Understanding Glycans: Structure, Function, and Significance
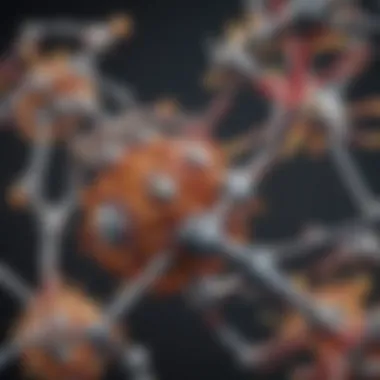
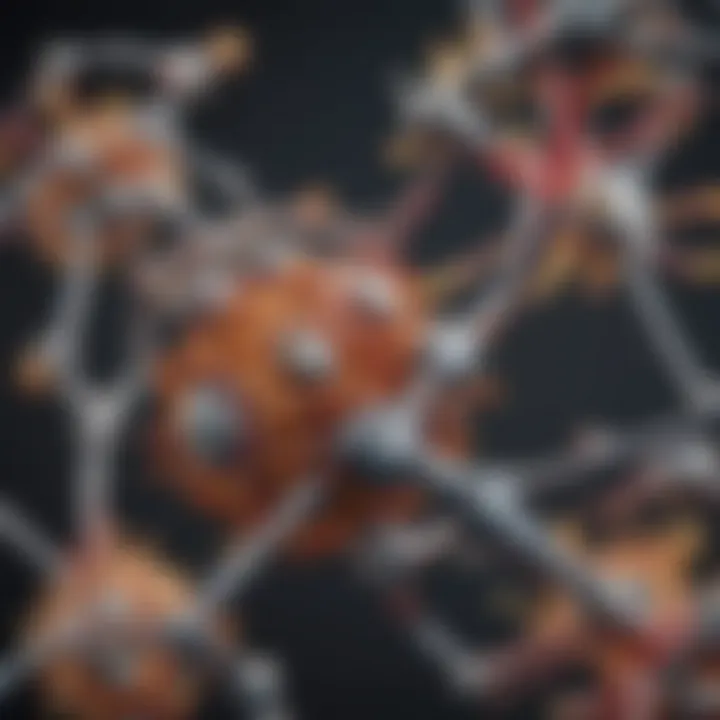
Intro
Glycans represent a fascinating and often underappreciated element of biochemistry. These complex carbohydrates have pivotal roles in various biological functions and disease mechanisms. In recent years, the interest in glycans has surged, primarily due to their implications in cell signaling, immune responses, and even in the development of certain diseases. Their structures are not only diverse but also intricate, making them a significant area of study in both biology and medicine.
Understanding glycans involves delving into their chemical composition, biosynthesis, and how they interact with proteins and lipids. This knowledge proves crucial for developing targeted therapies and diagnostic tools. As we explore glycans in depth, we will cover key findings, methodologies involved in their study, and their broader implications for health and disease.
The research conducted around glycans has unraveled layers of complexity that allow us to appreciate their significance more fully. Through this exploration, we aim to bridge the gap between sophisticated research and practical application in medicine and biotechnology.
Preface to Glycans
Glycans are essential components in the field of biochemistry and molecular biology. Understanding them provides deeper insights into various biological processes. As complex carbohydrates, glycans possess unique structures and functions that significantly contribute to biological interactions. The significance of this topic lies in its broad applicability, ranging from cellular communication to implications in health and disease.
Definition of Glycans
Glycans are polysaccharides composed of sugar molecules. These sugar units, known as monosaccharides, link together to form larger structures. Common monosaccharides include glucose, galactose, and mannose. When these units bond, they can create disaccharides, oligosaccharides, and polysaccharides. Glycans can differ drastically in structure and complexity, making them versatile in their biological roles.
Importance in Biology
Glycans are not merely energy sources; they are fundamental in many biological processes. Here are some roles they play in biology:
- Cell Signaling: Glycans participate in cell signaling, influencing various physiological responses.
- Immune Function: They are crucial for immune interactions, aiding recognition of pathogens and modulating immune responses.
- Development: Glycans facilitate cell-cell interactions during development, guiding proper formation of tissues.
- Disease Mechanisms: Alterations in glycan structures can relate to disease states, including cancer and autoimmune disorders.
In summary, glycans are vital for maintaining health and understanding disease mechanisms. Their diverse roles and interactions highlight the need for further research in this area.
Structure of Glycans
Glycans are complex carbohydrates, and their structure is influential in determining their function and behavior in biological systems. The structure of glycans is multifaceted, composed of various building blocks called monosaccharides. These fundamental units connect through specific linkages to form larger carbohydrate molecules. Understanding the structure is paramount because it lays the foundation for how glycans participate in biochemical processes and interactions within cells. Additionally, structural variations lead to distinctly different characteristics and roles in health and disease.
The importance of glycan structure is highlighted in how they influence biological recognition events, such as cell signaling and immune responses. Each glycan type can possess unique structural features that facilitate specific interactions with proteins, lipids, and other macromolecules. Therefore, elucidating the structure of glycans contributes significantly to our knowledge of cellular mechanisms and potential therapeutic strategies.
Monosaccharides
Monosaccharides are the simplest form of carbohydrates and are fundamental to glycan structure. They serve as the building blocks for larger carbohydrate molecules, including disaccharides, oligosaccharides, and polysaccharides.
Types of Monosaccharides
Monosaccharides can be classified based on the number of carbon atoms they contain. Common types include:
- Trioses (3 carbons)
- Tetroses (4 carbons)
- Pentoses (5 carbons)
- Hexoses (6 carbons)
The key characteristic of monosaccharides is their functional groups. These groups allow them to participate in various chemical reactions, making them versatile components in carbohydrate assembly. For instance, glucose, a hexose, plays an essential role in energy metabolism.
A unique feature of monosaccharides is their ability to exist in linear or cyclic forms. This structural flexibility is significant as it can influence how these sugars interact with enzymes and other molecules, providing adaptability within biological contexts.
Properties of Monosaccharides
The properties of monosaccharides relate back to their structure, particularly their solubility and reactivity. Monosaccharides are typically soluble in water due to their hydroxyl groups that can form hydrogen bonds with water molecules. This solubility is vital for their function as biochemical intermediates and substrates.
Their key characteristic includes the ability to undergo oxidation and reduction reactions, influencing metabolic processes. For example, the oxidation of glucose results in energy production through glycolysis.
A unique aspect is that monosaccharides can undergo isomerization, causing variations in function and interaction. This versatility is advantageous as different sugars can fulfill specific roles in various biological pathways.
Disaccharides and Oligosaccharides
Disaccharides and oligosaccharides are formed from the combination of monosaccharides through glycosidic bonds.
Formation of Disaccharides
Disaccharides are formed when two monosaccharides undergo a condensation reaction, releasing a water molecule. This process creates an important subclass of glycans, including sucrose and lactose.
The key characteristic of disaccharides is their role in energy provision and storage in organisms. They participate in many biological functions, including serving as substrates for enzymes.
A unique feature of disaccharides is their ability to be hydrolyzed back into monosaccharides when needed, showcasing their role in efficiently managing energy resources within the cell.
Role of Oligosaccharides
Oligosaccharides consist of a small number of monosaccharide units, typically ranging from three to ten. They can have significant biochemical roles, particularly in cell recognition and signaling.
The key characteristic of oligosaccharides is their diversity in structure. This diversity can lead to distinct functions, such as recognizing specific cellular markers.
A unique feature of oligosaccharides is their involvement in glycoproteins and glycolipids, influencing their stability and functionality. Therefore, understanding these roles is crucial for applications in immunology and cellular biology.
Polysaccharides
Polysaccharides are the largest carbohydrates, consisting of long chains of monosaccharides linked through glycosidic bonds. Their structure dramatically impacts their biological functions.
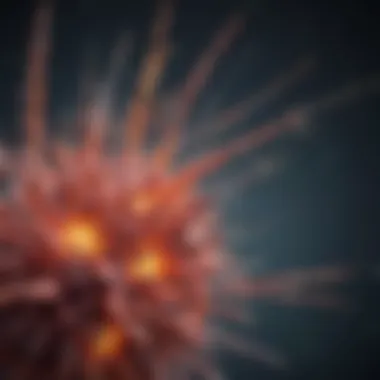
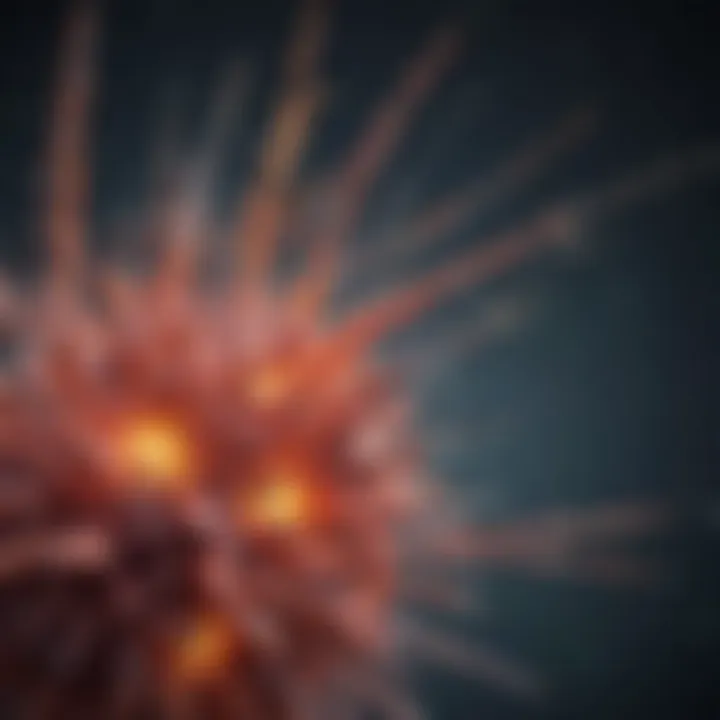
Structural Polysaccharides
Structural polysaccharides provide support and shape to organisms. Cellulose, found in plant cell walls, is an exemplary structural polysaccharide, offering rigidity due to its extensive hydrogen bonding between chains.
The key characteristic of structural polysaccharides is their insolubility in water, facilitating their function as structural materials.
A unique feature of these polysaccharides is their ability to form complex architectures, such as fibers, which are critical in plant anatomy, contributing to overall organismal strength.
Storage Polysaccharides
Storage polysaccharides, such as starch in plants and glycogen in animals, are essential for energy storage. They are formed by the polymerization of glucose and can be broken down when energy is needed.
The key characteristic of storage polysaccharides is the way they can vary in structure. For instance, starch consists of both amylose and amylopectin, affecting its digestibility and energy release.
A unique feature is their capacity to aggregate, forming granules within cells, ensuring that energy can be mobilized rapidly during periods of demand. Understanding storage polysaccharides' structure is crucial for insights into metabolism and energy management in organisms.
Biosynthesis of Glycans
The biosynthesis of glycans is a critical area of study in glycobiology due to its fundamental roles in various biological processes. Glycans, though structurally complex, are assembled through a series of intricate biochemical pathways known as glycosylation. Understanding these pathways is essential for grasping how glycans impact cell signaling, immune response, and other essential functions within the body.
Glycosylation Mechanisms
Glycosylation is the biochemical process by which glycans are attached to proteins and lipids. This mechanism can occur in different ways, primarily classified into N-linked and O-linked glycosylation. Each type has distinct implications for glycan structure and function.
- N-linked glycosylation occurs when the glycan is attached to the nitrogen atom of asparagine side chains in a protein. This type is prevalent in glycoproteins and is crucial for proper protein folding and stability.
- O-linked glycosylation involves the attachment of glycans to the hydroxyl group of serine or threonine residues. This type often plays a role in signaling pathways and cell recognition.
Glycosylation can also be influenced by various factors, such as the availability of nucleotide sugar precursors and the activity of glycosyltransferases, the enzymes that catalyze the addition of sugar residues. The complexity of glycosylation mechanisms underlines the need for a deeper examination of how these processes select specific sugars for attachment and what influences their variation in different cell types.
Enzymes Involved in Glycan Assembly
The biosynthesis of glycans relies heavily on specific enzymes that facilitate the addition and modification of sugar molecules. Some important enzymes involved include:
- Glycosyltransferases: These enzymes are responsible for forming glycosidic bonds, linking monosaccharides in various configurations. Their activity dictates the structure of the resulting glycan.
- Glycosidases: Also known as exoglycosidases, these enzymes cleave glycosidic bonds, removing sugar units from glycan chains. They play roles in remodeling glycans post-synthesis.
- Nucleotide sugar transporters: These transport proteins are crucial for supplying the necessary nucleotide sugars into the Golgi apparatus and endoplasmic reticulum, where glycosylation predominantly occurs.
The regulation of these enzymes is essential. Changes in enzyme activity can lead to significant shifts in glycan structures, which can directly affect biological function. Understanding these enzymatic interactions is key in exploring therapies for diseases where glycan structures are altered, such as in cancer or autoimmune disorders.
"The proper assembly of glycans is not just a matter of structural integrity; it is directly linked to the functional output of numerous biological systems."
In summary, the biosynthesis of glycans through glycosylation mechanisms and the roles of specific enzymes highlights a complex but necessary process critical to life. This area of study promises to illuminate potential therapeutic avenues and deepen our understanding of cellular functions.
Classification of Glycans
The classification of glycans is essential for understanding their diverse roles in biological systems. Glycans can be organized based on their structure and the type of linkage. This system aids researchers in comprehending how variations in glycan structures can lead to different biological functions. By knowing the classifications, scientists can predict the behavior of glycans in various physiological and pathological contexts. This understanding also facilitates advancements in therapeutic and diagnostic applications.
N-linked Glycans
N-linked glycans are attached to proteins through the nitrogen atom of asparagine residues. This form of glycosylation is vital for protein folding, stability, and functional activity. N-linked glycans are often complex and can be branched, which contributes to their immense variability.
Characteristics of N-linked Glycans:
- Biosynthesis: Formed in the endoplasmic reticulum and modified in the Golgi apparatus.
- Functions: Play roles in cell recognition, signaling, and modulation of protein activity.
- Examples: Often found on glycoproteins like antibodies and hormones.
The structural diversity of N-linked glycans is crucial for various biological interactions, influencing processes such as immune recognition and pathogen adhesion.
O-linked Glycans
O-linked glycans are linked to proteins via the oxygen atom of serine or threonine residues. They are typically shorter than N-linked glycans and can consist of various monosaccharides. Their formation mainly occurs in the Golgi apparatus and can involve a range of glycosyltransferases.
Characteristics of O-linked Glycans:
- Simplicity: Generally consist of one or a few sugar residues.
- Functions: Involved in cell signaling, modulating protein interactions, and influencing protein stability.
- Examples: Notably found in mucins, which are critical for protecting epithelial surfaces.
The flexibility in the structure of O-linked glycans allows for diverse interactions with other biomolecules, highlighting their significance in cellular communication.
Sulfated Glycans
Sulfated glycans are characterized by the addition of sulfate groups to their sugar units. This modification alters their charge and can significantly enhance their biological activities. They participate in various processes, including cell signaling and interaction with extracellular matrix components.
Characteristics of Sulfated Glycans:
- Biological Roles: Involved in anticoagulation, growth factor signaling, and cell adhesion.
- Significance: Their negative charge allows for strong interactions with positively charged proteins, influencing numerous cellular processes.
- Examples: Found in heparin and chondroitin sulfate, both known for their therapeutic applications.
The presence of sulfate groups on glycans provides unique functional properties, making sulfated glycans vital players in health and disease.
Functions of Glycans
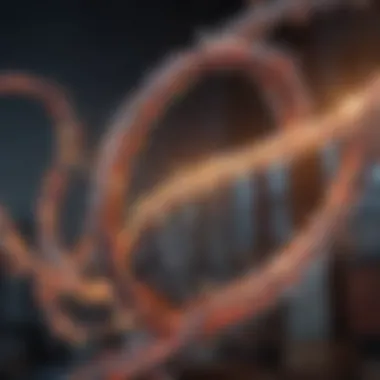
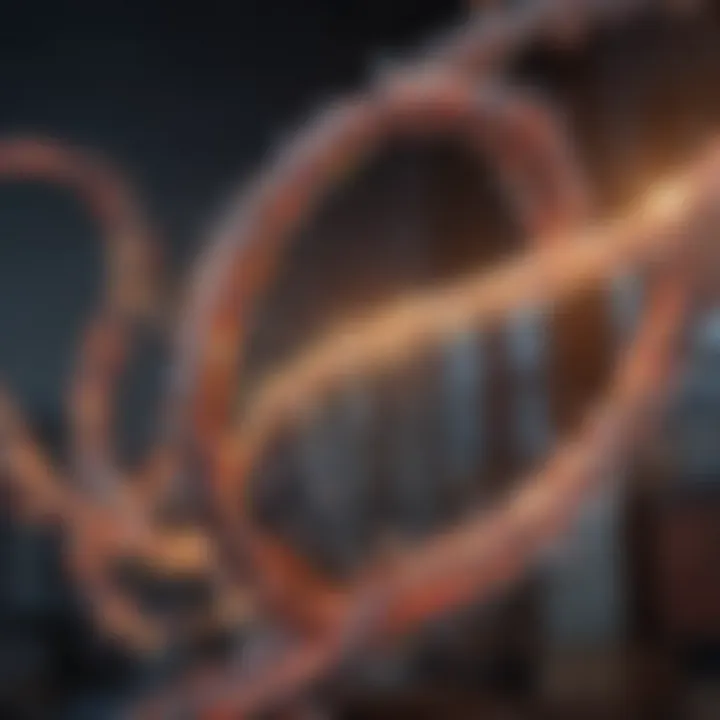
Glycans are not merely structural elements; they play vital roles in numerous biological functions. Understanding these roles is essential for grasping the full impact of glycans on health and disease. Glycans influence cell signaling, contribute to immune responses, and facilitate cell-cell interactions. This section delves into these functions, illustrating the significance of glycans in biological systems.
Cell Signaling
Glycans are integral to cell signaling pathways. They often modify proteins and lipids, impacting how cells communicate with one another. Glycoproteins and glycolipids possess carbohydrate moieties that can mediate binding interactions. This binding can trigger signaling cascades that influence cell growth, differentiation, and apoptosis. For example, the binding of specific glycans to receptors can initiate pathways that lead to immune activation or inhibition.
Moreover, alterations in glycan structures can affect signaling efficiency. Cancer cells often exhibit abnormal glycan patterns, which can lead to dysregulated signaling. Thus, understanding how glycans influence these pathways offers insight into tumorigenesis and other disorders.
Immune Response
Glycans are also pivotal in the immune system. They can modulate immune responses through interactions with various receptors on immune cells. These interactions determine how well the body can recognize and respond to pathogens. For example, pathogen-associated molecular patterns (PAMPs) often contain glycan structures that immune cells identify, allowing for effective immune responses.
The role of glycans in determining self versus non-self is noticeable in autoimmune diseases. Changes in glycan expression can confuse the immune system, leading to attacks on the body's own cells. Thus, comprehending glycans is important for developing therapies for autoimmune conditions.
Cell-Cell Interaction
Glycans are crucial for cell-cell interaction, influencing how cells adhere to one another. This adhesion is vital for tissue formation and stability. Glycans on the surface of cells can interact with counterpart glycans, promoting or inhibiting adhesion depending on the structural features.
For instance, during embryonic development, proper glycan-mediated cell adhesion is necessary for forming tissues. Disruption in these interactions can lead to developmental abnormalities or contribute to the metastatic spread of cancer cells.
In summary, the functions of glycans are multifaceted and impactful in various biological processes. They influence cell signaling, shape immune responses, and facilitate cell interactions, playing critical roles in both health and disease.
Glycans in Health and Disease
Glycans are not merely structural components within biological systems; they are pivotal in health outcomes and disease pathogenesis. Understanding their roles enables researchers to explore new therapeutic avenues. Specifically, glycans can influence cellular interactions, immune responses, and the development of various diseases, including cancer and autoimmune disorders. This section will elaborate on how glycans impact health and disease, emphasizing their diverse functions.
Role in Cancer
In cancer biology, glycans play a complex role in tumor development and progression. They influence cancer cell behavior by modulating cell signaling pathways. For instance, altered glycosylation patterns can enable cancer cells to evade immune detection. This immune evasion is a significant challenge in cancer treatment, as it allows tumors to grow unchecked.
Glycans can also affect cell adhesion, which is crucial for metastasis. When cancer cells lose normal glycosylation, they may become more mobile, facilitating the spread to other tissues.
Moreover, specific glycans serve as biomarkers in cancer diagnostics. For example, the presence of certain sialylated glycan structures can indicate the progression of various malignancies. Investigating these glycan changes can lead to improved cancer detection and targeted treatments.
Glycans in Infectious Diseases
Infectious diseases strongly interact with glycans, particularly at the interface of pathogen-host dynamics. Many pathogens exploit host glycan structures for invasion. For instance, the influenza virus recognizes specific sialic acids on glycoproteins to enter host cells. Similarly, bacteria such as Helicobacter pylori can bind to glycan receptors, facilitating colonization and infection.
On the host side, glycans play a role in immune responses against infections. They contribute to the recognition of pathogens by immune cells, enhancing the efficiency of the immune response. Understanding how glycans are involved in these processes can provide valuable insights into developing vaccines and therapies for infectious diseases.
Impact on Autoimmunity
Autoimmune diseases arise when the immune system mistakenly targets the body’s own cells. Glycans are crucial in moderating immune responses, and any alterations in glycosylation patterns can lead to inappropriate immune activation. For instance, changes in glycan structures on self-antigens can cause the immune system to recognize them as foreign, leading to diseases such as systemic lupus erythematosus or rheumatoid arthritis.
Notably, specific glycan types have been implicated in regulating inflammation and immune signaling. An imbalance in glycan expression may result in overstimulation of the immune system, contributing to autoimmunity. Researchers are now focusing on glycan-targeted therapies to restore normal immune function, offering a promising direction for treating autoimmune diseases.
"Understanding glycans offers a fresh perspective on health and disease mechanisms, potentially leading to novel therapeutic strategies."
Analytical Techniques for Glycan Study
Glycans are essential biomolecules that are intricate and diverse. Their study requires precise analytical techniques to gain insights into their structure and function. The exploration of these techniques is vital to understand glycans' biological roles and to translate that knowledge into applications in biotechnology and medicine. In this section, we will delve into three primary methods: Mass Spectrometry, High-Performance Liquid Chromatography, and Nuclear Magnetic Resonance Spectroscopy. These methods equip researchers with the tools necessary to dissect the complexities of glycans.
Mass Spectrometry
Mass Spectrometry (MS) is a powerful analytical technique that allows for the detailed characterization of glycans. It measures the mass-to-charge ratio of ionized molecules. When it comes to glycans, MS provides insights into their composition, structure, and fragmentation patterns. This information is crucial for understanding glycan identity and heterogeneity.
There are several types of mass spectrometry methods, including:
- Matrix-Assisted Laser Desorption/Ionization (MALDI)
- Electrospray Ionization (ESI)
Both methods have unique advantages. For instance, MALDI is typically employed when analyzing complex mixtures, offering high sensitivity and resolving power. Conversely, ESI is advantageous for studying larger glycans and their interactions with proteins. As a result, the choice of method depends on the specific goals and characteristics of the glycans being analyzed.
High-Performance Liquid Chromatography
High-Performance Liquid Chromatography (HPLC) plays a critical role in glycan analysis. HPLC enables the separation of glycans based on their size, charge, or hydrophobicity. By targeting specific characteristics, researchers can isolate glycans for further studies.
Advantages of HPLC include:
- High resolution and sensitivity
- Ability to analyze complex biological samples
One common application of HPLC in glycan research is in the analysis of glycoproteins. Researchers often exploit HPLC to separate glycans attached to proteins, enabling them to investigate glycan-protein interactions in biological processes. The integration of HPLC with mass spectrometry further enhances the precision of glycan analysis, providing a multi-dimensional approach to understanding glycans at a deeper level.
Nuclear Magnetic Resonance Spectroscopy
Nuclear Magnetic Resonance Spectroscopy (NMR) is another invaluable technique for glycan study. NMR provides high-resolution structural information about glycan molecules. It allows for the investigation of glycan conformation, linking positions, and types of sugar units.
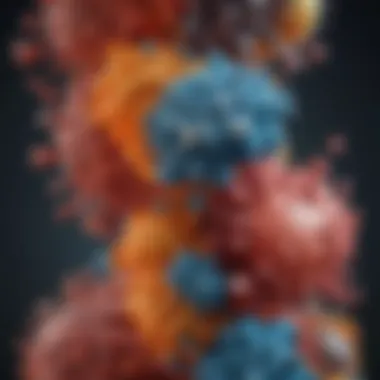
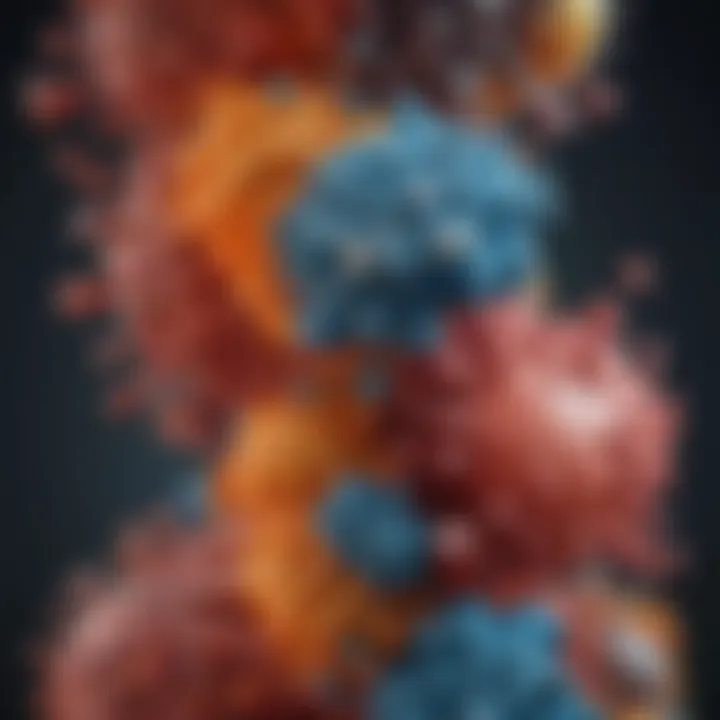
Key benefits of NMR include:
- Non-destructive analysis,
- Ability to analyze in solution,
This richness in data makes NMR particularly suitable for studying dynamic aspects of glycans. Researchers can observe conformational changes in glycans in real time, which is crucial for understanding interactions with biological macromolecules like proteins. The data obtained from NMR can complement findings from mass spectrometry and HPLC, leading to a more comprehensive understanding of glycan functionalities.
Applications of Glycans in Biotechnology
The applications of glycans in biotechnology are numerous and increasingly important, spanning therapeutic, vaccine development, and diagnostic uses. These complex carbohydrates provide unique functionalities that can enhance biological systems, making them critical in the formulation of new medical treatments and diagnostic techniques. The study of glycans has gained momentum in recent years due to their potential to address various health challenges and improve therapeutic outcomes.
Therapeutic Uses
Glycans are critical in developing therapeutic agents, particularly in the realm of biopharmaceuticals. These complex carbohydrates can improve the stability and efficacy of drugs, especially biologics such as antibodies. For example, glycosylation of therapeutic proteins can significantly influence their half-life and immunogenicity. By modifying glycan structures, researchers can tailor these molecules for specific clinical needs. Moreover, glycans play a key role in targeting delivery systems; they can facilitate the cellular uptake of drugs, ensuring the therapeutic agents reach their intended sites more efficiently.
"Proper glycan modification can mean the difference between an effective treatment and one that fails to elicit a desired response."
- Example Uses in Therapy:
- Antibody Formulation: Enhancing stability and reducing immunogenic responses.
- Enzyme Replacement Therapy: Using modified glycans to improve enzyme targeting and distribution.
- Cancer Treatments: Targeted therapies exploiting glycan interactions with specific receptors on cancer cells.
Vaccine Development
Glycans are also vital in vaccine development. By understanding glycan structures on pathogens, biotechnology firms can design more effective vaccines. Glycans can be used as adjuvants, substances that increase the immune response to vaccines. This strategic use helps to ensure that vaccines provoke a stronger and longer-lasting immune reaction. For instance, the design of glycoconjugate vaccines combines polysaccharide antigens with protein carriers, markedly improving their immunogenicity.
- Key Aspects of Glycan Utilization in Vaccines:
- Enhanced Immune Response: Glycans linked to proteins can lead to more robust antibody development.
- Targeting Specific Pathogens: Glycan compositions can be tailored to combat specific diseases, such as bacterial or viral infections.
- Broad Spectrum Applications: They enable the development of vaccines with the potential to protect against diverse strains of infectious agents.
Diagnostic Tools
The role of glycans in diagnostics is gaining prominence as well. Glycan profiling is emerging as a powerful tool for diagnosing diseases. Since glycan structures can change in disease states, analyzing these changes can provide insights into an individual's health status. Glycomic approaches can thus aid in identifying biomarkers for conditions such as diabetes, cancer, and autoimmune disorders.
- Applications in Diagnostics:
- Biomarker Identification: Altered glycan patterns can serve as indicators of disease progression or response to therapy.
- Disease Classification: Understanding specific glycan signatures can help differentiate between diseases with similar symptoms.
- Therapeutic Monitoring: Changes in glycan patterns can be tracked to monitor the effectiveness of treatments.
Future Directions in Glycan Research
The exploration of glycans is steadily evolving, revealing significant insights into their functions and implications in various biological contexts. Studying the future directions in glycan research is paramount for both theoretical and practical advancements in biology and medicine. As technological capabilities increase, the ability to analyze glycans more precisely enhances our understanding of their roles in cell biology and disease.
Emerging Technologies
Emerging technologies are reshaping glycan research, bringing about more sophisticated methods of analysis and manipulation. The integration of advanced techniques like CRISPR gene editing allows for deeper investigations into glycan biosynthesis. Researchers can now create cell lines with specific alterations in glycan production, facilitating the study of how these changes influence biological processes.
Mass spectrometry continues to be a pivotal tool. In recent years, improvements in this technology have enabled scientists to discern glycan structures with remarkable accuracy. Coupling mass spectrometry with chromatographic techniques results in high-resolution details about glycan composition and structure. Such advancements make it possible to analyze complex glycan mixtures, leading to discoveries that could not have been made with earlier methods.
Additionally, machine learning and artificial intelligence are beginning to play roles in glycan research. These technologies can process vast datasets quickly, identifying patterns and relationships within glycan data that may not be immediately apparent to human researchers. By harnessing these tools, future studies can yield insights that enhance our understanding of glycan functions in biological systems.
Potential Therapeutic Targets
The potential therapeutic targets arising from glycans warrant significant attention. Research shows that specific glycans play crucial roles in disease mechanisms, such as cancer and infectious diseases. For example, variations in glycan structures can affect how cancers progress and spread. By targeting these glycan alterations, therapies could be developed to hinder tumor growth or enhance immune responses against cancer cells.
Infectious diseases also present another avenue for therapeutic exploration. Pathogens often exploit glycans on host cells for entry or evasion of the immune response. Understanding the interactions between pathogen glycans and host cell receptors can lead to novel antiviral therapies that block these interactions.
Furthermore, autoimmune diseases exhibit connections with aberrant glycan structures. Identifying specific glycans that contribute to autoimmune responses could pave the way for targeted therapies that modulate the immune system’s activity.
"Glycans are not merely structural entities; their diverse functions in health and disease underline their potential as therapeutic targets."
In summary, the future directions in glycan research are rich with opportunities. Emerging technologies will not only refine our ability to investigate glycans but also open new pathways for therapies in treating various conditions. By identifying and targeting specific glycan structures, research holds promise for significant advancements in medicine and biotechnology.
Finale
The conclusion of this article highlights the indispensable role that glycans play in a multitude of biological functions and their broader significance in medicine and biotechnology. Glycans are not simply by-products of metabolic processes; rather, they are intricate regulators of cell behavior, which influence cell signaling, immune responses, and interactions between cells. Understanding glycans allows researchers to uncover mechanisms behind various diseases. This knowledge is crucial, especially as the landscape of healthcare continues to evolve toward more personalized approaches.
Moreover, the implications of glycan research extend to numerous fields, including drug development and diagnostics. By elucidating the pathways involving glycans, scientists can identify potential therapeutic targets that could yield significant advancements in treatment strategies for diseases such as cancer and autoimmune disorders.
In summary, the importance of glycans cannot be overstated. They are integral to cellular functions, with the potential to influence health outcomes. As research continues to advance, the understanding of glycans will likely become even more critical, opening new avenues for innovation in both research and practical applications.
Summary of Key Points
- Glycans are complex carbohydrates that serve critical functions in biological systems.
- They play significant roles in cell signaling, immune responses, and cell-cell interactions.
- Glycan biosynthesis and classification reveal the complexity and diversity of these molecules.
- Analytical techniques such as mass spectrometry, high-performance liquid chromatography, and nuclear magnetic resonance spectroscopy are essential for studying glycans.
- The application of glycans in biotechnology demonstrates their potential in therapeutic uses, vaccine development, and diagnostic tools.
- Emerging technologies and therapeutic targets represent exciting future directions in glycan research.
Implications for Future Research
Looking ahead, the implications for future research on glycans are expansive. There will likely be a focus on advancing analytical techniques to improve the characterization of glycans. Better methods of analysis will not only enhance understanding but also allow for the identification of glycan-related biomarkers in various diseases.
Furthermore, understanding the role of glycans in disease mechanisms presents a pathway for developing targeted therapies. Continued investigation is necessary to determine how glycan structures influence their functions in different biological contexts, particularly in the face of disease.
As knowledge in molecular biology progresses, integrating glycan research with genomics and proteomics may provide a more holistic understanding of cellular behavior and disease states. The future of glycan research will likely see collaborations between various scientific disciplines, fostering innovations that can significantly impact healthcare and biotechnology.
"The study of glycans is not just a niche area; it is a vital intersection of biology, medicine, and technology that holds great promise for future discoveries."