Understanding Biofilm Bacteria: Complexities and Implications
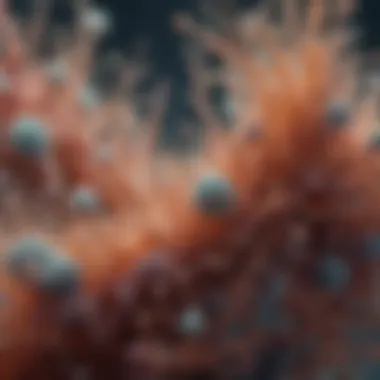
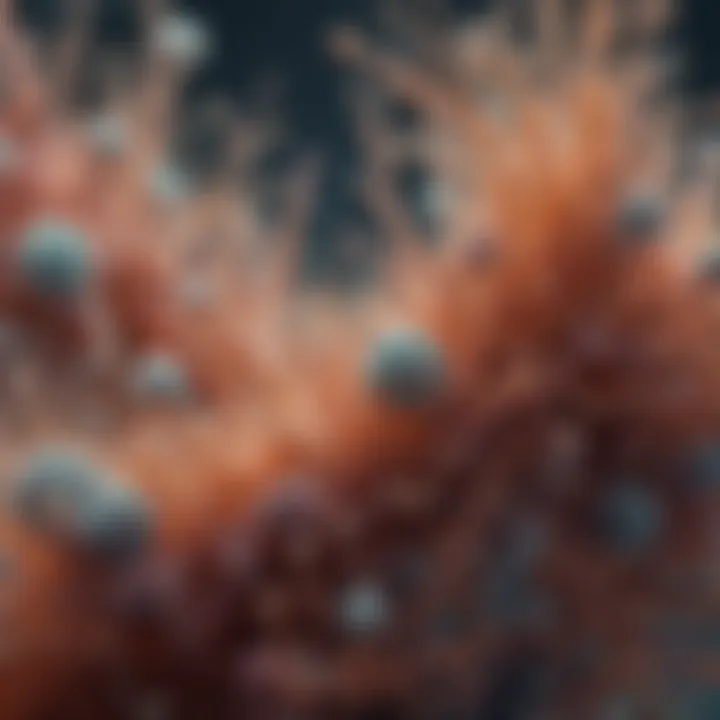
Intro
Biofilms are complex communities of microorganisms adhering to surfaces, surrounded by a self-produced protective layer of extracellular polymeric substances. These structures exhibit fascinating characteristics that have significant implications in numerous fields. Understanding biofilms is vital as they play crucial roles in diverse environments ranging from natural ecosystems to engineered settings.
Research into biofilms not only reveals intricacies of microbial life but also highlights their impact on human health. They can be found in places such as dental plaque, wastewater treatment facilities, and even in industrial pipelines. The dual nature of biofilms, both beneficial and detrimental, necessitates a thorough exploration.
This article aims to dissect the complexities and implications of biofilm bacteria, providing a detailed analysis of their structure, ecological significance, health impacts, and methods for their detection and eradication.
Prelims to Biofilm Bacteria
The examination of biofilm bacteria is crucial in several fields, including microbiology, medicine, and environmental science. Biofilms present unique challenges that affect both human health and industrial processes. Understanding the complexities of these microbial communities helps us to identify their role in chronic infections and their environmental impact.
Biofilms are structured assemblages of bacteria that adhere to surfaces and each other, creating a protective environment. This characteristic allows them to thrive in diverse settings—from natural ecosystems to man-made structures. The implications of biofilm presence stretch across public health, as pathogenic biofilms are often associated with persistent infections. They also influence biotechnological advancements, impacting everything from wastewater treatment to the development of novel materials.
Definition of Biofilm
A biofilm is defined as a complex community of microorganisms that attach to a surface, forming a multicellular aggregate. This attachment is facilitated by a slimy layer of extracellular polymeric substances (EPS), which is secreted by the bacteria within the biofilm. The EPS provides structural integrity and serves as a barrier against environmental stressors, including antimicrobial agents.
Biofilms can develop on various surfaces, including natural environments like riverbeds and man-made surfaces like medical devices. The formation process begins when free-floating bacteria settle on a surface, followed by colonization and maturation into a dense community. Over time, biofilms can change dynamically, with bacteria undergoing gene expression changes that affect their behavior and characteristics, presenting a formidable challenge for treatment.
Historical Context
The understanding of biofilms has evolved significantly over time. Historically, microbiologists studied bacteria in suspension, often overlooking those capable of forming biofilms. This oversight continued until the importance of biofilms became apparent in the late 20th century. Research began to reveal that a significant number of infections—chronic and acute—were associated with biofilm communities.
Research by pioneers like Robert Costerton in the 1980s was instrumental in changing perceptions about these microbial structures. He highlighted that biofilms could resist antibiotics, making them a considerable threat in clinical settings. As awareness increased, biofilms were no longer regarded merely as a nuisance in medical applications but as critical players in infection dynamics and chronic disease.
The recognition of biofilms has since led to increased research efforts. We now have a deeper understanding of the biochemical and ecological factors governing biofilm formation, the diversity of microbial species involved, and their adaptive mechanisms. More recently, advances in imaging and molecular biology have facilitated a more detailed examination of biofilms, laying the groundwork for innovative solutions to mitigate their adverse effects on health and industry.
"The formation of biofilms is not just a microbial survival strategy but an ecological event with profound implications for both natural environments and human health."
The study of biofilms continues to be a rapidly growing field. Insights into their biology and ecology are vital for developing strategies for detection, prevention, and eradication in both healthcare and environmental settings.
Biological Composition of Biofilms
The biological composition of biofilms is a foundational aspect of understanding these complex microbial structures. Biofilms are not merely clusters of bacteria; they are intricate communities comprised of diverse microbial populations. Each component contributes to the overall functionality and resilience of the biofilm, making this topic essential for research and practical applications in fields such as medicine, environmental science, and biotechnology.
Microbial Communities
Microbial communities within biofilms consist of various species, including bacteria, fungi, and protozoa. These communities interact symbiotically, leading to enhanced growth and survival in adverse conditions. Each species often has a specific role and offers distinct advantages. For instance, some bacteria may degrade pollutants while others provide structural support or help in nutrient cycling. Certainly, the diversity in microbial species enhances the adaptability of biofilms to their environment. This can result in their proliferation in both natural and engineered systems. Understanding these communities is crucial, especially regarding how they respond to treatment interventions and environmental changes.
Extracellular Polymeric Substances (EPS)
Extracellular polymeric substances (EPS) are a vital component of biofilm structure. These substances, mainly composed of polysaccharides, proteins, and nucleic acids, contribute to the physical integrity of the biofilm. EPS acts as a protective barrier, safeguarding microbial cells from environmental stresses such as UV radiation and chemical antibiotics. Furthermore, EPS plays a significant role in biofilm development, stability, and nutrient exchange within the microenvironment. The self-produced nature of EPS allows microorganisms to maintain a stable community while enhancing their resilience against hostile conditions.
Research indicates that manipulating EPS production could lead to improved strategies for biofilm control. Understanding the synthesis and function of EPS offers insights into developing more effective antimicrobial treatments.
Cell-Cell Interactions
Cell-cell interactions in biofilms are multifaceted and crucial for community cohesion. These interactions might include communication via chemical signals known as quorum sensing, which enables bacteria to coordinate behavior based on their population density. This signaling can lead to changes in gene expression that help the biofilm respond to environmental changes.
In addition to quorum sensing, physical interactions such as adhesion to surfaces and to each other are essential for biofilm stability. The ability for cells to adhere promotes community formation and is fundamental for biofilm establishment. Overall, the intricacies of cell-cell interactions provide a robust framework that underpins the survival and adaptability of biofilms.
Understanding the biological composition of biofilms is key to unraveling their complexities and implications for health, the environment, and technology.
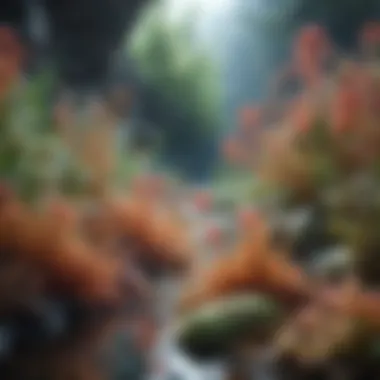
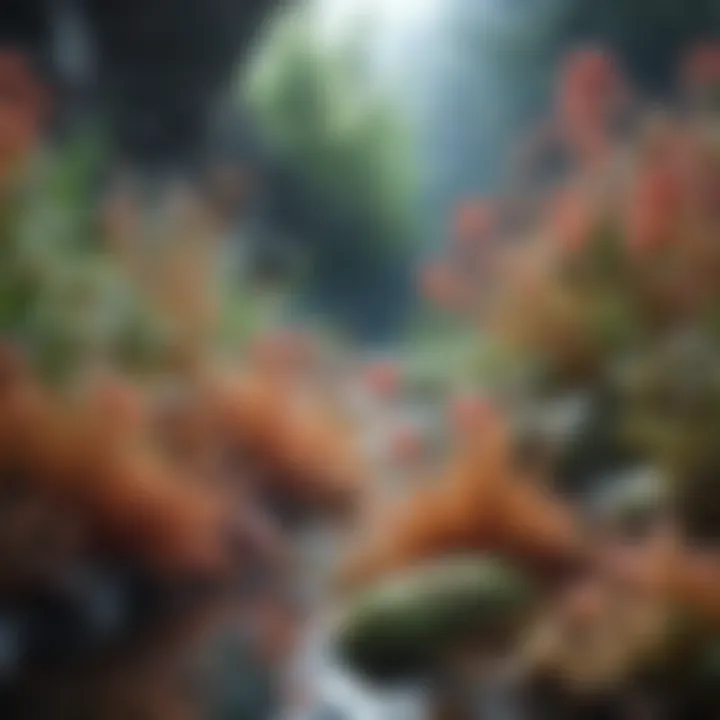
Ecological Significance of Biofilms
Biofilms play a vital role in ecological systems, influencing various processes that contribute to the overall health of their environments. Their complex structure allows for diverse microbial communities that can adapt and thrive in different ecological niches. Understanding their ecological significance is crucial for comprehending both natural and engineered systems. Biofilms can enhance nutrient cycling, contribute to biodiversity, and impact water quality, making them essential components of ecosystems.
Biofilms in Natural Ecosystems
In natural ecosystems, biofilms can be found in a variety of habitats, including rivers, lakes, and soils. They serve as a habitat for microorganisms, creating a niche where various species interact. This interaction promotes stability and resilience in ecosystems.
- Nutrient Cycling: Biofilms facilitate the breakdown of organic matter, which is pivotal in natural nutrient cycles. Microbes in biofilms break down complex organic substances, making nutrients available for primary producers, such as plants and phytoplankton.
- Natural Purification: In aquatic systems, biofilms contribute to water purification. They absorb and break down pollutants, which improves water quality. This function is crucial, particularly in streams and rivers, where biofilms mitigate the effects of nutrient runoff.
- Habitat Creation: By providing surfaces for colonization, biofilms create habitats for various microorganisms and larger organisms. This increases the overall diversity of life in ecosystems and supports food webs.
Studying biofilms in natural ecosystems can reveal patterns of microbial interactions and their impacts on overall ecological balance. It also helps in understanding how environmental changes influence these interactions.
Biofilms in Engineered Systems
Biofilms are not only crucial in natural environments but also in engineered systems, such as wastewater treatment plants, industrial processes, and even medical devices. Their presence can lead to both benefits and challenges.
- Wastewater Treatment: Engineered systems leverage biofilms to treat waste effectively. In these systems, biofilms are used to enhance the degradation of pollutants, contributing to cleaner effluents.
- Biofilm Formation in Medical Devices: While biofilms can be beneficial, they often lead to complications in medical settings. For example, in catheters or prosthetic devices, biofilm formation can lead to infections that are resistant to standard treatments. Understanding these processes is essential for developing strategies to mitigate such risks.
- Biofuel Production: Biofilms are also being researched for potential applications in the production of biofuels. They can efficiently break down organic matter, leading to increased yields of renewable energy sources.
Health Implications of Biofilm Bacteria
Biofilm bacteria present significant health implications, influencing both clinical outcomes and public health standards. Understanding the complexities of these microbial communities is vital for addressing their role in human diseases. Biofilms can hinder treatment efficacy, complicate infections, and contribute to persistent diseases, highlighting the need for effective intervention strategies.
Pathogenic Biofilms
Pathogenic biofilms are clusters of bacteria that adhere to surfaces in the body, forming a protective matrix. These biofilms are often resistant to antibiotics and are found in conditions like chronic wounds, dental plaques, and implant-associated infections.
The bacteria in these biofilms can communicate through signaling molecules, a process known as quorum sensing. This allows them to coordinate their behavior, enhancing their survival. Pathogenic biofilms can cause various health issues, including:
- Increased resistance to antibiotics, making treatment more challenging.
- Prolonged infections, as the protective nature of biofilms prevents immune cells from eradicating the bacteria.
- Biofilm-related infections, often requiring surgical intervention for removal.
"Biofilms can be responsible for up to 80% of chronic infections, demonstrating their clinical significance."
The pathogenic potential of biofilms necessitates more research into targeted therapies. New approaches such as anti-biofilm coatings or the use of enzymes to disrupt biofilm structure are being explored.
Biofilms in Chronic Infections
Chronic infections are often associated with biofilm formation. These infections usually persist despite ongoing treatment, causing significant morbidity. Common examples include:
- Cystic fibrosis, where biofilms from Pseudomonas aeruginosa contribute to lung infections.
- Urinary tract infections, often involving uropathogenic E. coli in biofilm form.
- Osteomyelitis, where bacteria can invade bone tissue and form biofilms, complicating treatment.
Biofilms in chronic infections play critical roles in:
- Biofilm resilience: Protecting bacteria from antibiotics and the host immune response.
- Recurrent infections: Even if initial treatment succeeds, biofilm residues can lead to reinfection.
- Systemic complications: Infections can spread beyond local sites if left untreated.
Biofilm Resistance Mechanisms
Understanding biofilm resistance mechanisms is crucial because it directly affects how we approach infections and other issues related to biofilms. Biofilms are known for their ability to withstand various environmental challenges, including antibiotic treatments. This resistance not only complicates treatment options for infections but also poses challenges in industrial and environmental contexts where biofilms are prevalent. By delving into the mechanisms that confer resistance, we can develop better strategies for control and remediation.
Antibiotic Resistance
Antibiotic resistance in biofilms is a significant concern in clinical settings. Bacteria within biofilms are often more resistant to antibiotics compared to their planktonic counterparts. Factors contributing to this resistance include:
- Altered Microenvironment: The structure of biofilms can create microenvironments that protect bacteria from antibiotic penetration. Nutrient gradients, cell density, and waste accumulation can impact the effectiveness of treatments.
- Gene Expression: Bacteria in biofilms may express specific genes that confer resistance. This can include genes that produce enzymes which degrade antibiotics or that change the target site of the drug.
- Persister Cells: Within biofilms, there are a subset of cells known as persisters that can survive antibiotic treatment. These cells are in a dormant state and can repopulate the biofilm once the antibiotic is removed.
Such resistance mechanisms have profound implications, particularly in chronic infections associated with medical devices. Efforts are ongoing to develop new strategies that target biofilm-specific features, to enhance the effectiveness of existing antibiotics.
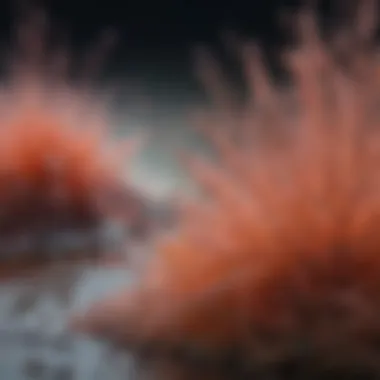
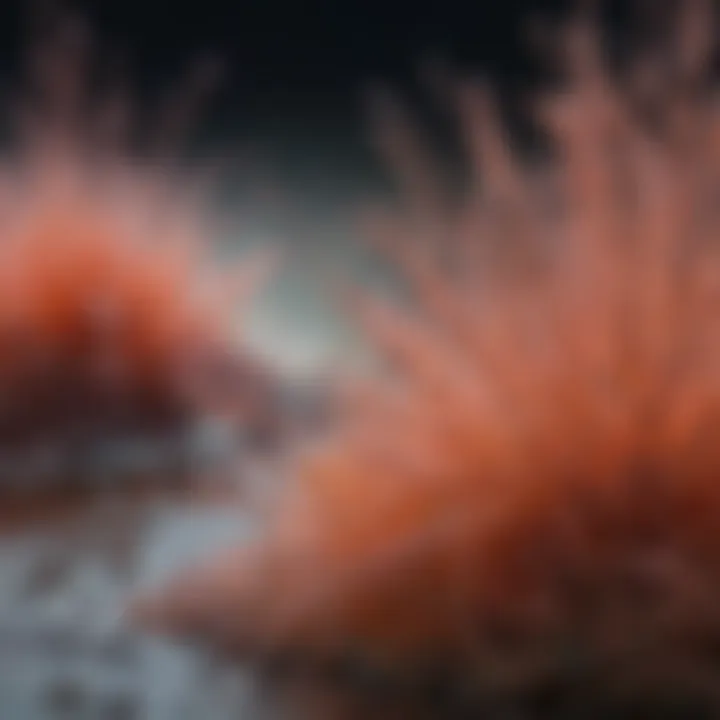
Environmental Stress Responses
Biofilm bacteria also exhibit various environmental stress responses that enhance their survival. These responses can occur in reaction to changes in temperature, pH, or the presence of toxic substances. Key adaptations include:
- Metabolic Adjustment: Biofilms can alter their metabolic pathways to utilize different energy sources under stress conditions. This flexibility allows the population to thrive in varying environments.
- Bulk Density Alterations: Some biofilms can modify their cell density or structure in response to external stresses. This can lead to changes in the biofilm's thickness, which may impact its susceptibility to treatments.
- Stress-Induced Gene Expression: Similar to antibiotic resistance, certain environmental stresses may prompt the expression of specific genes that help the biofilm withstand adversity.
These resistance mechanisms highlight the complex nature of biofilms. They illustrate the need for targeted research to better understand and combat biofilms in both medical and environmental settings. As researchers continue to explore biofilm behavior, the potential for innovative solutions will likely emerge.
The bacteria in biofilms outsmart antibiotics and environmental stressors, presenting a significant challenge in medicine and industry.
Detection and Analysis of Biofilms
The process of detecting and analyzing biofilms is crucial in understanding their complex nature and functionality. Detecting biofilms helps in recognizing their presence in various environments, which is pivotal for managing their effects on health and industry. Furthermore, understanding the composition and behavior of biofilms leads to better strategies for their control and manipulation. Since biofilms are hidden and can adapt to different environments, effective detection methods are necessary to reveal their impact on both ecosystem and human health.
Microscopic Techniques
Microscopic techniques are among the oldest yet reliable methods for biofilm detection. These techniques provide a direct visual insight into the composition of biofilms as well as their spatial arrangement. Common techniques include:
- Light Microscopy: Offers basic images of biofilm structure, using staining methods like crystal violet to enhance visibility.
- Fluorescence Microscopy: Allows observation of live biofilms, revealing not just the structure but also physiological states of individual cells within the biofilm. The use of fluorescent dyes can indicate viability or metabolic activity.
- Scanning Electron Microscopy (SEM): Provides high-resolution images, enabling detailed examination of biofilm architecture. SEM captures surface topography, which is useful for understanding physical interactions among the microbial community.
- Transmission Electron Microscopy (TEM): Offers insights into bacterial ultrastructures and the ultra-fine details of biofilm components.
While these techniques offer great detail, they also require specialized equipment. As a result, accessibility can be a challenge for many research institutions.
Molecular Methods
Molecular methods have emerged as powerful tools in the detection and analysis of biofilms. These techniques allow scientists to explore the genetic material of microbial communities within biofilms, providing insights into their diversity and function. Key methods include:
- Polymerase Chain Reaction (PCR): A technique that amplifies specific DNA sequences, making it easier to detect target microbial species in complex biofilm communities. These sequences can reveal insights into community composition and potential pathogenicity.
- Next-Generation Sequencing (NGS): Provides comprehensive genetic information from biofilm samples. NGS can elucidate the entire microbial community’s structure, identifying dominant species and their possible interactions.
- Fluorescent In Situ Hybridization (FISH): A technique that uses fluorescent probes to bind to specific RNA sequences in bacterial cells, allowing visualization under a microscope. It provides information about the spatial distribution of microbes within the biofilm.
Overall, molecular methods enable a deeper understanding of the dynamics and complexities of biofilms. They complement traditional microscopic techniques, providing an integrated approach to biofilm research.
Understanding both the microscopic and molecular methods is essential for researchers, educators, and industry professionals engaged in biofilm studies.
Strategies for Biofilm Control
Bacterial biofilms pose significant challenges in various fields, from healthcare to industrial processes. Effective strategies for biofilm control are thus essential to mitigate their adverse effects. This section delves into the methods utilized for disrupting or preventing the formation of biofilms. Understanding these strategies provides insights into managing biofilm-related issues and highlights the need for continued research and innovation.
Physical Disruption
Physical disruption methods involve mechanical ways to break the structure of biofilms. These techniques may include practices like scrubbing, ultrasound, and hydrodynamic shear forces. The application of these physical processes can effectively detach bacteria from surfaces, thereby reducing biofilm density.
- Scrubbing: This is a simple yet effective way of removing biofilms. It involves using brushes or other cleaning tools to scrub the surface. This technique is primarily used in healthcare settings where biofilms may form on medical equipment or surgical tools.
- Ultrasound: High-frequency sound waves can penetrate biofilm layers and disrupt the physical structure. This method can lead to the release of embedded bacteria. It is particularly useful for biofilms that are difficult to remove through traditional scrubbing or chemical methods.
- Hydrodynamic Shear Forces: Fluid flow can create shear forces that can dislodge biofilm organisms from surfaces. In industrial settings, adjusting the flow rates of liquids can help control biofilm growth on pipes and machinery.
While these methods can be very effective, there are considerations regarding the potential damage to surfaces or materials. Therefore, careful evaluation of the surface material and the biofilm's resilience is necessary before applying physical disruption tactics.
Chemical Agents
Chemical agents are vital in biofilm management as they target the microbial communities through various mechanisms. These agents can disrupt the extracellular polymeric substances (EPS) that hold the biofilm together, allowing for easier removal.
- Biocides: These substances kill or inhibit the growth of bacteria and other microorganisms. Common biocides include chlorine, hydrogen peroxide, and quaternary ammonium compounds. Proper concentration and application methods are crucial to ensure both effectiveness and safety.
- Enzymatic Treatments: Using enzymes, such as proteases or cellulases, can degrade specific components of EPS. This degradation can weaken the biofilm structure and enhance susceptibility to physical removal.
- Antimicrobial Peptides: These naturally occurring compounds can penetrate biofilm structures and disrupt microbial membranes. Their ability to work against a wide range of bacteria makes them a promising area of study in biofilm control.
Chemical agents require careful consideration of concentration and exposure time to balance effectiveness and minimize environmental impact. The risk of developing resistance is also a point of concern when using chemical treatments.
Effective control of biofilms is a multifaceted challenge and requires a combination of physical and chemical strategies for optimal outcomes.
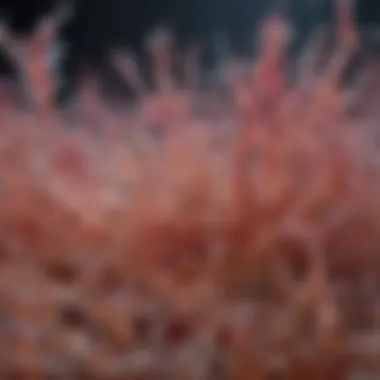
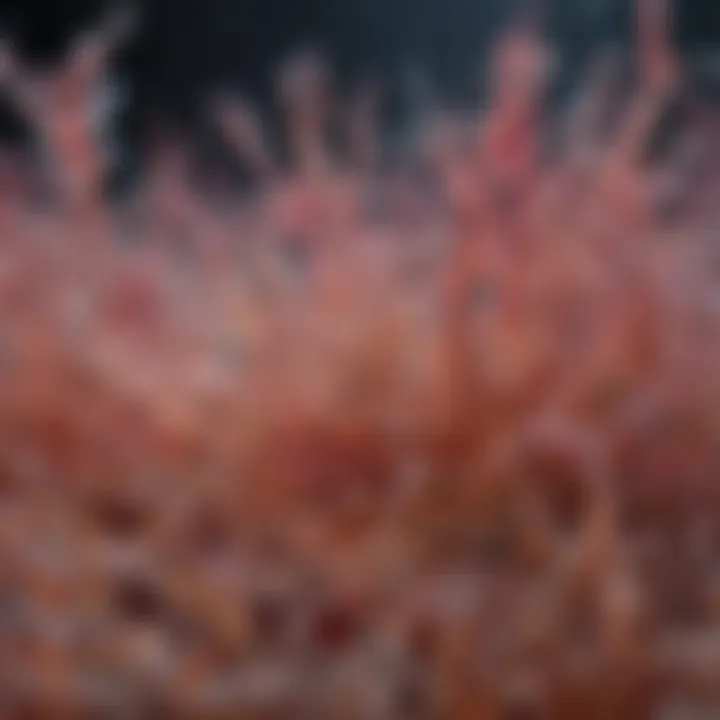
Applications of Biofilm Research
The study of biofilm bacteria has gained substantial attention due to their unique properties and implications in various fields. Understanding how these microbial communities interact, survive, and thrive in complex environments has opened new avenues for innovation and problem-solving. This section explores the critical applications of biofilm research in biotechnology, environmental science, and engineering.
Biotechnological Innovations
Biotechnological advancements often hinge on the ability to manipulate biological systems for human benefit. Biofilms play a pivotal role in several biotechnological innovations. Their structure allows for increased efficiency in bioprocesses, such as fermentation and biocatalysis. For instance, biofilm reactors capitalize on the innate resilience and high surface area of biofilms, enhancing productivity in wastewater treatment and waste recycling. They can also facilitate the production of pharmaceuticals and biofuels by hosting specific microbial communities that optimize metabolic pathways. Moreover, the genetic modification of biofilm-forming bacteria is leading to breakthroughs in areas like synthetic biology, where customized microbes can be designed for specific tasks.
Environmental Bioremediation
Biofilms are essential players in environmental bioremediation, a process using microorganisms to degrade contaminants in soil and water. The functional diversity of biofilm communities enables the breakdown of complex organic compounds, making them adept at tackling pollution. For example, biofilms can remove heavy metals and hydrocarbons from polluted sites. The inherent stability and self-regeneration capacity of biofilms allow them to thrive in harsh conditions where traditional remediation methods might fail. As a result, researchers are developing biofilm-based technologies to clean up oil spills and heavy metal-contaminated sites more effectively.
Bioengineering Solutions
The intersection of biofilm research and bioengineering has led to innovative solutions for various industrial challenges. Biofilms enhance wastewater treatment systems, improving nutrient removal and the degradation of organic matter. In the agricultural sector, fungi and bacteria that form biofilms can aid in soil remediation and nutrient cycling, promoting sustainable practices. Additionally, bioengineering efforts focused on biofilm formation are being utilized in creating advanced materials with novel properties. For instance, harnessing biofilms in coatings can provide antimicrobial resistance, prolonging the lifespan of medical devices and reducing the occurrence of related infections.
"Biofilm research offers transformative potential across multiple industries, marrying ecological understanding with practical applications that address pressing global challenges."
In summary, the applications of biofilm research are expansive and critical. They intertwine with the development of biotechnology, environmental sustainability, and engineering solutions, showcasing the versatility and importance of biofilms in addressing contemporary issues.
Future Directions in Biofilm Research
Research on biofilm bacteria has reached a pivotal juncture, and understanding future directions is crucial for both scientific advancement and practical applications. Biofilms are complex structures contributing to various industrial, ecological, and health-related issues. Therefore, exploring emerging technologies and interdisciplinary approaches could provide significant insights and drive innovative solutions for the challenges posed by biofilms.
Emerging Technologies
Emerging technologies are reshaping the landscape of microbiological research. These advancements offer new methods for studying biofilms, which can enhance our understanding of their behavior, composition, and interaction with the environment.
- Genomic Sequencing: Techniques like metagenomics allow researchers to analyze the genetic material in biofilms, revealing the diversity of bacterial communities. This can help identify keystone species and understand their roles in biofilm formation and stability.
- Microscale Imaging: Advanced imaging techniques such as atomic force microscopy and high-resolution fluorescence microscopy enable scientists to visualize biofilm architecture in real-time. This visual data can elucidate how biofilms develop and respond to external stimuli.
- Nanotechnology: The use of nanoparticles in biofilm research is gaining attention. Nanoparticles can be engineered to disrupt biofilm formation or enhance the delivery of antimicrobial agents, offering a potential strategy for combating pathogenic biofilms.
- Artificial Intelligence: Machine learning algorithms can analyze large datasets generated from biofilm studies, finding patterns that may not be evident through traditional analytical methods. These insights can lead to predictive models of biofilm behavior under various conditions.
Each of these technologies represents a frontier in biofilm research. By integrating these methods, researchers can form a comprehensive understanding of biofilms and develop targeted strategies for control and manipulation.
Interdisciplinary Approaches
Interdisciplinary collaboration is essential for addressing the complexities associated with biofilms. Different fields can contribute unique perspectives and expertise that enrich the study of biofilms and their implications in various settings.
- Microbiology and Engineering: Collaborating across these domains can result in improved bioreactor designs, optimizing conditions for microbial growth while managing biofilm development.
- Environmental Sciences: Insights from environmental disciplines can guide how biofilms influence nutrient cycling and ecosystem health, especially in aquatic systems. They can also provide strategies for bioremediation efforts that harness biofilms' natural capabilities.
- Medicine and Public Health: Research in clinical settings can bridge gaps regarding disease management linked to biofilms. By understanding how biofilms form on medical devices or within the human body, innovative treatment options can arise, potentially leading to better patient outcomes.
- Data Science and Computational Biology: By applying computational techniques to biofilm research, researchers can model complex interactions and predict responses to environmental changes or treatments. This partnership allows for the integration of vast datasets, enhancing the overall understanding of biofilms.
The interplay between various scientific disciplines paves the way for groundbreaking discoveries in biofilm research, ultimately leading to improved management strategies and therapeutic approaches.
The End
The conclusion of this article serves as a critical reflection on the complex nature of biofilm bacteria and their multifaceted implications. Understanding biofilms enhances our comprehension of microbial life forms that exist in varied environments, from natural ecosystems to engineered systems. The implications of biofilm research extend beyond mere academic understanding, affecting public health, environmental management, and industrial bioprocess optimization.
In this article, significant key elements were addressed. Biofilms play a pivotal role in both promoting ecosystem stability and presenting challenges in medical settings due to their resistance properties. The examination of treatment strategies for pathogenic biofilms reveals that despite progress, significant challenges remain in effectively combating these resilient structures. Equally crucial is the understanding of biofilm interactions with antibiotics, which presents unique avenues for future pharmacological developments.
Furthermore, this article highlights the beneficial applications of biofilm research in biotechnological innovations. An increased grasp on the mechanisms and behavior of biofilms can lead to enhanced techniques in bioremediation and environmental protection. As microbiology continues to evolve, novel approaches to biofilm management will likely emerge, demonstrating the significance of continuous research in this field.
As we synthesize the information presented, it is evident that understanding biofilm bacteria is essential. It encourages collaboration between disciplines, contributes to advancements in technology and medicine, and ultimately fosters a deeper appreciation for the complexities of microbial life. > This compels an integrated understanding of biofilms, as their presence and behaviors carry vast implications in many aspects of life and industry.
Summary of Key Insights
- Biofilms characterize a collection of microbial communities that can thrive in diverse environments.
- Their structural composition and associated extracellular polymeric substances contribute to their resilience and functionality.
- Biofilm bacteria pose both threats in health care settings and opportunities in biotechnological applications.
- Research into biofilm resistance mechanisms is crucial for developing effective treatment strategies.
Implications for Future Research
The necessity of further research into biofilms cannot be overstated. Potential areas of exploration include:
- Emerging detection techniques: Innovations in identifying biofilm presence early can lead to better management strategies.
- Targeted biofilm eradication: Studying the mechanisms by which biofilms resist treatments can inform better therapeutic approaches.
- Interdisciplinary studies: Collaboration between microbiologists, engineers, and environmental scientists can yield new insights and innovations.
Continued research into these areas will better equip us to harness biofilms for beneficial purposes while mitigating their adverse impacts.