Understanding Immobilization: Concepts and Applications
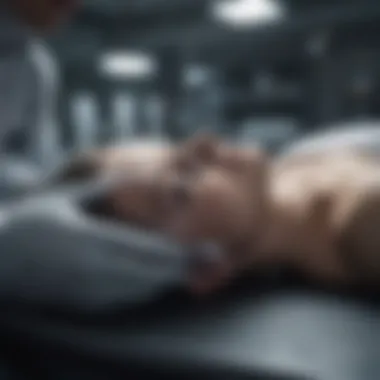
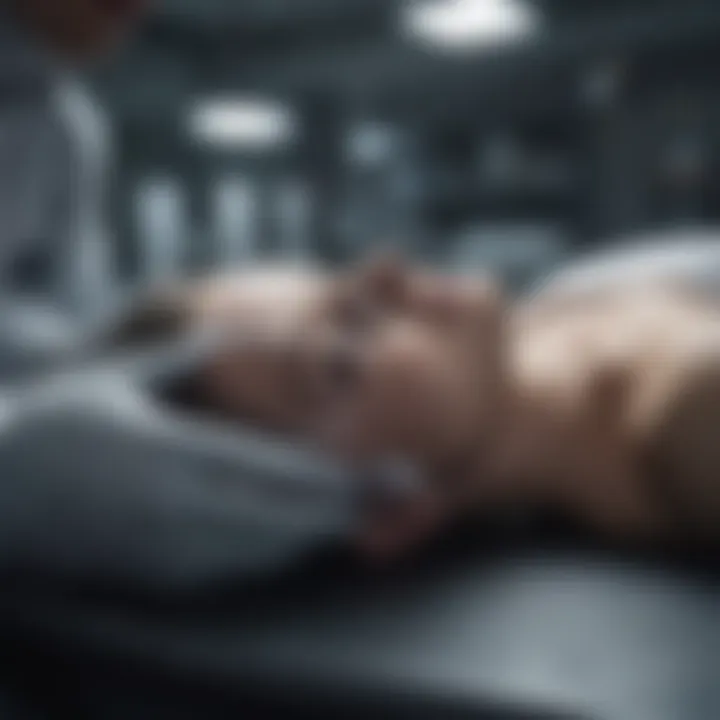
Intro
Immobilization is a concept that spans various scientific disciplines, including biotechnology and pharmaceuticals. It involves the confinement or attachment of biological molecules, such as enzymes or cells, to a solid support, effectively preventing them from moving freely in a solution. This process offers numerous benefits, enhancing stability and facilitating easier handling in various applications. Understanding the mechanisms and implications of immobilization is crucial for researchers, educators, and professionals working in these fields.
Through this exploration, we aim to dissect the complexities of immobilization, shedding light on its various applications and paving the way for innovative approaches in research and practical uses.
"Immobilization is vital for optimizing the performance of enzymes and cells in industrial and research settings."
The significance of immobilization cannot be overstated. It leads to improved efficiency in biochemical reactions and can significantly impact therapeutic developments. By reviewing different immobilization methods, we can appreciate their contributions to enzyme technology and pharmaceutical advancements.
This article will present a structured discussion, beginning with an overview of research findings in immobilization, followed by an examination of methodologies used in related studies. Through this course, it seeks to empower readers with a comprehensive understanding of immobilization, its applications, and the implications it carries for ongoing and future research endeavors.
Defining Immobilization
In the context of scientific research and application, defining immobilization is essentiel. The concept refers to the process of restricting the movement of substances, which is crucial in various fields, such as biotechnology and pharmaceuticals. Immobilization serves multiple purposes, including increasing the stability of enzymes, enhancing the efficiency of biochemical reactions, and providing controlled drug release.
Understanding this concept is important for students, researchers, and professionals. It lays the groundwork for numerous applications in biotechnology and drug development. By engaging with the principles and mechanisms of immobilization, one can appreciate its influence on modern science and technology.
Conceptual Framework
The conceptual framework of immobilization can be divided into several fundamental aspects. First, it often involves physical, chemical, or biological methods to bind substances in a non-mobile state. Each method has distinct characteristics that impact the efficiency and efficacy of immobilized materials.
Key elements include:
- Substrate Interaction: The material to which the enzyme or cell is attached will affect its activity and stability.
- Environment: Factors like pH, temperature, and ionic strength can influence the behavior of immobilized substances.
- Type of Immobilization: Choices between covalent bonding, adsorption, or entrapment strategies are crucial.
A well-designed immobilization strategy must consider these factors to achieve optimum performance in specific applications.
Historical Background
The evolution of immobilization techniques spans several decades, reflecting advances in biochemistry and materials science. Initial approaches were predominantly made in the 20th century, with significant milestones that shaped current practices.
The early days focused on physical adsorption and encapsulation. Gradually, covalent attachment methods gained popularity due to their stability and reusability. The development of materials like silica gels, agarose, and polymers expanded possibilities in immobilization technologies.
Throughout history, advancements in analytical techniques and molecular biology propelled the study of immobilization further. The introduction of recombinant DNA technologies and bioengineering has allowed scientists to explore new avenues in enzyme engineering and drug delivery.
Understanding the historical context of immobilization sheds light on its development and current applications. It also provides a critical basis for ongoing research aiming to refine and innovate immobilization methods.
Mechanisms of Immobilization
Immobilization plays a critical role in various scientific fields, particularly in biotechnology and pharmaceuticals. Understanding the mechanisms of immobilization is fundamental for optimizing processes involving enzymes, cells, and other biological components. It influences efficiency, stability, and overall performance in applications such as drug delivery and bioremediation. This section delves into the different mechanisms of immobilization through physical, chemical, and biological methods. Each method has unique advantages and challenges that influence their usage in specific scenarios.
Physical Methods
Physical methods of immobilization generally take advantage of non-covalent interactions to secure biomolecules in a fixed position. These include methods like adsorption, encapsulation, and confinement within a matrix.
- Adsorption: This involves binding biomolecules onto a support material through weak interactions. Materials like activated carbon and silica gel are frequently used as support matrices. The benefits of adsorption include simplicity and the potential for reusability. However, the major drawback is that the immobilized biomolecule may leach over time.
- Encapsulation: This technique encases biomolecules within polymers or gels. This method can protect sensitive enzymes from environmental factors while allowing substrate access. On the downside, the diffusion of substrates through the encapsulating material can limit efficiency.
- Confinement: Here, the biomolecule is physically immobilized in a microenvironment, restricting its movement while maintaining its functional state. This method often provides a stable environment but may challenge mass transfer.
In summary, physical methods are advantageous for their ease of use and adaptability but can struggle with stability and efficiency.
Chemical Methods
Chemical methods involve forming covalent bonds between the biomolecule and the support material. This results in a more stable attachment, mitigating the risk of leaching:
- Covalent Bonding: This technique uses reactive surface groups to create strong covalent links with biomolecules. For instance, support materials can be functionalized with groups like amines or carboxylic acids. This method enhances stability; however, it may adversely affect the activity of sensitive enzymes by altering their structure.
- Cross-Linking: In this approach, agents such as glutaraldehyde connect biomolecules to one another and to a support structure. Cross-linking can create a robust three-dimensional network, improving durability. However, this may also reduce the available active sites of enzymes.
- Grafting: This process attaches functional groups onto support materials, promoting interaction with biomolecules. Grafting can enhance bonding strength and activity but requires careful handling during the preparation phase to ensure proper attachment.
Overall, chemical methods provide a good balance of stability and activity, though they demand meticulous execution to avoid compromising biomolecule functionality.
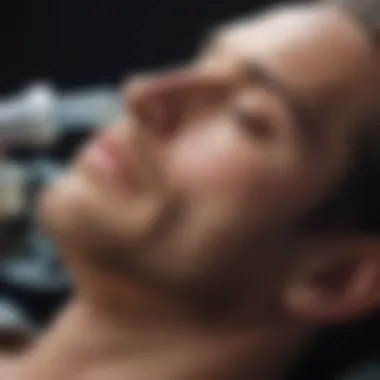
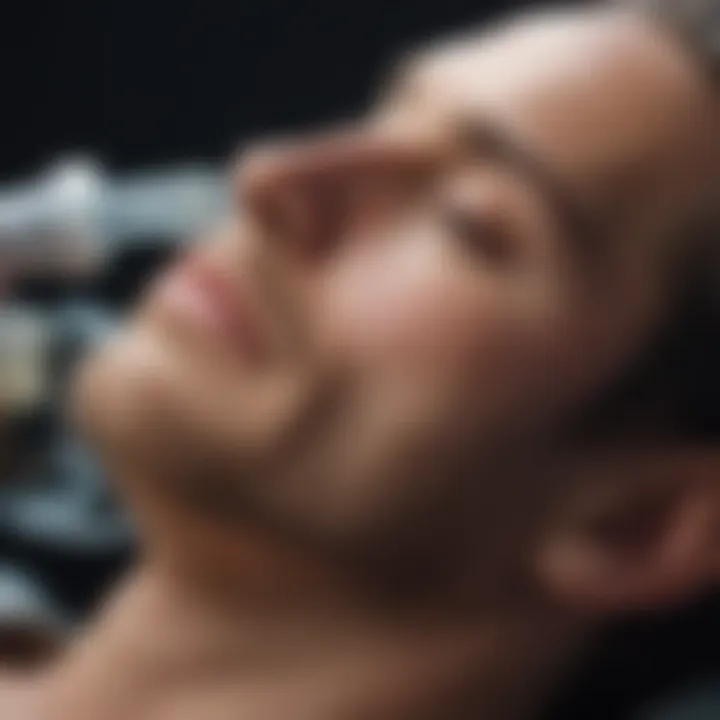
Biological Methods
Biological methods utilize natural processes or bioengineering to achieve immobilization. These methods often align more closely with biological systems and lead to greater compatibility with the intended application:
- Biopolymers: Utilizing materials like alginate and chitosan, biopolymers can encapsulate and stabilize enzymes or cells. They provide a natural environment that can boost activity and stability. Yet, sourcing and processing biopolymers can be challenging and costly.
- Cell Entrapment in Gel Beads: This technique imprisons cells in porous gel beads, allowing them to undergo natural metabolic processes. This application is essential in fermentation processes, though diffusion limitations can affect product yield.
- Enzyme Activation: Different techniques can trigger enzyme action with minimal loss of function. Genetic engineering can enhance enzyme stability and activity, yielding benefits in various applications. However, extensive knowledge of molecular biology and enzymatic functionality is essential for success.
The choice of immobilization technique significantly impacts the overall efficiency and effectiveness of the expected application. Selecting the appropriate method requires careful consideration of the specific requirements and constraints of each situation.
Each mechanism of immobilization presents distinct advantages and challenges. Understanding these mechanisms enables researchers and professionals to select the most suitable approach for their needs, ultimately enhancing the efficacy of biotechnological applications.
Applications in Biotechnology
In the realm of biotechnology, immobilization plays a crucial role. This technique allows for the stabilization and control of biomolecules, which can enhance their performance in various applications. From enzyme reactions to cell culture processes, applications in biotechnology offer multiple benefits. One significant advantage is that immobilized enzymes typically demonstrate higher stability compared to their free counterparts. This stability can lead to longer operational lifetimes and lower costs in industrial processes.
Enzyme Immobilization
Immobilization of enzymes offers a range of methods, advantages, and challenges that are significant for both research and practical applications.
Methods
The methods of enzyme immobilization include physical adsorption, covalent attachment, and entrapment. Each method has unique characteristics that make it suitable for specific applications.
- Physical adsorption is favored for its simplicity and effectiveness. It allows enzymes to remain active while being attached to supports. However, the weak forces involved can lead to desorption over time.
- Covalent attachment, on the other hand, provides a much stronger bond between the enzyme and the support material. This method improves durability but might affect enzyme activity due to alteration of the active site.
- Entrapment involves encapsulating enzymes within a gel or membrane, which can protect them while allowing substrate to pass through. This method, however, can restrict substrate access and lower enzymatic efficiency.
In summary, choosing the right method depends on the specific application and desired outcomes.
Advantages
The advantages of enzyme immobilization are significant in biotechnology. One key characteristic is the increased enzyme stability under various conditions. Immobilized enzymes can withstand extreme temperatures and pH changes better than free enzymes. This ability translates into enhanced performance, which is highly beneficial in industrial applications.
Moreover, they can often be reused multiple times without a significant loss of activity. This reusability can lead to notable cost savings in large-scale processes. Overall, these advantages position enzyme immobilization as a desirable approach in biotechnological applications.
Challenges
Despite its benefits, enzyme immobilization also presents several challenges. One major issue is the potential loss of enzyme activity during the immobilization process. The methods used can sometimes denature enzymes or alter their active sites, leading to reduced efficiency.
Moreover, the costs associated with the initial immobilization process can be substantial, making it less attractive for smaller-scale applications. Finally, optimizing the reaction conditions for immobilized enzymes can be complex, as the dynamics may differ from those of free enzymes. These challenges must be addressed to fully realize the potential of enzyme immobilization in biotechnology.
Cell Immobilization
Cell immobilization provides vital techniques that support various biotechnological applications.
Techniques
There are various techniques used in cell immobilization, including encapsulation, adsorption, and covalent bonding. Each technique has its own merits and limitations.
- Encapsulation allows for the protection of cells while maintaining their viability. It uses materials like alginate or polyacrylamide.
- Adsorption involves attaching cells to surfaces using physical forces. This is simple yet can lead to cell detachment.
- Covalent bonding forms a stable link between the cells and the support, significantly increasing retention, though it may affect cell function.
The choice of technique is determined by the specific needs in a given application, such as fermentation processes or bio-organisms enhancement.
Importance in Fermentation
The importance of cell immobilization in fermentation processes cannot be underestimated. By immobilizing cells, researchers can create more efficient fermentation systems. This technique enhances yield and productivity, as immobilized cells often outpace their free counterparts.
Moreover, the retention of biomass leads to fewer fluctuations in fermentation conditions. This stability allows for more controlled and predictable fermentation processes. The overall increase in efficiency makes cell immobilization a preferred strategy in industrial bioprocesses.
Limitations
Limitations of cell immobilization also exist and must be recognized. One critical consideration is cell viability post-immobilization. The techniques used can sometimes reduce the metabolic activity of cells, which subsequently lowers the efficiency of the process.
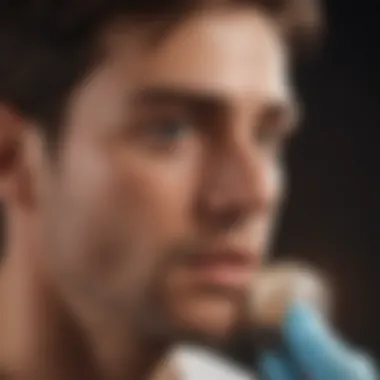
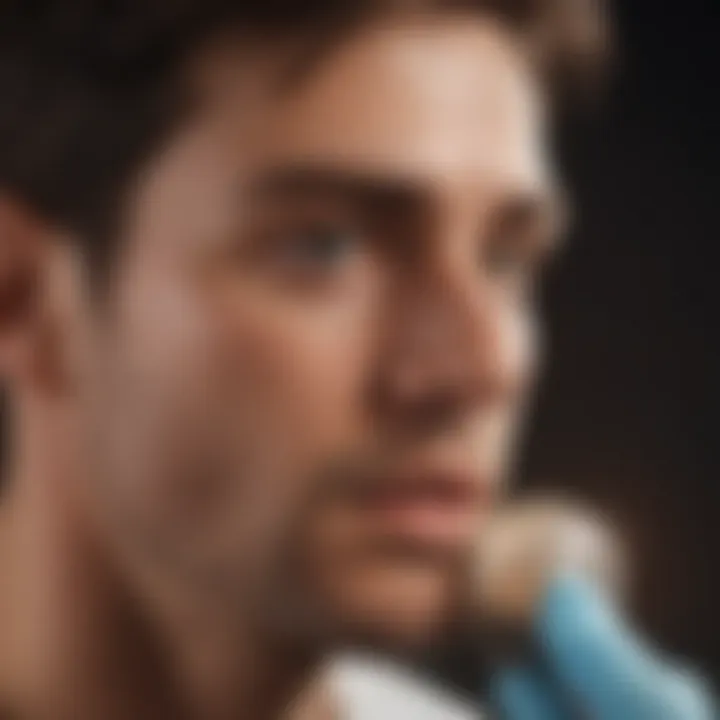
Additionally, mass transfer limitations can arise, making it difficult for nutrients to access immobilized cells. This aspect needs attention, as it may hinder optimal growth conditions. Finally, the costs and complexities involved in developing and maintaining immobilized systems can be a barrier in widespread adoption.
By understanding both the applications and challenges in biotechnology, one can appreciate the nuances of immobilization and its strategic role in advancing scientific and industrial outcomes.
Pharmaceutical Applications
The pharmaceutical applications of immobilization play a pivotal role in enhancing the effectiveness and reliability of various drug development processes. By immobilizing drugs or their carriers, researchers can achieve targeted delivery, improved stability, and controlled release of therapeutic agents. This section delves into two major areas where immobilization is highly significant: drug delivery systems and immunological applications.
Drug Delivery Systems
Drug delivery systems benefit substantially from immobilization techniques. The primary goal of these systems is to ensure that therapeutic agents reach their intended site of action at the right dose and over an appropriate period. Several key advantages of employing immobilization in drug delivery include:
- Targeted Delivery: Immobilization allows for precise localization of drugs, minimizing systemic side effects.
- Controlled Release: This technique enables the gradual release of drugs into the bloodstream, ensuring sustained therapeutic activity over time.
- Stability Enhancement: Through immobilization, the stability of sensitive drugs can be improved, extending their shelf life and effectiveness.
Various methods are utilized for creating these systems, including hydrogels, liposomes, and nanoparticles. Each technique presents unique benefits and challenges. For example, hydrogels can provide a moisture-rich environment that supports drug release, while nanoparticles are advantageous for their small size and large surface area that can improve cellular uptake.
However, careful consideration must be given to the selection of materials and methods for immobilization. The biocompatibility of materials is crucial, as it influences the safety and efficacy of drug delivery systems. Regulatory frameworks also require extensive testing to validate the effectiveness and safety of these systems before clinical approval.
Immunological Applications
In the realm of immunology, immobilization is increasingly recognized for its transformative impact. This technique allows for the development of biomaterials that can interact with the immune system, leading to vaccine development, antibody production, and diagnostic tests. The implications of these applications are profound, as they facilitate advancements in the treatment and prevention of diseases.
Immunological applications using immobilization can enhance the performance of antigens in vaccines. By immobilizing antigens on solid supports, the immune response can be augmented, leading to better efficacy of immunizations. Additionally, this method allows for the production of immunoassays that offer rapid and sensitive detection of pathogens.
The following are potential benefits of immobilization in immunology:
- Increased Stability: Immobilized antigens maintain their structure and function over extended periods.
- Efficient Antibody Production: Immobilized targets facilitate easier interaction with immune cells, promoting quicker antibody response.
- Rapid Testing: Innovative diagnostic tests developed using immobilization techniques can provide swift results, critical in clinical settings.
Environmental Implications
The significance of understanding immobilization extends well beyond laboratory walls and into environmental realms. The adoption of immobilization techniques in environmental science addresses critical challenges like pollution and resource sustainability. This section explores how immobilization plays a crucial role in bioremediation and waste treatment solutions, both of which are pivotal in mitigating the adverse effects of human activities on the ecosystem.
Bioremediation Techniques
Bioremediation involves the use of living organisms to remove or neutralize contaminants from the environment. Immobilization techniques enhance the efficiency of bioremediation by stabilizing microorganisms or enzymes, allowing for continuous and targeted degradation of pollutants.
Key benefits of bioremediation techniques include:
- Increased Specificity: Utilizing immobilized microorganisms ensures that degradation processes target specific pollutants effectively. This precision minimizes collateral damage to other organisms in the ecosystem.
- Sustained Activity: Immobilized cells can maintain their activity over extended periods, reducing the frequency of intervention needed in contaminated sites.
- Cost-Effectiveness: The reusability of immobilized biocatalysts lowers operational costs associated with chemical treatments.
Considerations regarding these techniques involve the choice of support materials for immobilization, as well as the conditions required for optimal activity. Limitations can arise if the environment is not conducive to the immobilized organisms, impacting their functionality and success rates in pollution reduction.
Waste Treatment Solutions
Waste treatment through immobilization techniques provides a methodical approach to manage solid and liquid waste effectively. This process focuses on the immobilization of metabolic-active organisms or enzymes within solid matrices or films, leading to enhanced degradation and detoxification of hazardous materials.
Some notable advantages include:
- Enhanced Removal Rates: Immobilized enzymes or microorganisms can significantly improve the degradation rates of organic substances in wastewater.
- Reduction of Hazardous Byproducts: Certain immobilization approaches minimize the formation of harmful byproducts that conventional treatment methods may produce.
- Adaptability: Various immobilization strategies such as gel entrapment and covalent attachment allow for tailored treatment solutions suited to different types of waste.
However, these solutions require careful consideration of operational parameters, such as pH, temperature, and nutrient availability, to ensure that the immobilized systems remain efficient.
"Effective waste treatment is crucial for sustainable environmental management, as it enables communities to minimize their ecological footprint."
In summary, the environmental implications of immobilization techniques are profound. They stand to significantly enhance bioremediation practices and waste treatment solutions, fostering both ecological and economic benefits. As we continue to face escalating environmental challenges, refining and innovating immobilization strategies will be essential for ensuring a healthier planet.
Comparison of Immobilization Techniques
Comparing various immobilization techniques is crucial in this article. The effectiveness of immobilization depends on the selected method, which impacts the overall performance in applications ranging from biotechnology to environmental sciences. This section will examine both the efficacy of methods and financial implications. By understanding these factors, stakeholders can make informed decisions regarding the choice of immobilization techniques from a technical and economic standpoint.
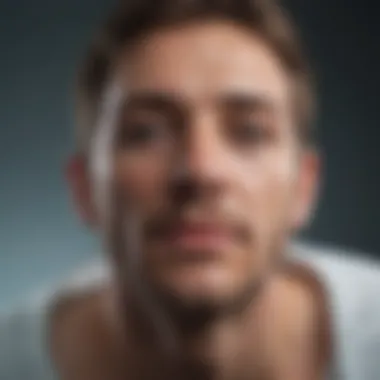
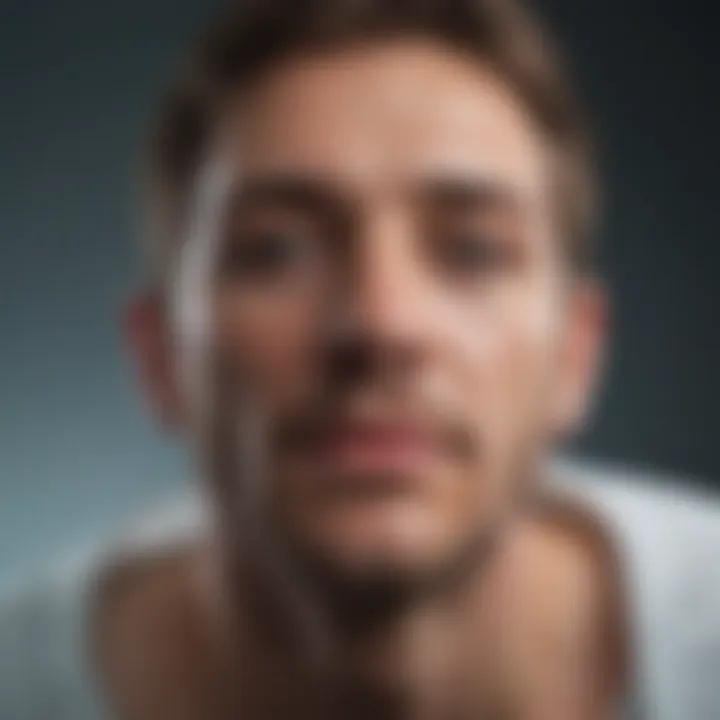
Efficacy Overview
Efficacy pertains to how well each immobilization method achieves its intended purpose. Numerous factors influence efficacy, including the stability of the immobilized product, the activity retention of enzymes or cells, and the ease of reusability. Effective immobilization can enhance reaction rates, increase process efficiency, and improve longevity.
Different methods such as physical adsorption, covalent bonding, and entrapment vary in their efficiency for specific applications. For instance, covalent bonding typically provides greater stability and durability for enzymes, while physical adsorption offers simplicity and rapid application without complicated procedures.
Each technique's efficacy can be summarized in the following aspects:
- Stability: How the immobilized material withstands environmental changes.
- Activity retention: The capability of the enzyme or cell to retain its functional properties.
- Reusability: The potential for repeated uses of the immobilized agent with minimal loss of performance.
- Process control: The ability to manipulate the immobilization process to optimize outcomes.
Cost Analysis
Cost plays a significant role in determining the feasibility of a particular immobilization technique. Expenses include not only the initial investment but also maintenance and operational costs. For many researchers and companies, the economical choice can outweigh the most effective method, especially in large-scale applications.
When analyzing costs, several components must be considered:
- Material expenses: Some methods require specialized materials which can drive up costs. For example, covalent methods might use expensive substrates or linkers that increase initial outlay.
- Labor costs: The complexity and time required for different methods can affect overall labor expenses.
- Scale of deployment: Large-scale applications might benefit from lower per-unit costs, making initial investments more justifiable.
- Efficiency of use: A method that allows for high product yield often compensates for its initial cost through long-term savings.
In summary, while comparing immobilization techniques, it is vital to balance both efficacy and cost. Choosing the right method can significantly influence the success of experiments or commercial processes. In a rapidly advancing field, staying informed about techniques and their financial implications is key to achieving optimal outcomes.
Future Directions
The exploration of future directions in immobilization is essential for advancing various fields such as biotechnology, pharmaceuticals, and environmental science. As scientific challenges grow more complex, understanding potential advancements in this area becomes critical. Innovations in immobilization techniques can provide numerous benefits, including enhanced efficiency, cost-effectiveness, and sustainability in industrial processes.
The importance of focusing on future directions lies in several specific elements. First, the evolution of technology could lead to more effective immobilization strategies that increase the durability and reusability of biocatalysts. Second, addressing existing limitations within current methods can facilitate new applications that were previously unattainable. Finally, this focus can drive research efforts toward exploring innovative materials and methodologies that enhance the overall effectiveness of immobilization.
Innovations in Immobilization Techniques
Recent developments in immobilization techniques are noteworthy. These innovations range from nanotechnology applications to advances in bioconjugation. Nanoparticles, for example, can serve as carriers for enzymes, providing a more stable and efficient immobilization method. Other techniques involve the use of smart polymers that respond to environmental stimuli, allowing for controlled release and activity of immobilized agents.
- Nanoparticles: They allow higher surface area and improved stability.
- Smart Polymers: Can change properties based on external conditions.
- Advanced Surface Modifications: Improve attachment and functionality of enzymes.
The implications of these innovations are significant. By improving the reliability and efficacy of immobilized enzymes or cells, we can enhance their practical applications in industries ranging from food processing to renewable energy.
Potential Research Areas
Identifying potential research areas becomes critical to push forward the boundaries of what immobilization can achieve. Some of the emerging fields that warrant attention include:
- Sustainable Materials: Researching biodegradable and non-toxic materials for immobilization can help reduce environmental impact.
- Artificial Intelligence: Integrating AI in the design of immobilization processes can lead to enhanced efficiencies and optimization strategies.
- Gene Editing Technologies: Exploring how CRISPR and other gene-editing tools can create custom organisms for specific immobilization applications will open new pathways.
As scientists and researchers delve into these areas, significant discoveries may arise. This exploration not only enriches our understanding of immobilization but also creates opportunities for practical applications that can benefit society in health, environment, and technology.
"The continuous evolution of immobilization techniques has the power to transform how we approach various scientific challenges."
Case Studies
Case studies play a crucial role in understanding the practical applications of immobilization. They provide tangible examples of how immobilization techniques are implemented in diverse fields. By examining specific instances, readers gain insights into the methods' efficacy and real-world implications. Case studies illustrate what works effectively within specific contexts and offer lessons for improving future applications. Furthermore, these studies foster a better understanding of both successes and challenges in immobilization. This empirical evidence can guide researchers and professionals toward optimal practices.
Successful Applications
Successful applications of immobilization demonstrate the technique's versatility and effectiveness across various fields. Examples include:
- Enzyme Technology: In industrial applications, immobilized enzymes enhance reaction rates and provide stability. For instance, the use of immobilized glucose isomerase has revolutionized the production of high-fructose corn syrup, enabling cost-effective and efficient processes.
- Bioremediation: In environmental science, immobilized microbial cells aid in degradation of pollutants. For example, Pseudomonas putida has been immobilized on various supports to effectively degrade toluene in contaminated environments.
- Drug Development: In pharmaceuticals, immobilization techniques are employed in drug delivery systems. A notable success is the use of chitosan-coated nanoparticles to deliver chemotherapy drugs. This method allows for targeted delivery, reduces side effects, and enhances therapeutic efficacy.
These examples reflect how immobilization can be tailored to meet the unique needs of different applications, leading to successful outcomes.
Lessons Learned
The examination of case studies also provides valuable lessons that can inform future practices. Key takeaways include:
- Optimization is Key: Many successful applications of immobilization relied on fine-tuning the parameters of the process. Adjusting factors such as pH, temperature, and support type can dramatically impact efficiency.
- Cost-Benefit Analysis: While some techniques may offer superior performance, cost considerations are crucial. For example, large-scale enzyme immobilization often requires balancing efficacy with affordability to ensure competitiveness in the market.
- Handling Variability: Biological systems exhibit variability, making it essential to design robust methods. Researchers must consider potential fluctuations and develop strategies to overcome these challenges.
Lessons from applied case studies guide ongoing research and innovation. They emphasize the need for adaptability and strategic thinking in implementing immobilization across disciplines.
Understanding these lessons aids in designing more effective immobilization strategies in the future, ultimately expanding the applicability and success of these techniques.