Understanding Cell Differentiation: Mechanisms and Implications
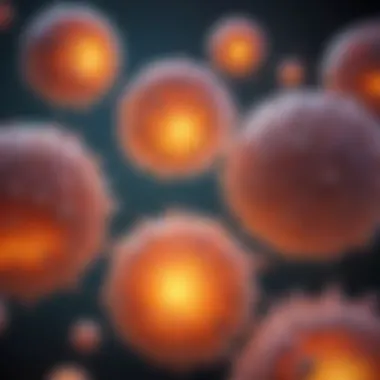
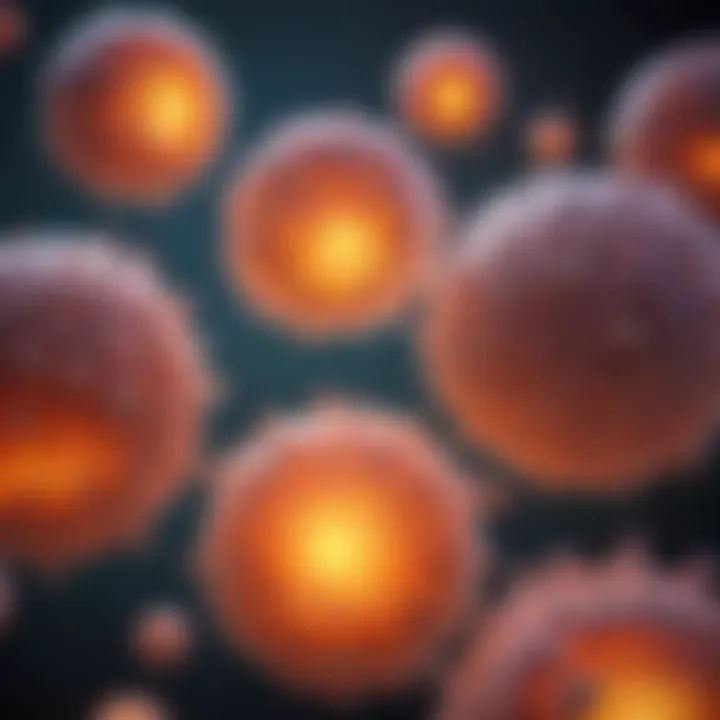
Intro
Cell differentiation is a fundamental process in biological systems. It transforms unspecialized cells, like stem cells, into distinct types that perform specific functions in the body. This process is not merely a switch that turns one kind of cell into another; rather, it involves an intricate interplay of genetic, epigenetic, and environmental factors. By understanding how cells differentiate, we can gain insights into development, disease mechanisms, and even regenerative medicine.
Research Overview
Cell differentiation is one of the most researched topics in biology. Key findings suggest that intrinsic factors, such as gene expression patterns, along with extrinsic stimuli, like signaling molecules, guide the differentiation pathway of cells. This research is crucial as it sheds light on developmental biology and medicine.
- Summary of key findings: Studies have demonstrated that the activation of specific genes is essential for the formation of specialized cells. For instance, transcription factors can turn on or off genes that influence a cell's fate. External signals, such as growth factors, can enhance or inhibit these processes.
- Importance of the research in its respective field: Understanding these mechanisms informs us about developmental anomalies and a range of diseases, from cancer to degenerative disorders. Moreover, it holds potential for advancements in regenerative medicine, as insights gained can direct stem cell therapies and tissue engineering.
Methodology
The methodologies employed in the study of cell differentiation are diverse and multifaceted. Researchers typically use a combination of experimental and analytical methods to dissect this complex biological process.
- Description of the experimental or analytical methods used: Techniques often encompass genetic manipulation, such as CRISPR-Cas9, alongside staining and imaging methods to observe differentiation. Culturing cells in specific conditions helps mimic developmental environments, facilitating the study of cell behavior.
- Sampling criteria and data collection techniques: Sampling usually involves specific cell types. Embryonic stem cells are frequently utilized for understanding differentiation pathways due to their capacity to develop into any cell type. Data collection may include quantitative PCR for gene expression analysis or flow cytometry for cell sorting.
The findings from these methodologies contribute significantly to the broader field of developmental biology, enhancing our understanding of how complex organisms develop from a single cell.
Prolusion to Cell Differentiation
Cell differentiation serves as a cornerstone in the field of biology, reflecting how unspecialized cells evolve into various cell types, each tailored with unique functionalities. This transformation is pivotal not just for development but also for maintaining the intricate balance necessary for proper biological function throughout an organism's life.
The significance of understanding cell differentiation extends beyond the academic realm; it holds practical implications in medicine and biotechnology. Recognizing how cells acquire distinct identities can inform therapeutic strategies for conditions like cancer, where differentiation pathways may be disrupted.
In this section, we will delve into the definition of differentiation and its importance, followed by an overview of cellular development. These discussions will lay the foundation for deeper explorations into the mechanisms and implications of cell differentiation as covered in the subsequent sections of this article.
Definition and Importance of Differentiation
Differentiation is defined as the process by which a less specialized cell becomes a more specialized cell type. This process is crucial during the development of multicellular organisms, impacting everything from the formation of tissues to the function of vital organs. The implications of differentiation are profound because they determine the identity and functionality of each cell in an organism. For instance, a stem cell can differentiate into various cell types, playing multiple roles depending on the signals it receives from its environment.
The importance of differentiation creates direct pathways to understanding developmental biology. Any disruptions in the differentiation process may lead to developmental disorders or diseases, including cancer. Therefore, the study of cell differentiation is vital for designing targeted treatments and regenerative medicine strategies that harness the body's ability to repair or restore damaged tissues.
Overview of Cellular Development
Cellular development encompasses several processes that occur from fertilization to the formation of complex tissues and organs. In the earliest stages, the zygote divides and differentiates, establishing the foundational cell types. This initial differentiation is often influenced by intrinsic genetic programming and extrinsic signals from the cellular environment.
Throughout development, cells continuously communicate. This communication is mediated through signaling pathways that guide cells to become specific types. For example, cells in the developing nervous system receive signals that promote their differentiation into neurons, while those near the gut receive different signals that direct them to become intestinal cells.
Understanding cellular development is essential as it reveals patterns and stages that cells undergo during differentiation. This knowledge is not only fundamental in developmental biology but also crucial in regenerative medicine and therapeutic interventions.
"Differentiation is the biological process through which cells gain distinct identities, a phenomenon fundamental to the structural and functional diversity of life."
In summary, the introductory section unveils the centrality of cell differentiation within biological systems. This narrative sets the stage for a more in-depth exploration of the mechanisms involved, highlighting its crucial role in both health and disease.
Historical Perspectives
Understanding the historical backdrop of cell differentiation is essential in grasping how contemporary views and approaches have evolved. The journey of scientific thought in this domain spans centuries, reflecting shifts in knowledge that impact present-day research and applications. This exploration not only elucidates how past theories laid the groundwork for current understanding but also highlights the iterative nature of scientific inquiry. Through examining early notions to modern insights, one can appreciate the complexities and adaptative mechanisms of cell differentiation and its significance in biology.
Early Theories of Differentiation
The early theories of cell differentiation primarily grappled with the concept of how a single fertilized egg could develop into a multitude of distinct cell types, each with unique functions. One pivotal idea was the preformation theory, which suggested that organisms develop from miniature versions of themselves. This notion implied that all cells were pre-formed, needing only to grow larger. Such ideas were prevalent until the 19th century.
Another influential perspective came from the cell theory proposed by scientists like Matthias Schleiden and Theodor Schwann. They posited that all living things are composed of cells, which are the fundamental units of life. While cell theory promoted an understanding of cells as the building blocks of organismal structure, it did not fully account for differentiation.
"The cell theory revolutionized biology but left many questions about how cells acquire specific functions unanswered."
Only later did researchers begin to realize that differentiation is a complex, dynamic process driven by numerous factors, rather than a linear progression from a pre-formed state.
Modern Understanding
In contemporary science, cell differentiation is viewed as a highly regulated process influenced by an array of genetic and environmental factors. The discovery of stem cells significantly altered the framework of differentiation studies. Researchers found that stem cells have the capacity to develop into various specialized cell types, which suggested that differentiation is not a one-way street but includes potential for plasticity.
Modern tools like CRISPR and advanced imaging techniques have enabled scientists to dissect the mechanisms of differentiation at an unprecedented level. This includes the understanding of pathways such as the Wnt and Notch signaling pathways, which play crucial roles in regulating how stem cells differentiate into specific cell types.
Furthermore, genomics has shed light on how gene expression is intricately linked to differentiation. The recognition that transcription factors can activate or repress genes essential to cell fate decisions has transformed insights into the field. Researchers are now exploring not only genetic regulation but also epigenetic modifications, which can stably change gene expression without altering the underlying DNA sequence, suggesting a dual layer of regulation in differentiation processes.
The modern approach interlinks multiple disciplines, including genetics, cellular biology, and developmental biology, enhancing the understanding of differentiation's role in health and disease. Such understanding lays the foundation for advancements in regenerative medicine, advancing our capability to manipulate cell fates for therapeutic benefits.
Genetic Regulation of Differentiation
Genetic regulation of differentiation is a fundamental aspect of developmental biology. It focuses on how genes control the process by which unspecialized cells transform into various specialized cells. Understanding these mechanisms is essential for several reasons. First, they clarify how specific cell types arise from a common progenitor. Second, they illuminate the potential for manipulating these processes in research and medicine, especially in regenerative therapies and cancer treatment. The expression of genes at precise times and locations is crucial for the proper development of tissues and organs.
Role of Genes in Differentiation
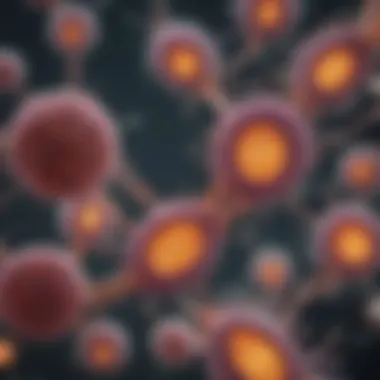
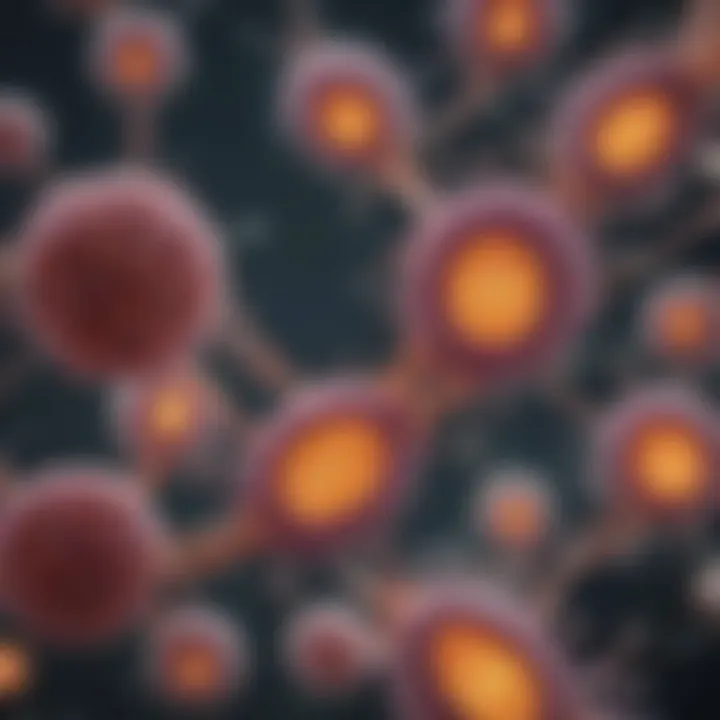
Genes serve as the blueprint for cellular functions and characteristics. In the context of differentiation, specific genes are turned on or off at various stages, guiding the transition from pluripotent stem cells to specialized cell types.
- Key Genes: Certain genes, such as those in the homeobox family, play essential roles in regulatory networks that dictate cell fate.
- Gene Expression Patterns: The pattern of gene expression influences not only the type of cell that will develop but also its fate throughout the organism's life.
- Mutations and Differentiation: Mutations in differentiation-related genes can lead to abnormal cellular functioning, which may cause diseases including cancer.
This intricate regulation underpins the complexity of multicellular life. Understanding how specific gene functions contribute to differentiation can provide insights into both normal development and disease mechanisms.
Transcription Factors and Differentiation
Transcription factors are proteins that bind to specific DNA sequences, controlling the transcription of genetic information from DNA to messenger RNA. They are pivotal in regulating differentiation.
- Role of Transcription Factors: These factors act as molecular switches that can turn genes on or off in response to external and internal signals.
- Examples of Key Factors: Proteins such as Oct4, Sox2, and Nanog are crucial for maintaining pluripotency. Conversely, they also play roles in triggering differentiation under appropriate conditions.
- Networks of Interaction: Transcription factors do not operate alone; they interact within complex networks. The balance of signals from transcription factors ultimately leads to specific cell fates.
Understanding the regulation by transcription factors is important as it offers new avenues for controlling differentiation in clinical applications, such as tissue engineering and stem cell therapy.
Differentiation Genes and Pathways
Differentiation genes are specific genes that directly influence the fate of cells as they develop into specialized types. These genes can be grouped into several pathways, each playing unique roles in the differentiation process.
- Signaling Pathways: Several key pathways, such as Wnt, Notch, and Hedgehog, are critically involved in regulating differentiation.
- Interaction with Other Factors: These pathways often require input from other signaling molecules to modulate gene expression properly and influence the differentiation cascade.
- Feedback Mechanisms: Feedback mechanisms within these pathways help maintain cell identity and prevent aberrant differentiation processes.
Gaining insight into differentiation genes and their related pathways helps elucidate how cells normally develop and how dysregulation can lead to health issues.
"Understanding genetic regulation in differentiation illuminates pathways for novel therapeutic strategies in medicine."
By maintaining a focus on the genetic and molecular basis of differentiation, researchers can reveal the underlying principles governing cellular development and how we might harness these insights for future applications.
Cellular Signaling Pathways
Cellular signaling pathways play a pivotal role in the process of cell differentiation. They dictate how cells respond to various external and internal stimuli, guiding their development into specialized types necessary for organismal function. This section will focus on the significance of these pathways, exploring both extracellular signals and intracellular mechanisms.
Extracellular Signals
Extracellular signals are essential in directing the behavior of cells in their environment. These signals typically consist of proteins or other molecules released by neighboring cells, which bind to receptors on the surface of target cells. The interaction initiates a cascade of reactions inside the cell, which may lead to changes in gene expression, metabolic activity, or even cell fate decisions.
For example, growth factors such as fibroblast growth factor (FGF) and nerve growth factor (NGF) are known to promote differentiation. They perform this function by activating specific receptor tyrosine kinases. Upon binding, these receptors undergo dimerization and autophosphorylation, leading to the activation of downstream signaling pathways such as the MAPK/ERK pathway. This pathway ultimately influences transcription factors that drive the cells toward differentiation.
The importance of understanding extracellular signals cannot be understated, as they mediate communication between cells in various contexts, such as tissue repair, immune responses, and development. Their dysregulation may lead to diseases such as cancer, where cells may grow uncontrollably due to unchecked signaling.
"Cellular signaling pathways are the languages through which cells communicate their needs, intentions, and developmental cues."
Intracellular Signaling Mechanisms
Once extracellular signals bind to their respective receptors, intracellular signaling mechanisms are activated. These mechanisms involve a series of proteins and enzymes that relay the signal from the cell membrane to the nucleus. This transition is crucial as it translates external cues into specific cellular outcomes.
Several key players are involved in these signaling pathways, including kinases, phosphatases, and transcription factors. For instance, the activation of protein kinase A (PKA) upon binding of a hormonal signal can lead to phosphorylation of target proteins, influencing various cellular processes including differentiation.
Moreover, the involvement of second messengers, such as cyclic AMP (cAMP) and calcium ions, is significant in amplifying the signals within cells. These molecules facilitate rapid responses to external changes, enabling cells to adapt quickly during differentiation processes.
Conclusively, understanding intracellular signaling mechanisms is essential for comprehending how cells interpret signals and make fate decisions. By mapping these pathways, researchers can identify targets for therapeutic interventions in various diseases, including developmental disorders and cancer.
Epigenetic Modifications
Epigenetic modifications play a pivotal role in cell differentiation. They define how genes are expressed without altering the underlying DNA sequence. This is crucial in creating the diverse cell types observed in multicellular organisms. Understanding these modifications provides insights into cell identity and function. Moreover, epigenetic changes influence how cells respond to environmental signals. Thus, they serve as a bridge between genetics and phenotype, showcasing the dynamic nature of biological systems.
Role of Epigenetics in Differentiation
Epigenetics refers to heritable changes in gene expression that do not involve modifications to the DNA sequence itself. These changes can alter how cells differentiate into various specialized types. The role of epigenetics in differentiation is multifaceted, involving processes like DNA methylation and histone modification.
- DNA Methylation: This process generally silences gene expression. When methyl groups are added to cytosine bases in DNA, it can hinder the transcription machinery from accessing the gene. For specific genes crucial for differentiation, methylation patterns are modified, allowing expression appropriate for a given cell type.
- Histone Modification: Histones are proteins around which DNA is wrapped. Changes in the chemical structure of histones can promote or inhibit transcription. For example, acetylation of histones usually leads to an open chromatin structure, promoting gene activity necessary for differentiation.
These mechanisms allow undifferentiated cells to transition into specialized cells by turning on or off specific genes. Thus, epigenetics acts as a regulatory layer, ensuring that differentiation happens in a timely and organized fashion.
Chromatin Structure and Accessibility
The structure of chromatin is integral to gene regulation during cell differentiation. Chromatin, composed of DNA and proteins, can exist in two forms: euchromatin and heterochromatin.
- Euchromatin is a less dense form that allows access for transcription factors and other regulatory proteins. Its presence indicates active gene expression, which is essential for differentiation.
- Heterochromatin, in contrast, is tightly packed and generally inactive in terms of transcription. This form protects genes from being turned on prematurely, ensuring a proper differentiation pathway.
The accessibility of chromatin changes during differentiation. Factors such as transcription factors and non-coding RNAs can facilitate or inhibit chromatin remodeling, which alters gene expression patterns. Understanding chromatin accessibility can reveal how specific signals drive differentiation and maintain the identity of differentiated cells.
"Epigenetic modifications bridge the gap between genetic instructions and the functional realities of cells, shaping the pathways of differentiation across diverse biological contexts."
In summary, epigenetic modifications are crucial in cell differentiation, influencing how genes are expressed without changing the DNA sequence. Their impact on chromatin structure highlights the complexities and intricacies involved in defining cellular identity.
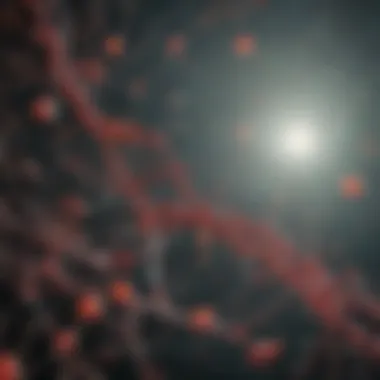
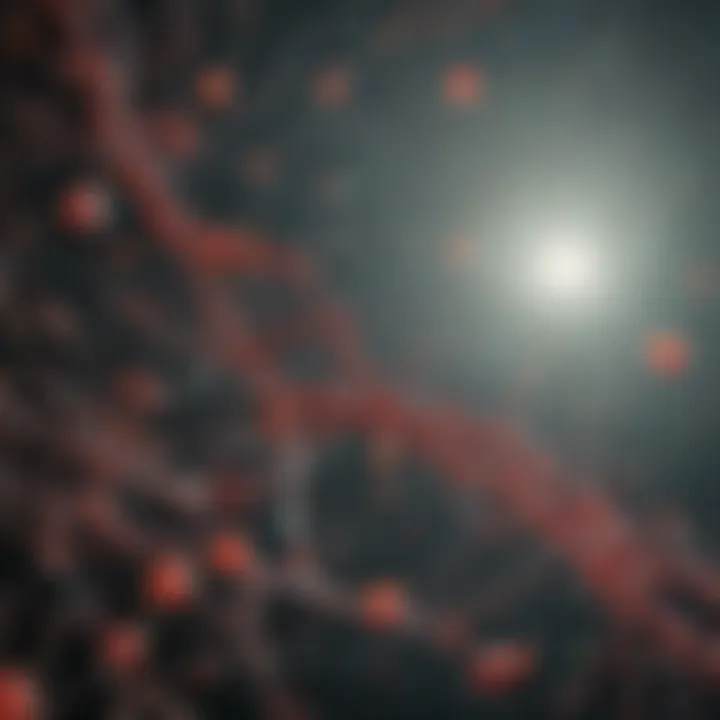
Types of Cell Differentiation
Understanding the different types of cell differentiation offers vital insights into biological processes. Differentiation enables unspecialized cells to acquire unique functions that facilitate the entire organism’s development. This section elucidates two essential types of differentiation: differentiation in embryonic development and the roles of adult stem cells and plasticity.
Differentiation in Embryonic Development
During embryonic development, cell differentiation is a fundamental process that guides the formation of specialized tissues and organs. This process begins with a single fertilized egg, or zygote, which undergoes numerous divisions, eventually leading to a multicellular embryo.
As the embryo develops, cells begin to specialize. This specialization is critical as it establishes the various cell types required for proper organ function. For instance, some cells will become neurons, while others will form muscle cells or epithelial cells. The region of differentiation is orchestrated by intrinsic genetic information and extrinsic environmental signals.
Key Factors Influencing Embryonic Differentiation:
- Gene Expression: Specific genes are activated to guide cell fate.
- Signaling Molecules: Growth factors and hormones facilitate communication between cells.
- Cellular Environment: The surrounding matrix plays a role in guiding differentiation.
The process is tightly regulated to ensure that cells differentiate at the right time and place, which is crucial for forming a functional organism. Any disruption can lead to developmental disorders or congenital malformations.
Adult Stem Cells and Plasticity
Adult stem cells present in various tissues exhibit remarkable plasticity. This means they retain the capacity to differentiate into multiple cell types even after development. They serve as a repair system for the body, contributing to maintenance and regeneration.
Adult stem cells are distinct from embryonic stem cells because they are already partly differentiated. However, their ability to adapt and provide new cells where needed highlights their importance in health and disease contexts. For example, hematopoietic stem cells in the bone marrow can produce different types of blood cells.
Characteristics of Adult Stem Cells:
- Self-Renewal: They can divide and replicate to maintain their population.
- Multilineage Potential: They can differentiate into several cell types, though typically limited to their tissue of origin.
- Response to Injury: They mobilize to areas of damage to support tissue repair.
"Adult stem cells are invaluable in regenerative medicine, as they hold promise for therapies against degenerative diseases."
This adaptability and the ability to switch fates contribute to fields like therapy and tissue engineering, demonstrating the indispensable role adult stem cells play in modern biology.
Understanding these types of differentiation is essential not only for comprehending normal biological functions but also for tackling challenges in medicine and developmental biology.
Cell Lineage and Fate Mapping
Cell lineage and fate mapping are vital concepts in understanding cell differentiation and its implications. These methodologies allow scientists to trace the developmental history of individual cells, providing insight into how differentiated cell types arise from progenitor populations. The study of cell lineages enables researchers to elucidate the connections between different cells, making it easier to understand both normal and abnormal development processes. The implications of this understanding extend across many fields, including developmental biology, regenerative medicine, and cancer research.
The benefits of these approaches are manifold:
- Understanding Development: Tracking cell lineages provides clarity on how different cell types emerge during embryonic development.
- Addressing Diseases: It allows researchers to follow the pathways that lead to disorders such as cancer, where cell differentiation goes awry.
- Regenerative Medicine: Cell fate mapping enhances techniques aimed at tissue regeneration by revealing how stem cells can be utilized effectively.
Despite their importance, cell lineage and fate mapping face certain considerations. For example, ethical issues arise when studying embryonic stem cells, which need careful management. Furthermore, the accuracy of lineage tracing can sometimes be compromised by technical limitations.
"Understanding the intricate pathways of cell lineage can illuminate not just normal development, but also anomalies that lead to diseases."
Tracking Cell Lineages
The process of tracking cell lineages primarily involves identifying and labeling cells at specific developmental stages. This could be achieved through various techniques such as genetic markers or lineage tracing agents. In bedrock biology, this tracking often starts at the single-cell level, providing a detailed map of how individual cells contribute to an organism’s structure and function.
Several common methods include:
- Genetic Labeling: Techniques such as Cre-Lox and fluorescent reporters allow scientists to mark specific cells, tracking their divisions and fates through generations.
- Single-Cell RNA Sequencing: This technique provides a snapshot of the transcriptome of individual cells, revealing which genes are active in specific cell types and providing insights into their lineage.
- CRISPR/Cas9: This powerful gene-editing tool can be utilized to insert labels into the genomic DNA, aiding in lineage tracing.
Each of these methods allows for a deeper understanding of how cells relate contextually and temporally during organism development.
Technological Advances in Fate Mapping
Recent advancements have significantly boosted the resolution and accuracy of fate mapping. Traditional techniques have evolved, employing cutting-edge technology to create more detailed lineage maps. The use of advanced imaging technologies, such as live-cell imaging and high-resolution microscopy, has improved the ability to visualize cellular processes in real-time.
Opportunities provided by these advancements include:
- High-Dimensional Imaging: Methods like light-sheet fluorescence microscopy allow for three-dimensional imaging of living embryos, making it easier to track cellular movements and fates.
- Machine Learning: Algorithms can now analyze vast datasets generated from lineage tracking studies, identifying patterns that were previously difficult to discern.
- In Vivo Techniques: Techniques like optogenetics enable the manipulation of specific cells within a living organism, followed by real-time monitoring of their differentiation outcomes.
These technologies not only enhance our understanding of cell differentiation but also provide tools for potential therapeutic applications. The integration of technology in lineage and fate mapping stands as a beacon of progress in both research and clinical arenas.
Implications of Differentiation
Cell differentiation is not only a fundamental biological process but also holds significant implications in various fields, particularly in health and medicine. Understanding how cells differentiate helps in comprehending the intricate networks that govern growth, repair, and functionality in organisms. This section delves into the implications, exploring the relevance of cell differentiation in health and disease, as well as its role in regenerative medicine.
Differentiation in Health and Disease
The process of cell differentiation plays a crucial role in maintaining homeostasis within the body. When cells differentiate as expected, they perform their designated functions effectively, contributing to overall health. For instance, specialized cells in the immune system differentiate to provide defense against pathogens. On the other hand, abnormalities in cellular differentiation can lead to diseases, most notably cancer.
Cancer arises when the regulatory mechanisms of differentiation fail, leading to uncontrolled cell growth. Here, cells do not lose their ability to grow, and often, they do not differentiate properly. This improper differentiation creates a population of cells that can proliferate uncontrollably, forming tumors. Understanding the pathways that govern differentiation could unveil new therapeutic targets for cancer treatment.
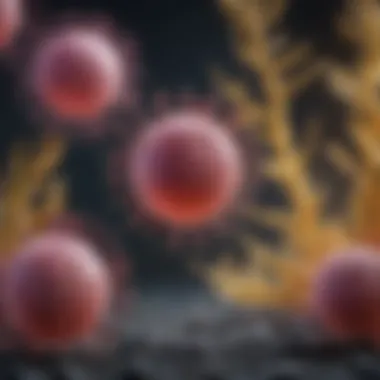
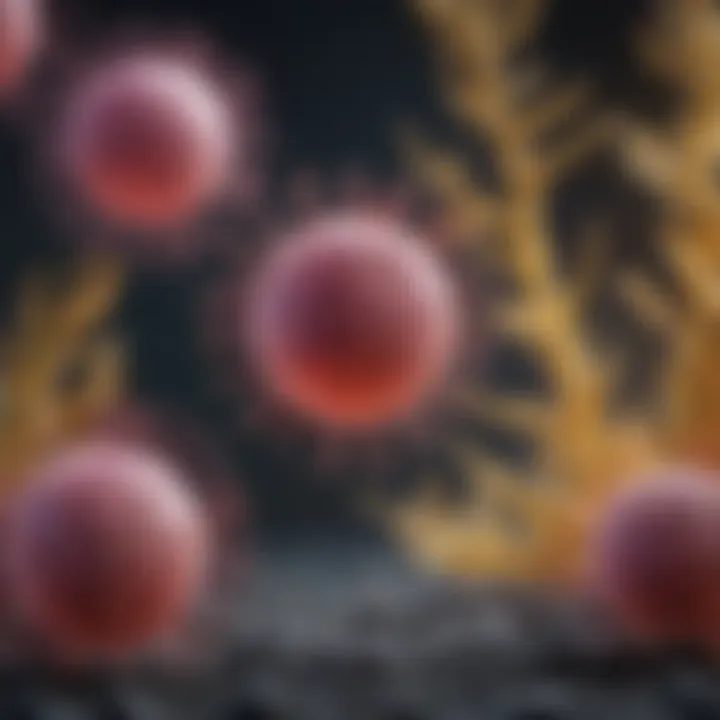
In addition, other diseases related to differentiation include various genetic disorders. For example, mutations in genes responsible for differentiation pathways can result in conditions like muscular dystrophy or neurodegenerative disorders. Thus, studying differentiation also helps in identifying potential biomarkers for such diseases, allowing for earlier diagnosis and intervention.
"The failure of proper cell differentiation is often at the heart of many diseases, particularly cancers and genetic disorders."
Role in Regenerative Medicine
The significance of differentiation extends into the realm of regenerative medicine, which seeks to restore the normal function of damaged tissues and organs. Stem cells, due to their ability to differentiate into various cell types, are pivotal in this area of science. By understanding how stem cells differentiate into specific cell types, researchers can enhance the potential for tissue engineering and cell therapy.
For example, in conditions like spinal cord injuries or heart disease, the ability to generate cells that can replace damaged tissues can revolutionize treatment methods. For instance, deriving cardiomyocytes from induced pluripotent stem cells can potentially repair heart damage. Similarly, differentiating neural stem cells into neurons offers hope for neurodegenerative diseases.
The implications for therapeutic applications are vast. With advancements in technology and a deeper understanding of the mechanisms that control differentiation, scientists are developing new strategies to harness the body’s own regenerative capabilities. This approach not only holds promise for treating currently incurable diseases but also emphasizes the importance of differentiation in therapeutic contexts.
Challenges and Controversies
Cell differentiation is a complex and essential process in biology, but it comes with its own set of challenges and controversies. These debates make clear the need for continued exploration and understanding of this area. One significant challenge is the nuanced understanding of how differentiation can vary between cell types and organisms. The mechanisms that drive differentiation can result in a myriad of cell behaviors, leading to differences in tissue development and functionality, which raises questions about the predictability of these processes.
In the realm of scientific research, differing opinions on the fundamental aspects of differentiation have emerged. Researchers often debate over the role of specific genes, the impact of various signaling pathways, and how these elements interact with epigenetic modifications. These discussions are essential as they can influence experimental approaches and the interpretation of results. Furthermore, these debates often ignite discussions that can lead to advances in research methodologies and deepen our understanding of cellular processes.
Scientific Debates in Cell Differentiation
Scientific debates in cell differentiation primarily focus on the mechanisms driving this process. One major area of dispute involves how much influence inherent genetic programming has over the differentiation process compared to the external environment. Some scientists argue that genetic factors are predominant, while others emphasize the role of environmental factors such as nutrient availability or mechanical forces.
Another critical debate centers around the concept of plasticity in differentiated cells. Can mature cells revert to a more stem-like state? Research has shown that certain differentiated cells can indeed exhibit plasticity, leading to ongoing discussions about how this ability could be harnessed in regenerative medicine.
Examples of core scientific debates include:
- The role of transcription factors: How do these proteins regulate gene expression during differentiation?
- The impact of extracellular signaling: What external signals are crucial for guiding differentiation?
- The balance between stability and flexibility: How do cells maintain their identity once differentiated?
Ethical Considerations
The progress in understanding cell differentiation also raises ethical considerations that cannot be overlooked. As methods such as stem cell therapy and genetic engineering evolve, questions arise regarding the moral implications of manipulating cell fates.
For example, the use of embryonic stem cells in research is a contentious issue, leading to discussions about the moral status of embryos. Opponents argue that utilizing embryos for research poses ethical dilemmas, while proponents emphasize the potential benefits for regenerative medicine and disease treatment.
Moreover, genetic modification techniques, like CRISPR, can alter differentiation processes at the genetic level. This also prompts questions about the potential for unintended consequences in altered cell behaviors, which might pose risks to health or the environment.
Key ethical discussions include:
- The morality of using human embryos for research and treatment.
- Consent issues surrounding the use of cells from donors.
- The impact of genetic editing on future generations and biodiversity.
These challenges and controversies not only highlight the complexities involved in understanding cell differentiation but also underscore the importance of a balanced approach toward research in this vital area.
Future Directions in Research
Cell differentiation is a field of study that continues to evolve. New discoveries provide insight into the complexities of how cells decide their fate. Research in this area not only enhances our understanding but also opens new avenues for application in medicine and biotechnology.
"The future of cell differentiation research is closely tied to the advancements in our technological capabilities and understanding of biological processes."
Emerging Technologies
Emerging technologies play a vital role in advancing our knowledge of cell differentiation. Techniques such as CRISPR-Cas9 gene editing allow researchers to manipulate genes with precision, shedding light on the specific roles of various genes in this process. Another important technology is single-cell RNA sequencing. It enables the examination of gene expression at the individual cell level. This level of detail helps identify how different cells differentiate in response to various stimuli.
Additionally, organoid technology provides a way to study cell differentiation in a more controlled and realistic environment. Organoids, which are miniaturized and simplified versions of organs, can mimic the in vivo conditions that promote differentiation. This technology can provide significant insights into developmental biology and disease modeling.
Potential Applications
The potential applications of advancements in cell differentiation research extend to various domains. In regenerative medicine, understanding the differentiation process can lead to innovative therapies for conditions like spinal cord injuries or degenerative diseases. By harnessing stem cells and guiding their differentiation, scientists aim to create replacement tissues or organs.
In cancer research, exploring how differentiation relates to tumor formation can aid in developing targeted therapies. Cancer cells display aberrant differentiation patterns. Recognizing these patterns can help in identifying new treatment strategies to revert cancer cells back to a more differentiated state, which is typically less aggressive.
Further, the biotechnology sector can benefit from insights into cell differentiation for enhanced production of therapeutics, including monoclonal antibodies and insulin. Understanding how to manipulate cell fate can lead to more efficient bioproduction processes.
Summary and The End
Cell differentiation is an essential process in the biological sciences that deserves significant attention. This article dives into the multifaceted mechanisms that underlie differentiation. Understanding these mechanisms is crucial for comprehending how unspecialized cells evolve into specialized types, performing distinct functions essential for growth, repair, and overall organismal health.
In the summary, we revisit the main themes explored throughout the sections. Key points include the genetic regulation of differentiation, highlighting the roles of transcription factors and the intricate pathways that govern these processes. Cellular signaling pathways also play a vital role, as they relay external signals that can influence differentiation outcomes. The section on epigenetic modifications emphasizes how these factors can alter gene expression without changing DNA sequences, thereby impacting cell fate decisions.
The implications of differentiation, specifically in health and disease, shed light on how aberrations in this process can lead to disorders such as cancer. Furthermore, regenerative medicine benefits greatly from understanding differentiation, as harnessing stem cells can lead to therapies for various ailments. The challenges and controversies presented reveal areas that require further research and ethical reflection.
Overall, this summary reinforces the significance of each topic discussed and highlights how integral cell differentiation is to both developmental biology and applied medical research. A comprehensive grasp of these concepts can empower future advances in science and medicine.
Recap of Key Points
- Definition: Cell differentiation transforms unspecialized cells into specialized types with unique functions.
- Genetic Regulation: Gene expression, influenced by transcription factors, directs differentiation.
- Signaling Pathways: External and internal signals activate cellular responses crucial for differentiation.
- Epigenetics: Modifications affecting gene expression can lead to diverse outcomes without altering DNA.
- Health and Disease: Understanding differentiation is fundamental for addressing various medical conditions.
- Future Directions: Advancements in technologies and their applications can change regenerative medicine.
Concluding Remarks
The exploration of cell differentiation presented in this article underscores its critical role in development and health. As scientific methods evolve, so does the potential for insightful findings in the realm of differentiation. Future research is not only imperative for deepening our understanding but also essential for translating this knowledge into practical applications in medical sciences.
By comprehensively examining the mechanisms and implications of cell differentiation, we lay the groundwork for innovative solutions to medical challenges. The dialogue around differentiation invites continuous inquiry, ensuring that it remains a dynamic area of research with profound relevance to biology and beyond.