Understanding LIBS Spectra: A Deep Dive into Material Analysis
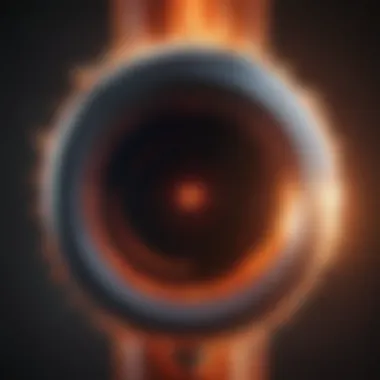
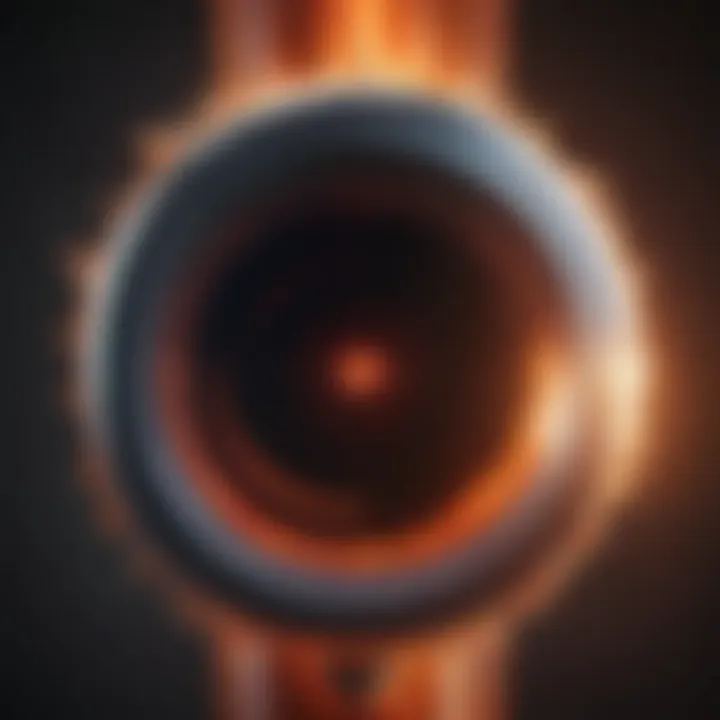
Intro
Laser-Induced Breakdown Spectroscopy (LIBS) has emerged as a highly regarded technique in the realm of material analysis. With roots in plasma physics and spectroscopy, LIBS utilizes a focused laser to ablate a tiny volume of material, creating a plasma that emits light characteristic of the elemental composition of the sample. This process opens up a treasure trove of information for scientists and researchers, enabling them to discern compositions with high precision. Understanding LIBS spectra is not just an academic endeavor—it has real-world applications that stretch across various fields, such as chemistry, geology, and even forensics.
In this article, we aim to peel back the layers of LIBS technology, shedding light on its fundamental principles, methodologies, and transformative potential. The complexities of emission spectra, calibration methods, and recent advancements will be examined, setting the stage for a thorough understanding of how LIBS aids the exploration of elemental analysis. This is crucial for professionals and enthusiasts alike who wish to harness its capabilities in their pursuits.
Research Overview
Summary of key findings
LIBS has made significant strides in its efficacy and scope. Recent studies indicate that the technique can achieve a detection limit in the ppm (parts per million) range for several metals and nonmetals, making it a preferred choice for various applications. For instance, techniques that combine LIBS with complementary methods, such as X-ray fluorescence (XRF), allow for enhanced accuracy and statistical relevance in material characterization.
A notable finding is the adaptability of LIBS in extreme environments. Researchers have successfully employed this technique in challenging settings, such as analyzing samples in remote locations or under varying atmospheric conditions. These studies reinforce LIBS's versatility and reliability as a method for real-time, in-situ analysis.
Importance of the research in its respective field
The impact of LIBS research extends across multiple disciplines. In the field of environmental monitoring, LIBS is increasingly utilized to assess contamination levels in soils and sediments. Similarly, it serves a vital role in metallurgy by providing insights into metal purity or alloy composition. Furthermore, in archaeology, LIBS aids in the analysis of artifacts, contributing valuable data on the materials that composed historical objects.
"The versatility of LIBS positions it as a vital tool for researchers looking to gain rapid insights into material properties, making it highly relevant in today’s scientific landscape."
Methodology
Description of the experimental or analytical methods used
The LIBS technique starts with the generation of a laser pulse, typically using solid-state or fiber lasers. When this laser is aimed at a target sample, it vaporizes a minute portion of that material, forming a plasma cloud. As the plasma cools, it emits light that contains spectral lines corresponding to various elements present in the sample.
This light is captured using a spectrometer, which separates the light into its constituent wavelengths, thereby allowing the identification of elements based on their unique spectral signatures. Advanced data analysis techniques, including chemometrics, are employed to interpret the spectra accurately and isolate individual elemental contributions.
Sampling criteria and data collection techniques
Sampling for LIBS analysis can vary greatly depending on the target application. Typically, solid samples are preferred, but LIBS is also capable of analyzing liquids and gases. A major advantage is the minimal sample preparation required, which sets it apart from other analytical techniques.
Data collection often involves repetitive measurements to enhance statistical significance. The repeatability of readings ensures calibration curves can be established, bolstering the technique's reliability in various conditions. Moreover, laser parameters, such as energy and pulse duration, can be adjusted for different materials to optimize the analysis.
Prelude to LIBS
The world of material analysis is vast and complex, yet it remains vital for advancing various fields, from chemistry to materials science. At the heart of this endeavor is Laser-Induced Breakdown Spectroscopy (LIBS), a technique designed to unlock the secrets hidden in materials through the exciting examination of light emitted from a plasma. Understanding LIBS is not just about grasping the mechanics of the technique, but diving into the profound benefits it offers researchers and professionals alike. The significance of LIBS extends beyond the laboratory; it has applications that touch environmental monitoring and even archaeology.
Historical Context
The origins of LIBS can be traced back to the 1960s when laser technology began to emerge as a promising tool for scientific exploration. Scientists quickly recognized that lasers, when directed at materials, could create a high-energy plasma. This plasma, in turn, emitted light across a spectrum that held valuable information about the elemental composition of the ablated material. Over the decades, as laser technology evolved, so did the applications of LIBS. Early applications primarily focused on the aerospace and defense industries, but its versatility allowed it to flourish in various domains.
In the 1980s, advances in optics and detection technology propelled LIBS into the spotlight. Researchers were able to refine their methods, leading to more accurate and reliable spectral analysis. By the turn of the century, LIBS had established itself as a powerful analytical tool in diverse fields, including metallurgy, geology, and even art conservation. This evolution demonstrates how a simple experimental technique can develop into a core part of scientific inquiry, bridging gaps between fundamental research and practical applications.
Current Applications of LIBS
Today, the landscape of LIBS applications is expansive, reflecting its adaptability and robustness as an analytical method.
- Environmental Monitoring: LIBS is increasingly being utilized in environmental science to detect heavy metals and pollutants in soils and water. This capability is essential for assessing contamination levels and ensuring public health.
- Materials Science and Engineering: In this domain, LIBS plays a critical role in quality control and characterization of materials. It allows for real-time analysis during manufacturing processes, thus ensuring that the final products meet stringent standards.
- Cultural Heritage and Archaeology: Beyond the realms of industry, LIBS is revolutionizing the way scientists analyze artifacts and art pieces without causing any damage. Its non-invasive nature allows for the examination of paintings, pottery, and historical materials, yielding insights into their composition and origins.
By integrating LIBS into these varied contexts, researchers and practitioners can harness its potential to not only perform elemental analysis but also contribute to understanding complex phenomena that shape our world. This multifunctionality is key—it is not only about analyzing materials but also about fostering innovation and enhancing safety across multiple sectors.
Fundamental Principles of LIBS
Understanding the fundamental principles of Laser-Induced Breakdown Spectroscopy (LIBS) establishes a solid foundation for grasping its applications and implications in scientific analysis. These principles not only clarify how LIBS operates but also reveal the underlying mechanisms that allow for precise material characterization. Recognizing the core elements of LIBS enhances the reader's comprehension and opens doors to exploring its potential in various fields, from environmental monitoring to materials science.
Overview of the LIBS Process
LIBS relies on a sequence of well-orchestrated events that transform a focused laser beam into a spectrum of light containing critical information about a material's elemental composition. Let’s break this process into three segments to demystify each aspect.
Laser Ablation
The first step in the LIBS process is laser ablation, where a concentrated laser pulse strikes the surface of a sample. This action removes a minute amount of material, converting it into a vaporized plume. The key characteristic here is that the laser must deliver enough energy to surpass the absorption threshold of the material, resulting in efficient material removal. This makes laser ablation a popular choice in LIBS for its ability to target very small sample areas with precision.
One distinct feature of laser ablation is its adaptability across various materials—be they metals, ceramics, or organic compounds. However, there are disadvantages to note, including the potential for varying ablation efficiencies depending on the sample's surface properties, which may lead to inconsistencies in results.
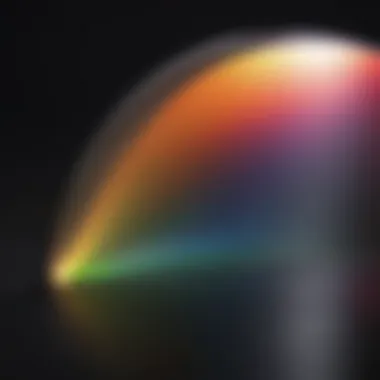
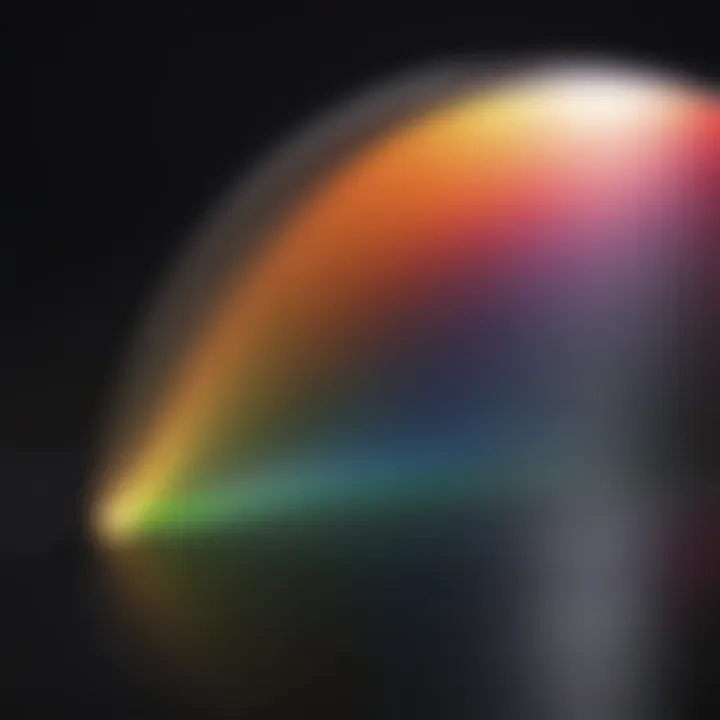
Plasma Formation
Following laser ablation, the vaporized material transforms into plasma, a critical step in the LIBS mechanism. Plasma formation is characterized by high temperatures and ionization, which create a cloud of charged particles. This is significant as it is the environment where elemental analysis occurs. Plasma acts as a beneficial medium, allowing for the disbursement of elements from the sample, thereby making them available for analysis.
A unique aspect of plasma formation is the emission of a broad range of wavelengths, which contributes to the diversity of spectral lines captured later on. However, one disadvantage is that plasma stability can vary, which may complicate data interpretation and require further calibration to ensure accurate readings.
Emission of Light
The final stage in the LIBS process involves the emission of light from the plasma. As atoms in the plasma return to their ground state, they release energy in the form of photons. The emission characteristics are crucial, as they provide the insight needed for spectroscopic analysis. The primary advantages of this aspect are the rapid and simultaneous multi-element analysis capabilities, making LIBS remarkably efficient.
However, it's important to highlight that light emission can be influenced by several factors, like temperature variations within the plasma and surrounding environment, which may affect the resolution or intensity of the detected signals.
Scientific Underpinnings of Emission Spectra
The light produced from the plasma during the LIBS process results in characteristic emission spectra, which are pivotal for material analysis. Delving into the scientific anatomy of these spectra reveals the mechanisms that underpin photon emissions and the significance of atomic structure for accurate interpretation.
Photon Emission Mechanisms
The mechanisms behind photon emissions in LIBS are diverse, primarily involving processes like spontaneous emission and stimulated emission. A significant attribute of photon emission mechanisms is their ability to provide unique spectral fingerprints for different elements, which is advantageous in distinguishing between elements with similar atomic masses. This specificity makes the LIBS method valuable in diverse fields such as chemistry and geology.
Moreover, the spontaneous emission is a unique feature, as it allows for immediate analysis without requiring additional sampling techniques. Yet, as with any method, there are limitations, such as the need for precise calibration to avoid spectral overlap, which could lead to erroneous interpretations.
Role of Atomic Structure
The atomic structure plays a pivotal role in LIBS, as it determines how elements emit light and how spectra appear. The arrangement of electrons around the nucleus impacts the energy levels involved in photon emissions, illuminating the diversity in emitted spectra across different elements. This characteristic renders LIBS a beneficial analytical approach for determining elemental abundance with accuracy.
Each element's unique energetic details can become exceedingly valuable for research and diagnostics. However, it's critical to acknowledge that atomic structure variations, influenced by factors such as bonding and the material's physical state, can complicate spectral interpretation, occasionally limiting the analysis to certain contexts.
By exploring these fundamental principles, one can appreciate LIBS not just as a technique but as a sophisticated method rooted in extensive scientific theory and practical application. As we delve deeper into spectral analysis and calibration techniques, it will become clear how these foundational elements intertwine to support advanced investigation in various domains.
The Spectral Analysis in LIBS
The spectral analysis plays a crucial role in Laser-Induced Breakdown Spectroscopy (LIBS), functioning as the lens through which researchers gain insight into material composition. By understanding the different types of spectra generated, one can unravel the elemental makeup and physical properties of the materials being analyzed. This segment of the article reveals the depth and breadth of spectral analysis, covering important aspects that guide measurements, calibrations, and interpretations.
Understanding Spectra
Line Spectra vs. Continuous Spectra
Line spectra and continuous spectra represent two fundamental types of data that can be gathered in analytical spectroscopy. The former, line spectra, emerges from discrete energy levels of atoms. When a material is vaporized by a laser beam, atoms emit light at specific wavelengths, leading to bright lines on a dark background. This specific characteristic makes line spectra valuable for determining elemental composition, as each element produces a unique set of lines in the spectrum.
In contrast, continuous spectra arise from a broad range of wavelengths, typically produced by solid, liquid, or gaseous substances heated to high temperatures. The choice between line and continuous spectra largely depends on the context of analysis.
- Key Characteristics:
- Line Spectra: Specific peaks corresponding to particular elements signify precise identification of material components.
- Continuous Spectra: A broader range of data offers insights into thermal properties and overall material characteristics.
These distinctions underscore the necessity for both approaches in LIBS, combining them can greatly enhance analytical precision.
Characteristics of LIBS Spectra
The characteristics of LIBS spectra hinge on several factors: the matrix of the sample, the energy of the laser used, and the overall environment in which analysis occurs. A prominent feature of LIBS spectra is the abundance of emission lines superimposed on a potential continuum. This unique blend offers nuanced insights into both elemental composition and physical state of the material being studied.
A vital part to consider is that LIBS can yield a spectrum that is relatively simple for homogeneous samples while being much more complex for heterogeneous ones. Depending on how well the measurement conditions are controlled, the generated spectral information can be both a boon and a bane—too much interference can complicate the analysis.
- Key Characteristics:
- Emission Lines: Clear identification of elements provides vital intelligence about composition.
- Intensity Variation: The intensity of emissions correlates with the concentration of elements, aiding both identification and quantification.
Together, these characteristics help shape a comprehensive understanding of LIBS, enhancing its effectiveness in diverse applications.
Interpretation of LIBS Data
Interpreting LIBS data is perhaps one of the most intricate steps in the analytical process. While the data collected contains vast amounts of information, making sense of it in relation to specific elemental analysis is of paramount importance.
Peak Identification
Peak identification in LIBS data involves discerning the various emission lines that correspond to distinct elements. Each element generates a range of peaks based on its electronic structure. Pinpointing these peaks accurately allows researchers to not only ascertain the presence of elements but also examine their concentrations in mixed samples.
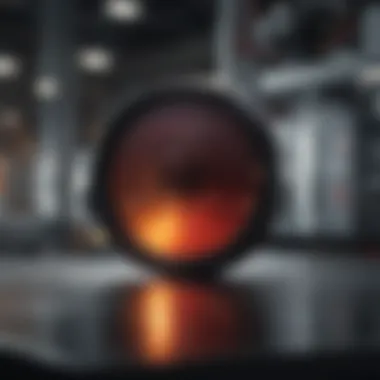
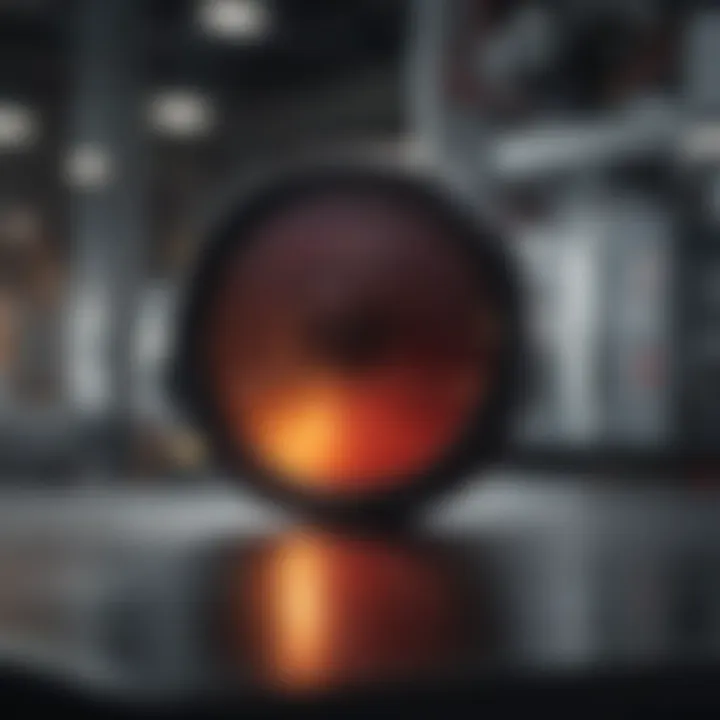
- Key Characteristics:
- Specific Peaks: Identification leads to accurate elemental determination, vital in applications ranging from environmental monitoring to materials science.
- Spectral Overlap: Complex samples may exhibit overlapping peaks, necessitating sophisticated analytical techniques to resolve these issues.
Correct peak identification is paramount. Misinterpretation can skew results and lead to erroneous conclusions.
Quantitative vs. Qualitative Analysis
When analyzing LIBS data, the distinction between quantitative and qualitative analysis is essential. Qualitative analysis identifies what elements are present in a sample, while quantitative analysis goes a step further by quantifying how much of each element exists. Each of these approaches serves a unique purpose; qualitative analysis is typically the first step, offering a broad perspective on sample composition.
- Key Characteristics:
- Quantitative Analysis: Enables precise measurements, indispensable in industrial applications where elemental concentration is critical.
- Qualitative Analysis: Useful for initial assessments, helping to guide further quantitative investigations.
Understanding the interplay between quantitative and qualitative methods enriches the overall interpretation of LIBS spectra, leading to more informed scientific conclusions. > Both forms of analysis are crucial, ensuring that researchers can accurately assess both presence and concentration of elements.
Calibration Techniques for LIBS Spectra
Calibration is central to the reliability and accuracy of Laser-Induced Breakdown Spectroscopy (LIBS) results. Without proper calibration, the data gleaned from LIBS techniques can easily veer off course, leading to misleading interpretations and erroneous conclusions. Therefore, understanding calibration techniques is crucial for researchers and professionals who aspire to utilize LIBS effectively in various applications.
Calibration can be likened to adjusting the tuning of a musical instrument; only when it's spot on, can the true melody of data come forth. It ensures that the emitted light spectra are aligned with the real elemental compositions of the materials analyzed. Proper calibration results in an enhanced signal-to-noise ratio, allowing for improved detection limits and overall accuracy.
Standard Calibration Methods
When it comes to calibrating LIBS systems, several standard methods are employed to ensure that the spectrum readings are not just noise but reflect the true characteristics of the analyzed specimen. Let's break down the most common techniques:
- External Calibration: This involves analyzing known standards that are similar in composition to the samples being tested. By measuring the spectra from these reference materials, a calibration curve can be established. This curve serves as a benchmark against which the unknown samples are compared.
- Internal Standardization: In this approach, a known element is added to the sample before analysis. The intensity ratios of the analyte to the internal standard can provide insights into the concentration of the analyte, making it easier to account for variations in the LIBS system.
- Matrix Matching: Since different materials can affect the spectra differently, ensuring that the calibration standards match the sample matrix is key. This technique involves using standards that mimic the physical and chemical properties of the unknown samples.
- Multiple Calibration Points: Instead of relying on a single calibration point, employing multiple points over the range of interest can yield a more accurate fitting. This method compensates for non-linear responses often seen in spectroscopy.
Each standard method has its own merits and pitfalls. The choice of a calibration technique often depends on the specific requirements of the material being analyzed and the available resources.
Challenges of Calibration
Calibrating LIBS spectra comes with its unique set of hurdles. Addressing these challenges is vital to enhance the credibility of the spectral analyses.
- Surface Effects: One of the intricacies of using LIBS is how the surface condition or the morphology of the sample can influence the results. Variances in surface roughness, contamination, or oxidation can sway the emitted light characteristics, complicating calibration processes.
- Dynamic Plasma Environment: The plasma generated during the LIBS process can vary significantly from one shot to the next. This inconsistency can introduce variability in the emission spectra, complicating the establishment of a stable calibration.
- Non-linear Responses: Some elements exhibit non-linear behavior in their spectral response, making it challenging to create a reliable calibration curve. If not accounted for, this can lead to significant errors in quantification.
- Complexity of Samples: In multi-elemental analyses, deciphering the overlapping spectral lines becomes a struggle. This may create confusion during the calibration process because it’s not always straightforward to separate critical data without advanced analysis techniques.
Tackling these challenges necessitates a thoughtful approach and often, a blend of calibration techniques tailored to specific situations. Thus, it's paramount for practitioners in the field to remain aware of these pitfalls while being adaptable in their methodologies to ensure their LIBS analyses produce trustworthy results.
"In science, errors are a part of the journey to excellence. Calibration, in LIBS, becomes the roadmap navigating these complexities to yield clarity in data interpretation."
Recent Advances in LIBS Technology
The arena of Laser-Induced Breakdown Spectroscopy (LIBS) constantly evolves, driven by technological advancements and innovative methodologies. Understanding these recent trends is crucial, not only for researchers aiming to deepen their knowledge but also for industries that leverage LIBS for material analysis. New developments in this field promise higher accuracy, enhanced sensitivity, and broader applicability, making LIBS an even more valuable tool for scientists and engineers.
Emerging Trends in LIBS
In recent years, we have witnessed remarkable progress in LIBS technologies that have altered the landscape of elemental analysis. For instance:
- Portable Systems: The push towards creating portable LIBS setups has underscored the necessity for on-site analysis in fields such as environmental monitoring and mining exploration. These systems are generally lighter and more user-friendly, which is a game changer when immediate data is crucial.
- Hyperspectral LIBS: An emerging trend involves combining LIBS with hyperspectral imaging. This integration allows for the collection of detailed spectral information across a broader range of wavelengths. As a result, researchers can obtain richer datasets, enabling finer discrimination among elemental compositions in complex materials.
- Artificial Intelligence in Data Analysis: AI's integration into data processing is also gaining traction. By employing machine learning algorithms, researchers can analyze spectral data more efficiently. This reduces the time needed for interpretation and enhances the accuracy of quantitative assessments.
Each of these trends highlights the importance of adaptability in LIBS technology, catering to specific needs across various fields.
Integration with Other Analytical Techniques
Combining LIBS with other analytical techniques enhances its effectiveness, offering a more comprehensive view of material analysis. Such integrations exemplify the versatility of LIBS, further establishing its relevance in scientific inquiry.
LIBS and Mass Spectrometry
The synergy between LIBS and mass spectrometry introduces a powerful analytical duo. When employing mass spectrometry, researchers can gain insight into the elemental and isotopic composition of samples with unparalleled precision. The key characteristic of this integration is its ability to provide complementary information: while LIBS excels in elemental identification through light emission, mass spectrometry brings the advantage of quantifying ionized particles.
This integrated approach is especially beneficial in fields like geology, where accurate mineral compositions are crucial. One unique feature of the LIBS and mass spectrometry combination is that it significantly enhances trace element analysis. The disadvantages may include a steeper learning curve and higher equipment costs, yet the advantages generally outweigh these hurdles due to the breadth of data attained.
LIBS in Combination with Imaging Techniques
Linking LIBS with imaging techniques creates a holistic approach to material analysis. This combination facilitates real-time imaging and elemental analysis simultaneously, which is particularly useful in cultural heritage preservation and forensic science. The distinguishing characteristic of LIBS combined with imaging techniques is the capacity to visualize surface features while also determining chemical compositions.
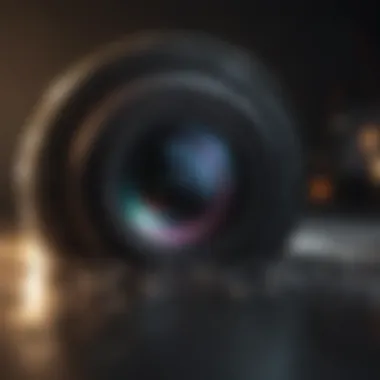
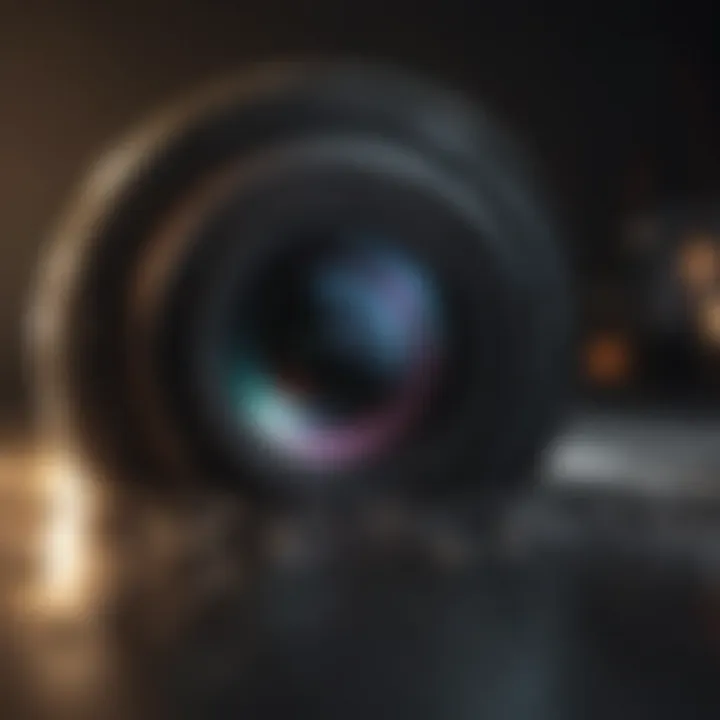
For instance, in the context of cultural heritage, conservators can identify pigment compositions in artwork without damaging the piece. However, the integration often requires sophisticated setups and can complicate data interpretation. The trade-off here is the potential for a richer analytical perspective, much needed in the fields that require depth in both visual and elemental data analysis.
In understanding these advances, we can appreciate how LIBS will continue to evolve, addressing the nuanced needs of researchers and professionals across varied domains.
Applications of LIBS in Different Fields
Laser-Induced Breakdown Spectroscopy (LIBS) stands as a formidable technique bridging gaps across various scientific domains. It has carved a niche, particularly in contexts where rapid analysis is pivotal. The applications of LIBS are vast and equally significant, ranging from environmental monitoring to the intricate scrutiny of artifacts in archaeology. As industries and academia continually seek precise and efficient methodologies for elemental analysis, LIBS emerges as a reliable tool equipped to meet these demands. Below, we explore its crucial roles in three distinct fields.
Environmental Monitoring
In the realm of environmental monitoring, LIBS is like a sharp knife cutting through layers of complexity. The technology plays a critical role in assessing pollution levels, analyzing soil composition, and monitoring water quality. This is where LIBS shines, given that it requires minimal sample preparation and offers real-time results. The speed at which data can be acquired is crucial, especially when tracking potentially hazardous materials.
- Soil Analysis: By examining trace elements in soil, researchers can identify contamination sources or study nutrient availability. This becomes indispensable in agriculture where optimal soil health directly impacts yield.
- Water Quality Assessment: With the rise of concerns over contaminants in drinking water, LIBS allows for the monitoring of heavy metals such as lead and arsenic, providing readings that can be acted upon swiftly.
"The fast and non-destructive nature of LIBS lends itself well to environmental studies, making it a game changer in pollution detection and public health assessments."
Materials Science and Engineering
Moving into materials science and engineering, LIBS is akin to a seasoned detective uncovering the hidden truths of materials. The technique has become instrumental in characterizing materials, assessing their mechanical properties, and ensuring quality in manufacturing processes.
- Characterization of Materials: Researchers utilize LIBS to analyze the composition of metals, polymers, and composites. This allows for tailored material design and performance enhancement, vital in fields like aerospace and automotive engineering.
- Quality Control: On the production line, LIBS assists in rapid testing of materials for impurities. Consequently, it reduces waste and enhances safety, which bears direct implications for product lifecycle management.
For example, in the manufacture of lithium batteries, analyzing the elemental composition can lead to higher efficiency and safety of batteries—factors that determine their success in the market. As the demand for high-performance materials grows, the utility of LIBS in materials science stands to play a pivotal role in advancements across numerous engineering sectors.
Cultural Heritage and Archaeology
In the world of cultural heritage and archaeology, where every artifact tells a story, LIBS acts as a magnifying glass revealing hidden layers of history. By analyzing historical artifacts without damaging them, researchers can unlock secrets about past civilizations and their materials.
- Artifact Analysis: LIBS enables the detailed analysis of pigments, metals, and other materials used in historical artifacts. This not only aids in conservation efforts but also helps in authenticating pieces—an essential factor in the art market.
- Dating Techniques: While traditional methods of dating artifacts often require extensive sampling, LIBS offers an approach that minimizes damage, allowing experts to date finds with a higher degree of confidence.
The marriage of technology and historical inquiry transforms the understanding of artifacts, preserving cultural narratives for future generations. Ultimately, the integration of LIBS in these fields provides both an analytical roadmap and a treasure trove of insights into our past and present.
As LIBS continues to evolve, the potential applications across these varied fields hint at a promising trajectory fueled by innovation and discovery.
Future Directions in LIBS Research
As we stand on the brink of a new era for Laser-Induced Breakdown Spectroscopy (LIBS), it’s crucial to look ahead at what the future holds for this technology. The field has seen significant advancements, but there are still countless areas that warrant exploration and innovation. Understanding these future directions not only helps researchers stay ahead of the curve but also aids in the practical applications of LIBS across various scientific landscapes. Enhancing its effectiveness, versatility, and reach could fundamentally alter how we approach material analysis, making it not just a tool, but a game-changer in numerous sectors.
Research Gaps and Opportunities
Identifying research gaps in LIBS is like finding gold nuggets in a vast mountain; it requires keen insight and persistent exploration. While the technology has made significant progress, there are specific areas that need further attention:
- Sensitivity and Detection Limits: One of the foremost challenges lies in enhancing sensitivity. Many materials can be elusive when it comes to LIBS analysis, especially when present in trace amounts. Innovations that boost detection limits could open up new avenues in fields like environmental monitoring and forensics.
- Matrix Effects: The influence of different materials (or matrix effects) can interfere with the accuracy of LIBS results. Research focusing on overcoming these interferences could lead to more reliable and consistent data, broadening LIBS applications in industries such as metallurgy and pharmaceuticals.
- Method Standardization: The lack of standardized methods for sample preparation and analysis can lead to inconsistencies in results. Developing a set of guidelines could streamline processes and ensure greater trust in LIBS results within the scientific community.
- Algorithm Development: The interpretation of LIBS spectra relies heavily on sophisticated algorithms. There’s a significant opportunity for advancement in data analysis techniques that could enhance the precision of quantitative and qualitative analyses.
By addressing these gaps, researchers can pave the way for better understanding and broader applications of LIBS, effectively enriching scientific knowledge.
Potential Innovations in LIBS Methodology
The potential for innovative methodologies within LIBS is boundless. As technology evolves, so do the possibilities for enhancing LIBS techniques:
- Integration of Artificial Intelligence: Implementing machine learning and AI in LIBS could drastically reshape data processing. These tools could help in real-time analysis, enabling quicker and more accurate assessments of materials under examination.
- Portable LIBS Devices: The development of compact and portable LIBS systems could see wider adoption in field settings, from archaeological digs to remote environmental assessments. This portability would enhance accessibility and facilitate immediate data collection.
- Advanced Laser Techniques: Exploring alternative laser sources or expanding to ultrashort laser pulses can be pivotal. These techniques might improve the energy efficiency of the process while refining the quality of the spectral data generated.
- Hybrid Approaches: Combining LIBS with other techniques such as X-ray fluorescence or induced coupling plasma could yield synergistic effects that capitalize on the strengths of multiple methods, resulting in more holistic analyses.
"The future of LIBS is not just in its current application but in how creatively we can adapt and enhance its methodology to meet emerging scientific challenges."
Closure
The exploration of Laser-Induced Breakdown Spectroscopy, often truncated to LIBS, signifies more than just an analytical technique; it embodies a gateway to understanding material properties at a fundamental level. This conclusion encapsulates the highlights and future prospects discussed in the article, further emphasizing the relevance of LIBS in various scientific and industrial realms.
Summary of Key Insights
Reflecting on the journey through the intricacies of LIBS, several key insights emerge:
- Versatility in Applications: LIBS has proven invaluable across diverse fields— from environmental monitoring to materials science, revolutionizing how we analyze and interpret material compositions.
- Spectral Nuances: A substantial part of this technique lies in the interpretation of emission spectra, which extends beyond mere identification of elements; it also opens avenues for insights into material structures and interactions.
- Calibration Challenges: An understanding of the calibration techniques remains crucial. While standard methods exist, the ongoing battle against variability emphasizes the need for continual improvement and adaptation.
- Emergent Technologies: The integration of LIBS with other methodologies like mass spectrometry represents a cutting-edge advancement that enhances the accuracy and depth of elemental analysis. Such developments signify a shifting tide in analytical capabilities.
These insights not only underscore LIBS's significance as a tool in analytical chemistry but also its potential as a medium for deeper scientific inquiry.
Implications for Future Research
As we peer into the horizon of LIBS research, the implications are profound:
- Innovative Calibration Techniques: Future research could delve into developing advanced calibration methods that mitigate current challenges inherent in the LIBS process. More precise calibrations can enhance the technique's reliability across looser standards.
- New Applications: The exploration of LIBS in sectors not yet fully realized, such as biomedical applications or nano-material characterization, could elicit newfound understanding and discovery.
- Synergistic Methods: Continued investigation into hybrid techniques that combine LIBS with other spectroscopy forms or imaging methods will likely enhance analytical outcomes, resulting in synergistic advantages.
- Machine Learning: Integrating artificial intelligence to analyze LIBS data could refine peak identification and broaden qualitative insights, allowing for automated, refined spectral analysis.
The future beckons with possibilities driven by research and innovation. As we stride forward, the implications of unfolding LIBS methodologies will shape the trajectory of elemental analysis and characterization, cementing its role at the core of scientific inquiry.