their affinity or become susceptible to rapid degradation. This is important because it can severely impact the efficacy of treatments based on monoclonal antibodies.
As researchers navigate these challenges, they contribute to a growing body of knowledge that holds promise for future advances in treatments and diagnostics.
In summary, while many challenges arise in understanding antibody specificity, addressing issues like cross-reactivity and the complexities of antibody engineering is essential. Such understanding can lead to remarkable innovations in immunotherapy and diagnostic methods.
Recent Advances in Antibody Research
Recent advances in antibody research have transformed our understanding of the immune system. These developments significantly enhance our ability to create targeted therapies and effective vaccines. In this section, we will delve into two pivotal facets of this progress: new therapeutic innovations and next-generation sequencing technologies.
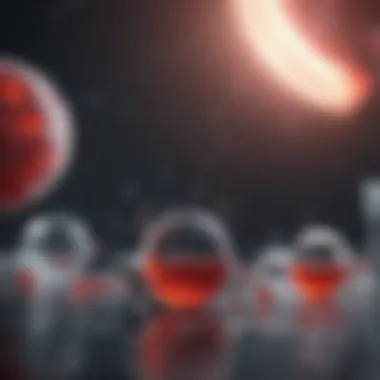
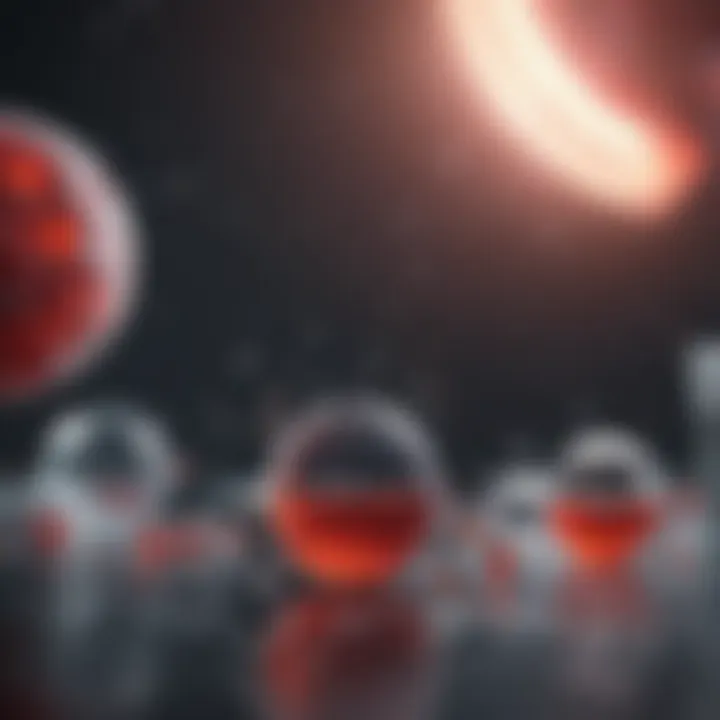
New Therapeutic Innovations
New therapeutic innovations often focus on harnessing the unique specificity of antibodies. A key area is the development of monoclonal antibodies. These are engineered to bind to specific antigens. For instance, Trastuzumab is an antibody that targets the HER2 receptor in breast cancer cells, significantly improving treatment outcomes.
The benefit of such innovations is substantial, as they can lead to precision medicine approaches. Techniques like antibody-drug conjugates combine antibodies with cytotoxic drugs to selectively kill cancer cells while minimizing damage to healthy tissues. This precision reduces side effects and enhances efficacy.
In addition to oncology, therapeutic antibodies also address autoimmune diseases. Adalimumab, for example, targets tumor necrosis factor (TNF), providing relief in conditions such as rheumatoid arthritis. The tailored immune response helps manage chronic diseases effectively.
Next-Generation Sequencing Technologies
The introduction of next-generation sequencing (NGS) technologies has opened new avenues for antibody research. NGS allows for rapid and comprehensive analysis of the antibody repertoire. Researchers can now sequence heavy and light chains of antibodies in high throughput, providing insights into diversity and specificity.
One of the significant advantages of NGS is its ability to identify rare antibody species. This is crucial for discovering new therapeutic antibodies that might not be found through traditional methods. The data generated can lead to the development of pooled antibody libraries, which enhance the screening process for high-affinity antibodies.
Moreover, NGS contributes to understanding the adaptive immune response. By comparing antibody sequences before and after immunization, researchers can track somatic hypermutation and clonal expansion. This understanding is vital in designing more effective vaccines.
"Advancements in antibody research are not just theoretical; they are shaping how we approach treatment and prevention in modern medicine."
In summary, recent advances in antibody research highlight the significance of continued explorations in both new therapeutic innovations and the application of cutting-edge sequencing technologies. Each development not only expands our knowledge but also drives practical applications that improve patient outcomes across various medical fields.
Future Directions in Antibody Specificity Studies
The landscape of antibody specificity research is poised for significant change and improvement. As researchers and scientists explore this area, new avenues of inquiry emerge. These directions are important, as they could enhance our understanding and applications of antibodies within immunology. The future promises to integrate more advanced methodologies, leading to refinement in therapeutic and diagnostic practices.
Potential Research Areas
There are several potential research areas which could yield valuable insights into antibody specificity:
- Understanding Rare Antibodies: Investigating how rare antibodies generate specificity can lead to breakthroughs in therapies for rare diseases.
- Exploring Multifunctional Antibodies: As antibodies can assume multifunctional roles, research into their versatility may provide insights into novel therapeutic applications.
- Novel Antigen-Antibody Interactions: Clarifying interactions beyond traditional models may reveal new pathways for vaccine development.
- Longitudinal Studies: Following antibody responses over time can help in understanding the long-term specificity of antibodies, especially in vaccination studies.
These research areas will add depth and breadth to our current knowledge and enhance the relevance of antibody specificity in real-world scenarios.
Integration of AI and Computational Models
The integration of artificial intelligence and computational models into antibody research cannot be overstated. Such technological advancements can transform how researchers analyze data and predict outcomes. Here are key considerations for this integration:
- Data Analysis: AI can process vast datasets more efficiently than traditional methods. This can lead to quicker insights into antibody-antigen interactions.
- Predictive Models: Leveraging machine learning algorithms can enable scientists to predict antibody behavior and specificity before conducting expensive laboratory tests.
- Simulations: Computational modeling can simulate antibody responses, providing researchers with data that is not always easy to obtain experimentally.
- Optimization of Therapeutics: AI can assist in refining antibody designs, optimizing their effectiveness in targeting specific antigens, which enhances therapeutic outcomes.
By marrying biology with computational power, researchers can unlock new possibilities in understanding and employing antibody specificity effectively. The future appears bright for this field as it adapts to new tools and technologies.
Ending
The conclusion serves as a vital component in any scholarly article. It encapsulates the core ideas presented and reinforces the significance of antibody specificity in immunology. In this article, we have journeyed through the complexities of antibody specificity, revealing how it shapes both therapeutic strategies and vaccine innovations.
Summary of Key Points
- Definition and Importance: Antibody specificity is fundamental to how the immune system identifies and neutralizes pathogens. It allows the differentiation between self and non-self, ensuring that only harmful entities are targeted.
- Mechanisms: We explored the molecular structure of antibodies and their interaction with antigens. Clonal selection theory and affinity maturation were discussed as crucial processes that enhance the responsiveness of the immune system.
- Applications: The use of therapeutic antibodies has fueled advancements in treating diseases such as cancer and autoimmune disorders. Additionally, vaccine development relies heavily on our understanding of antibody specificity, enhancing their effectiveness.
- Challenges and Recent Advances: Issues surrounding cross-reactivity pose significant challenges in antibody applications. However, recent innovations, including next-generation sequencing technologies, facilitate deeper insights into these complex interactions.
- Future Directions: As research progresses, the integration of artificial intelligence and computational models is expected to revolutionize antibody specificity studies, potentially unlocking new therapeutic avenues.
Significance of Ongoing Research
Ongoing research in antibody specificity is critical. It not only broadens the understanding of immune responses but also holds the potential to influence clinical practices significantly. Identifying novel antibodies through advanced techniques can lead to innovative treatments, transforming patient outcomes in various medical fields.
Moreover, exploring the subtleties of antibody interactions can pave the way for more effective vaccines, particularly in the context of emergent infectious diseases. As global health challenges persist, the drive to enhance antibody specificity through research will be paramount. By harnessing these insights, the scientific community aims to push the boundaries of immunology, leading to breakthroughs that could benefit public health on a large scale.