Understanding Neurons: Key to Cognition and Behavior
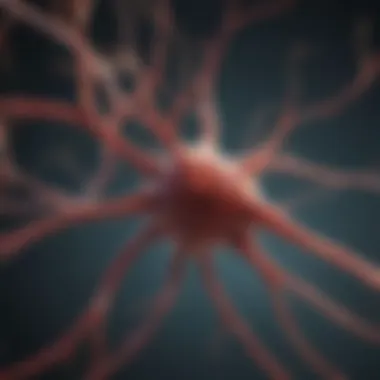
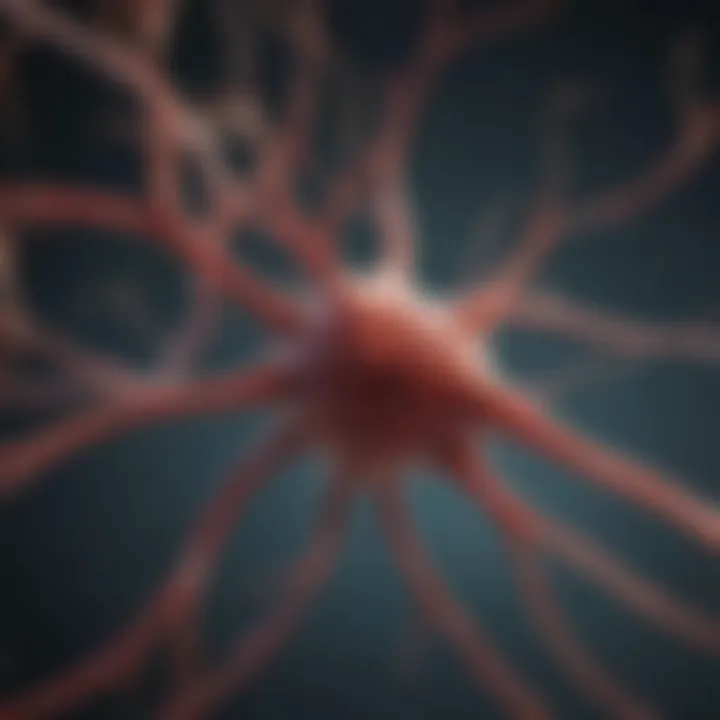
Intro
The human brain is often described as the final frontier of biological exploration. Itās a labyrinth of billions of neurons, each contributing to the symphony of chemicals and electrical impulses that dictate our thoughts, movements, and emotions. Understanding neurons goes beyond mere academic curiosity; it provides insights into our very identity and the way we experience the world.
This intricate web of neural connections forms the basis of everything we know about cognition and behavior. Neurons, the basic units of the brain, can be likened to individual musicians collaborating to create a masterpiece. Without one, the melody falters. Thus, dissecting their roles and interactions sheds light on the essence of human experience.
In this guide, we will embark on an exploration that covers key aspects of neuronal structure and function, the different types of neurons, and the latest in neuroscience research. By delving into this topic, we appreciate not just the complexity of the human brain, but also its incredible capacity for adaptation and resilience.
Research Overview
Neuroscience is a rapidly evolving field. Recent research has uncovered astonishing findings about how neurons communicate. For instance, it's been found that synaptic connections, the junctions where neurons connect, play a pivotal role in learning and memory. By remodeling these connections, the brain adapts, allowing for new information to be processed.
Summary of Key Findings
- Neurons and Plasticity: Neurons exhibit a remarkable ability to change their connections in response to experience, a phenomenon known as neuroplasticity. This is crucial for learning.
- Neuronal Types and Functions: Not all neurons are created equal. There are sensory neurons, motor neurons, and interneurons, each playing specialized roles in the nervous system.
- Health Implications: The structure and function of neurons can be compromised by various factors, including stress and age. This can lead to cognitive decline and various neurological disorders.
Importance of the Research in Its Respective Field
Understanding neurons isn't merely about mapping out biological pathways; it holds significance for mental health treatment, education, and even the development of artificial intelligence. Enhanced insights into neuronal function could lead to innovative therapies for conditions like Alzheimer's or Parkinson's, offering hope to many.
"The brain is the most complex organ in our body, which gives rise to thoughts, emotions, and ultimately choice."
-- Unknown
Methodology
In the realm of neuroscience, various methodologies are employed to study neurons and their interactions. These approaches can range from high-tech imaging to understanding cellular behavior at a molecular level.
Description of the Experimental or Analytical Methods Used
Research often utilizes techniques such as:
- Functional Magnetic Resonance Imaging (fMRI): Allows researchers to observe brain activity in real-time.
- Electrophysiology: Provides insight into the electrical properties of neurons, revealing how signals are transmitted.
- Histological Techniques: Enable the visualization of neuron structure through staining and microscopy.
Sampling Criteria and Data Collection Techniques
Typically, data is collected from both animal models and human subjects. This can include:
- Cell Cultures: Neurons grown in lab settings for controlled experiments.
- Longitudinal Studies: Observing the same subjects over time to analyze changes in neuronal function.
- Cross-sectional Studies: Looking at various groups to understand differences across populations.
The comprehensive analysis of these methodologies not only enhances our grasp of neuronal function but also drives the future of neuroscientific inquiry.
Prologue to Neurons and the Brain
The human brain is a marvel of nature, and at the heart of its complex functionality lies the neuron. This fundamental unit is not merely a cellular component; it is the engine driving our thoughts, emotions, and behaviors. Understanding neurons is akin to unlocking the mysteries of who we are as humans. This section lays the groundwork for exploring how neurons contribute to our well-being, cognition, and ultimately our identity.
Understanding Neurons
Neurons, often romanticized as the messengers of the brain, communicate through electrical impulses and chemical signals. They come in various shapes and sizes, each serving unique functions. To grasp their complexity, itās essential to visualize neurons as part of a vast network. You can think of a neuron like a tree:
- Dendrites act like branches, receiving signals from other neurons.
- The cell body holds the nucleus and processes the information.
- The axon extends like the trunk, transmitting signals to other neurons or muscles.
- Myelin sheath, akin to insulation on a wire, increases signal speed.
This intricate structure allows neurons to effectively relay messages, ultimately forming the basis of all our voluntary and involuntary actions, highlighting their importance in both medical and psychological fields.
The Brain's Architecture
The architecture of the brain is as intricate as the neurons it houses. The human brain is divided into several regions, each responsible for specific functions:
- Cerebrum: The largest part, associated with higher brain functions such as thought and action.
- Cerebellum: Positioned at the back, it governs coordination and balance.
- Brainstem: Connects the brain to the spinal cord, controlling essential life functions.
This nuanced architecture showcases how neurons work together within their respective regions to regulate intricate processes. For instance, in decision-making, different neuronal pathways can light up in response to various stimuli, illustrating the brainās collaborative nature. This collaboration is crucial, too, as understanding these connections can inform treatments for neurological disorders or cognitive impairments.
Historical Perspectives on Neuroscience
The journey of neuroscience is a fascinating tapestry woven through time. Early thinkers like Hippocrates hinted at the brain's role in human behavior. Fast forward centuries, the Renaissance sparked a wave of anatomical exploration. Pioneers like RenƩ Descartes and Thomas Willis laid groundwork that has influenced modern understanding. With the advent of technology in the 20th century, neuroscience blossomed into a rigorous field of study.
"From the first rudimentary sketches of the human skull to complex imaging techniques, our understanding of the brain has evolved dramatically."
Recent decades have also seen a plethora of discoveries about neurotransmitters and the neuronal role in comprehending emotions, behaviors, and complex decision-making. Neuroscience today doesnāt just scratch the surface; it digs deep into the implications these findings have on mental health, education, and artificial intelligence, spurring an ongoing dialogue on how we can improve human conditions.
In summary, neurons are not just cells; they are the building blocks of our thoughts, decisions, and behaviors, intricately linked through the complex architecture of the brain. Understanding these units lays the essential groundwork for exploring more extensive topics discussed in subsequent sections.
Types of Neurons and Their Functions
Understanding the types of neurons and their specific roles sheds light on the overall complexity of the human brain's functioning. Neurons are not just simple conduits for signals; each type has a unique role in forming the intricate web of connectivity that underpins our every thought, sensation, and action. By dissecting these categories, we gain valuable insight into how the specialized functions of afferent, efferent, and interneurons cooperate to create a dynamic communication network within the brain.
Afferent Neurons
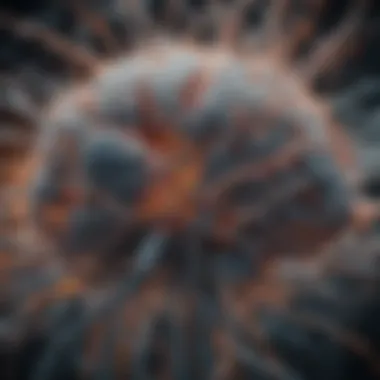
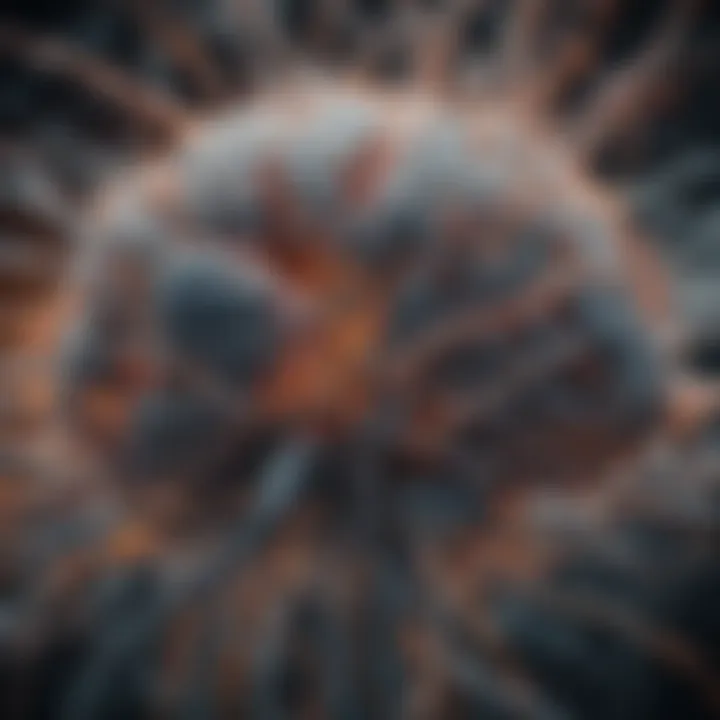
Afferent neurons, also known as sensory neurons, carry signals from the bodyās periphery to the central nervous system. These neurons are crucial in enabling us to interact with our environments. When you touch something hot, for example, it's the afferent neurons that transmit the sensory information straight to your spinal cord and brain, sparking an urgent responseālike pulling your hand back.
- Functionality: They convert external stimuli into electrical impulses.
- Significance: Afferent neurons facilitate our understanding of danger and pleasure, painting a direct sensory picture of what we experience around us. Their presence ensures that we remain in tune with our surroundings, which is vital for survival.
Another aspect worth mentioning is that afferent neurons generally have specialized structures catered to specific types of stimuliābe it temperature, light, or pressure. Therefore, how sensitively they respond can make a significant difference in our interactions with the world.
Efferent Neurons
In stark contrast, efferent neurons, or motor neurons, are the messengers relaying signals from the central nervous system to muscles and glands. Their primary responsibility is to facilitate movement and action. When you decide to kick a ball, efferent neurons come into play, translating your intent into physical action.
- Roles: They control voluntary movements, such as walking or picking up an object, and also manage involuntary activities, like heartbeat regulation.
- Importance: The efficiency and functionality of efferent neurons are paramount for coordinated motor function. If these neurons are impaired, it directly affects oneās ability to moveāa condition observable in various neurological disorders like amyotrophic lateral sclerosis (ALS).
Thus, efferent neurons are integral to transforming thoughts into action, facilitating everything from simple responses to complex bodily movements.
Interneurons
Interneurons serve as a bridge between afferent and efferent neurons. Found exclusively in the central nervous system, these neurons play a vital role in processing information and generating reflexes. Interneurons can range from simple relay connections to more complex circuits that can process sensory data, making sense of it before passing it along.
- Types:
- Functionality: They facilitate communication between sensory and motor pathways, thus allowing for rapid responses to stimuli without the brain's involvement.
- Local interneurons: These operate within a specific area, assisting in quick reflex actions.
- Relay interneurons: They send signals over longer distances in the nervous system.
Interneurons are often described as the brains of the operation. They integrate input from sensory neurons and coordinates responses via motor neurons, ensuring anything from simple reflexes to higher-order functions occur seamlessly.
The intricate nature and role of interneurons highlight how our nervous system can process vast amounts of information at rapid speeds, underlining their significance in everything from reflexes to complex thought processes.
The Structure of Neurons
The structure of neurons is fundamental to understanding how they function within the human brain. Neurons, as the primary signaling units, have unique characteristics that enable efficient communication across intricate networks. By examining their architecture, we can glean insights into how these cells contribute to cognitive functions and overall neurological health. The key elementsāthe cell body, dendrites, axons, myelin sheath, synapsesāwork in harmony to facilitate the transfer of information, which is critical in our day-to-day lives.
Cell Body and Dendrites
The cell body, or soma, serves as the neuron's control center. It houses the nucleus, which contains genetic material necessary for cell function. This component is essentially the brain of the neuron, overseeing metabolic activity and communication. Dendrites, branching out from the cell body, can be thought of as antennas. They receive signals from neighboring neurons, converting complex chemical information into electrical impulses. The greater the surface area of dendrites, the more signals a neuron can receiveābringing to mind the saying, "the more, the merrier."
- Functionality: Dendrites play a crucial role by increasing the neuron's receptive potential, allowing for a rich tapestry of interactions within the neuronal community.
- Implication: Any damage to dendrites can drastically affect a neuron's ability to communicate, leading to detrimental cognitive effects. This is often seen in neurodegenerative disorders.
Axons and Myelin Sheath
Axons are long extensions that transmit signals away from the cell body to other neurons or muscles. Think of them as the highways of the nervous system. What's fascinating is their coatingāthe myelin sheath. This layer acts like insulation around electrical wires, speeding up signal transmission and ensuring efficient communication. Without myelin, neuronal signals would slow down considerably, resulting in diminished performance.
- Speed: Myelin sheath facilitates a faster action potential propagation. A neuron may conduct signals at a stunning velocity of up to 120 meters per second in a myelinated fiber.
- Demyelination: Conditions like multiple sclerosis illustrate the severe consequences of lost myelin, causing severe motor and sensory deficits in affected individuals.
Synapses and Neurotransmission
The synapse is where the magic really happens. Itās the tiny gap between the axon terminal of one neuron and the dendrite of another. When an electrical impulse reaches the end of an axon, neurotransmittersāchemical messengersāare released into this synaptic cleft. Imagine neurotransmission as a game of catch; one neuron throws the ball (the neurotransmitter), and the next one must catch it (on its receptors) to continue the signaling process.
"Neurons that fire together wire together."
This quote captures the essence of synaptic plasticity, a fundamental principle of learning and memory.
- Types of Neurotransmitters: Key players include dopamine, serotonin, and glutamate, each serving different roles in cognition, mood, and behavior.
- Malfunctions: Dysregulation of neurotransmission can lead to psychological disorders, illustrating the delicate balance maintained within our neural frameworks.
Through understanding the structure of neurons, we uncover the intricacies of how they interact, adapt, and respond to internal and external stimuli. This knowledge not only paves the way for advancing treatments for neurological disorders but also enhances our comprehension of the human experience.
Neural Networks and Connectivity
Understanding the neural networks and connectivity within the human brain is essential for unlocking the mysteries of how we think, learn, and interact with the world. Neurons do not simply act as isolated entities; rather, they form intricate webs of connections that underlie all cognitive processes. The significance of analyzing these networks lies in revealing how information is processed and how experiences shape neuronal connections.
One critical aspect of neural networks is the formation of neural circuits. These circuits consist of neurons working in harmony to transmit signals. Connections are made through synapses, which are the junctions where neurons communicate. The interconnected nature of these circuits allows for complex behaviors, sensory processing, and regulation of bodily functions. For example, the reflex arc is a simple neural circuit that enables rapid reactions to stimuli, demonstrating the efficiency of neural pathways.
Formation of Neural Circuits
The formation of neural circuits begins during early brain development and continues throughout life. Neurons undergo a process called guided growth, where they extend axons to connect with appropriate targets based on genetic and environmental cues. The early experiences of an individual can significantly shape these connections. When a child learns to walk, for instance, specific neural circuits strengthen through repeated practice.
- Experience-Dependent Plasticity: This refers to how learning can modify the connectivity in neural circuits. The more a circuit is used, the stronger it becomes.
- Critical Periods: Certain windows during development are optimal for forming specific types of circuits. Missing these periods can affect future learning.
The hallmark of effective neural circuits is their ability to adapt and refine based on usage and demand. This adaptability is tied closely to the concept of plasticity, which we will explore further.
Plasticity and Learning
Plasticity is the brainās remarkable ability to change and reorganize itself. When it comes to learning, plasticity plays a pivotal role. Through mechanisms like synaptic plasticity, connections between neurons can strengthen or weaken depending on activity. For example, when you learn a new skill such as playing an instrument, specific pathways in your brain are reinforced through continuous practice.
- Long-Term Potentiation (LTP): This is often described as a cellular mechanism underlying learning and memory. When two neurons fire together, the synaptic connection strengthens.
- Long-Term Depression (LTD): Conversely, if a synapse is not used, the connection may weaken over time.
Studies indicate that the rich interconnected networks of neurons allow these processes to occur not just in one region of the brain but across multiple areas, enabling integrated learning experiences.
Role of Glial Cells
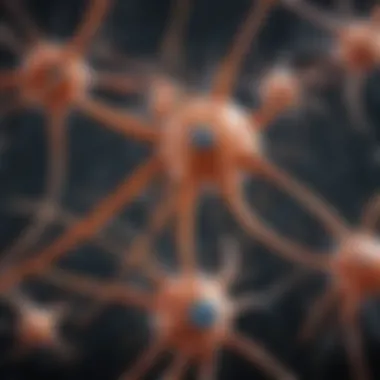
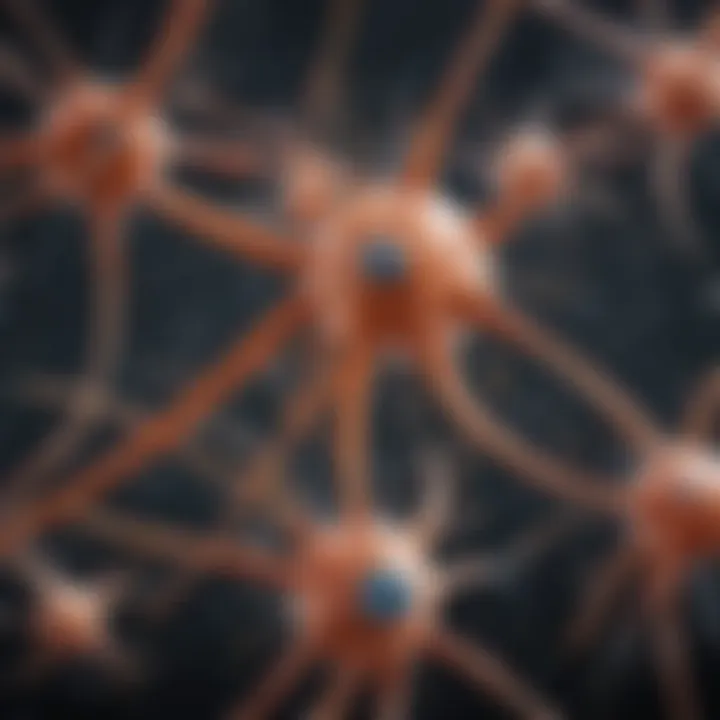
While often overshadowed by neurons, glial cells have an essential role in maintaining the health and functionality of neural circuits. They provide support, protection, and nourishment to neurons. Specifically, astrocytes, a type of glial cell, help regulate neurotransmitter levels and assist in neuronal communication. Some distinguishing roles include:
- Nutrient Supply: Glial cells maintain the ionic balance necessary for neuronal activity.
- Signal Modulation: They can influence synaptic transmission by releasing gliotransmitters.
- Repair Mechanisms: In cases of injury, glial cells are critical for restoring and protecting neuronal networks.
"Neurons may be the stars of the show, but glial cells are the unsung heroes, keeping the performance running smoothly."
Thus, understanding both neurons and glial cells provides a more holistic view of neural connectivity, reaffirming that the brain functions not just through individual neurons, but through cooperative networks in which all components play vital roles.
Neurons and Cognition
Cognition encompasses a vast array of mental processes, from learning new information to making decisions. At the heart of this cognitive machinery lies the network of neurons, which are responsible for transmitting signals and processing information. Understanding how neurons contribute to cognitive functions is crucial, as it provides insight into everything from educational strategies to therapeutic approaches in neurological disorders.
Neural Mechanisms of Learning
Learning is a dynamic process involving synaptic changes and the strength of connections between neurons. When we encounter new information, signals are transmitted through networks, adjusting pathways as necessary. This adaptability, often referred to as synaptic plasticity, is fundamental for effective learning.
For instance, when studying a subject, neurons that fire together tend to wire together. This means frequent activation of specific pathways enhances their efficiency, making it easier to recall that information later on. The role of neurotransmitters, such as dopamine, is paramount here; they not only signal pleasure but also enhance the reinforcement of learning through positive experiences.
Memory Formation and Retrieval
Memory formation is intricately linked to neuron activity. The brain categorizes memories based on their natureābe it declarative, procedural, or episodic. Each type relies on distinct neural structures and mechanisms. For instance, the hippocampus is vital for forming new declarative memories, while the striatum plays a significant role in procedural memory.
"Memory is the treasure house of the mind wherein the monuments thereof are kept and preserved"
- Thomas Fuller
As memories are solidified, a process called consolidation occurs, involving the reorganization of information across networks. Retrieval is equally complex. It often involves reconstructing memories rather than merely pulling them from storage. This reactivation is influenced by the context and cues present at the time of recall, showcasing the adaptive nature of memory.
Decision Making and Problem Solving
Decision making and problem solving are pivotal cognitive processes that rely heavily on the functioning of neurons. These processes often require the integration of various neural inputs, weighing the pros and cons based on past experiences, current context, and anticipated outcomes.
Frontal lobes, particularly the prefrontal cortex, are crucial here. This area is involved in planning, judgment, and reasoning, allowing us to evaluate alternatives before making a choice. Notably, emotional responses also play into decision making, as neurons in the limbic system influence how we feel about options, leading to choices that may not solely be based on rational assessment.
In essence, neurons not only facilitate the mechanics of cognition but also shape how we perceive and interact with the world. As research continues, the intersections of neuron health, cognition, and complex behaviors reveal profound implications for our understanding of personal and collective human experiences.
Health and Disease of Neuronal Systems
The health of neuronal systems is paramount to the functionality of the human brain. Itās not simply about avoiding disease but understanding the intricate web of connections that influence everything from memory to movement. The well-being of neurons directly correlates to overall cognitive performance, physical health, and emotional stability.
Neurons do not operate in isolation; they rely on their surrounding environment, called the neuroenvironment, which includes glial cells, blood supply, and biochemical signaling. When these systems falter, negative consequences follow, impacting cognitive processes and motor functions. The interplay between health and disease in neurons fundamentally shapes how we experience the world.
Neurodegenerative Disorders
Neurodegenerative disorders, such as Alzheimerās disease, Parkinsonās disease, and Huntington's disease, are marked by the progressive degeneration of neuronal function. These conditions not only disrupt the normal operation of the affected neurons but can also lead to widespread neuronal death.
For instance, in Alzheimerās, the build-up of amyloid plaques and tau tangles can effectively choke off communication between neurons. Symptoms often begin subtlyāmemory lapses here, confusion thereābut can escalate to profound impairment over time.
- Key Characteristics of Neurodegenerative Disorders:
- Progressive loss of neuronal function
- Common symptoms include memory loss, movement disorders, and cognitive decline
- Often linked to genetic and environmental factors
The challenges of diagnosing these disorders are compounded by their gradual onset and the complexity of the brain's structure. Early detection remains a frontier worth exploring, as interventions in the early stages may lessen or alter disease trajectory.
Impact of Stress on Neurons
Stress does not just leave emotional scars; it can physically change the structure and function of neurons. Chronic stress leads to excessive release of cortisol, which can hinder neuron growth and promote atrophy, particularly in the hippocampus, a brain region associated with learning and memory.
- Effects of Chronic Stress:
- Reduction of dendritic spines in neurons
- Impaired synaptic plasticity, making it harder for neurons to communicate
- Increased risk for mood disorders like anxiety and depression
Research indicates that prolonged stress can shift the balance of neurotransmitters, impairing overall cognitive function. Stress management strategies, such as mindfulness and exercise, are not just for well-being; they contribute to maintaining neuronal health and longevity.
Neuroinflammation and Health
Neuroinflammation is the brain's response to harmful stimuli, such as pathogens or stress, and it plays a dual role. While this immune response is essential for healing, chronic inflammation can lead to neuronal damage.
"Neuroinflammation can be a double-edged sword, protective in the short term but potentially devastating in the long run."
- Common Causes of Neuroinflammation:
- Infections
- Chronic Stress
- Autoimmune conditions
Persistent neuroinflammation has been implicated in a range of disorders, including Alzheimerās and multiple sclerosis. Understanding how to manage this inflammation can lead to new treatment pathways and ultimately better health outcomes.
Neurons are the foundation of cognitive function, movement, and emotional regulation. Paying attention to the factors affecting their health, such as neurodegenerative diseases, stress, and inflammation, provides insight into how we can foster resilience and enhance our quality of life.
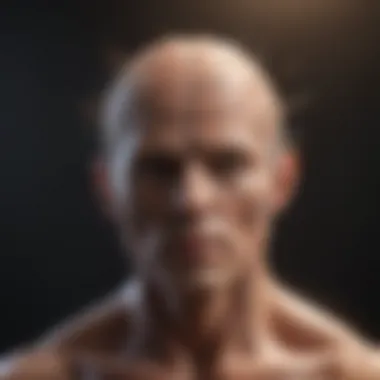
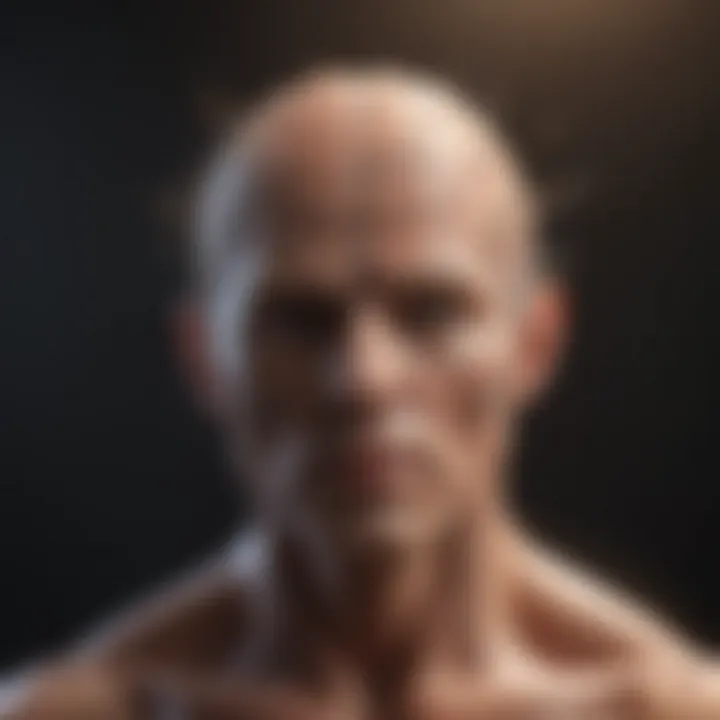
Technological Advances in Neuroscience
In the ever-evolving field of neuroscience, technology continues to change the game. Bringing novel tools and methodologies to the forefront, these advancements play a pivotal role in unraveling complex neural mechanisms. As the intersection of technology and neuroscience deepens, researchers gain unprecedented access to the brain's intricate workings, allowing for exploration that was previously wrapped in mystery.
Engaging with these technologies opens doors to new insights and applications that can profoundly impact our understanding of neuronal function, as well as the broader implications for mental health and cognitive enhancement. Letās break down a few key technological innovations that have been shaping the landscape of brain research.
Imaging Techniques
Imaging techniques revolutionize how scientists visualize the brain. Unlike just a snapshot, advanced methods like functional Magnetic Resonance Imaging (fMRI) provide a moving picture of brain activity. This technique detects changes in blood flow, which is closely related to neuronal activity. It is crucial for understanding which brain areas activate during specific tasks or emotions.
Other techniques such as Positron Emission Tomography (PET) and Diffusion Tensor Imaging (DTI) complement fMRI by allowing more detailed understanding of brain metabolism and the structural connectivity of brain networks, respectively. Key benefits of these imaging techniques include:
- Non-Invasiveness: Patients can undergo scans without the need for surgical procedures.
- Real-time Analysis: Provides data on brain activity in real-time or close to it.
- Mapping Brain Functions: Helps identify regions tied to behavior and cognition.
"The brain is a buzzing mass of activity, but imaging technologies help shed light into its dark corners."
With these tools, researchers can track changes across various functions, aiding in the study of developmental processes or the impacts of neurological disorders.
Neurophysiological Methods
Neurophysiological methods like electrophysiology and patch-clamp techniques delve deeper into the cellular underpinnings of neurons. These methods are all about measuring the electrical properties of neurons in real-time. Electrophysiology captures how neurons communicate via action potentials, revealing how they process and transmit signals.
Patch-clamp techniques allow for the study of individual ion channels on the neuronal membrane. This level of precision gives insight into:
- Ion Flow: Understanding how ions move in and out of cells, crucial for neuronal excitability.
- Neurotransmitter Release: Observing the mechanics behind how signals are passed between neurons.
- Psychopharmacology: Testing how certain drugs impact neuronal activity at a micro level.
Both methods enhance our grasp of foundational neuronal principles and support the development of therapies for various conditions, such as epilepsy, depression, and chronic pain.
Artificial Intelligence in Brain Research
The introduction of artificial intelligence into neuroscience marks a significant leap forward in data analysis and interpretation. With the capacity to analyze vast amounts of data quickly, AI algorithms can uncover patterns in neuronal activity that may be imperceptible to the human eye. This can be particularly helpful in areas like:
- Pattern Recognition: Identifying how neurons interact in healthy and diseased states.
- Predictive Models: Creating forecasts about mental health trajectories based on neural data.
- Enhanced Imaging Analysis: Improving the speed and accuracy of image interpretation from imaging studies.
Using AI not only accelerates research but also opens fresh avenues for diagnosing conditions by analyzing patterns in brain scans and behavior assessments.
The ongoing evolution of these technological advancements nurtures a fertile ground for breakthroughs in understanding the brain. As researchers explore these frontiers, embracing technology's potential will remain an essential key for tackling some of neuroscience's most pressing challenges.
Future Directions in Neuroscience Research
As we stand on the brink of important advancements in neuroscience, the future looks both promising and complex. The exploration into neuron health and technologies that could enhance our understanding of the brain is gathering pace and pushes the boundaries of what we know. This section will focus on gene editing, neuroprosthetics, and personalized treatments, outlining how these developments may take innovation a step further. These fields not only offer potential solutions but also raise critical questions about ethics, accessibility, and the implications for society as a whole.
Gene Editing and Neuron Health
Gene editing technologies, especially CRISPR-Cas9, are revolutionizing how we approach health in neurons. Historically, neurological diseases like Huntington's and spinal muscular atrophy have been challenging due to their genetic roots. With gene editing, we can potentially mutate specific genes responsible for these disorders. Researchers are investigating how altering genetic sequences can strengthen neuron health, possibly even repairing damaged neurons. The prospect of correcting genetic errors at their source is a transformative idea that could lead to effective treatments.
However, there are important considerations here; the precision of gene editing needs to ensure that it doesnāt cause other unintended mutations. The ethical debates surrounding the concept of 'designer brains' must also be addressed. As society grapples with these questions, the benefits of progressing in this area appear to be significant, painting a future that aligns medical interventions closer to genetic science.
Neuroprosthetics
Neuroprosthetics represents a fascinating intersection between biology and technology, focusing on restoring lost functions through artificial means. These are devices engineered to replicate or enhance neurological functionsāthink about bionic limbs or implants that can truly understand and interpret neuronal signals. When a person suffers from spinal injury or neurodegenerative diseases, neuroprosthetics can help restore motor functions, and in some cases, even sensory experiences that may have been lost.
The impact on quality of life can be monumental. Imagine someone who can regain the ability to move with a thought, bridging the gap between intention and action seamlessly. Clinical trials continue to reveal promising results, suggesting neuroprosthetics can enhance cognitive functions too. However, developments in this area come with hurdles, such as the need for biocompatibility and long-term reliability of these devices.
Personalized Neurological Treatments
The era of one-size-fits-all is giving way to personalized approaches in medical treatment, including for neurological disorders. Personalized neurological treatments tailor interventions based on the specific genetic, environmental, and lifestyle factors of an individual. The use of genetic profiling can help in selecting appropriate medications and therapies, minimizing side effects and maximizing efficacy.
Researchers are also looking into biomarkers that hint at how a person might respond to medication or therapy. The benefits are clear: more effective treatment options that consider the unique make-up of individuals could lead to higher success rates in managing conditions such as epilepsy or multiple sclerosis.
Closure
As we unpack the complexities woven into the fabric of neurons within the human brain, the significance of understanding these intricate entities becomes crystal clear. Neurons are not merely conduits for electrical impulses; they are the fundamental units driving our cognitive abilities, behaviors, and emotional responses. In this article, the exploration of neurons has illuminated many critical aspects, from their structural nuances to the profound impact they have on both health and disease.
Neurons' ability to communicate and form networks is fundamental to nearly all brain functions. The myriad connections forged between neurons contribute to everything from basic reflexes to sophisticated thought processes. This indicates that a healthy neuronal environment directly correlates with cognitive performance and overall well-being. Understanding this relationship also emphasizes the importance of maintaining neuronal health through lifestyle choices, such as diet, exercise, and stress management.
"The health of our neurons is intrinsically linked to the quality of our thoughts, decisions, and ultimately, our lives."
Moreover, the exploration of technological advancements, such as imaging techniques and neuroprosthetics, highlights a promising future. These innovations hold great potential to not only enhance our understanding but also to lead to novel therapeutic approaches that can address neural damage or dysfunction. The implications range from improved treatments for neurodegenerative diseases to advancements in personalized medicine.
In essence, this article serves as a gateway to a broader discourse on how neurons are the very essence of our identity as individuals, influencing not just our physical state but the essence of our experience in the world.
Summary of Key Insights
As we summarizethe key insights from our exploration, itās vital to reiterate the following:
- Complex Structure: Neurons are remarkably complex, with their structures enabling specific functions essential to communication in the brain.
- Neural Networks: The interplay and connectivity of neurons foster intricate neural networks, facilitating everything from memory to problem-solving.
- Health Factors: The well-being of neuronal systems is critical, with external factors significantly impacting their health; thus, lifestyle choices play a critical role.
- Research Innovations: Continuous advancements in neuroscience are shaping the future, with techniques like gene editing and neuroprosthetics poised to transform treatment paradigms.
Implications for Future Studies
The future of neuroscience research holds tremendous potential, driven by the need to understand better and address the challenges posed by neuronal health and functioning. Key implications include:
- Understanding Disorders: Ongoing studies are crucial for comprehending neurodegenerative disorders and developing tailored treatment plans.
- Interdisciplinary Approaches: Collaboration between neuroscience and fields like artificial intelligence may enhance knowledge about neural processes and improve predictive models of brain behavior.
- Personalized Treatments: Future research should aim to harmonize findings in genetics, neurobiology, and psychology, fostering personalized treatment options to improve outcomes for diverse populations.