Understanding Plasmid Vector Maps in Genetic Engineering
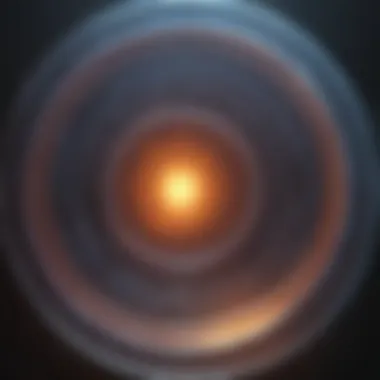
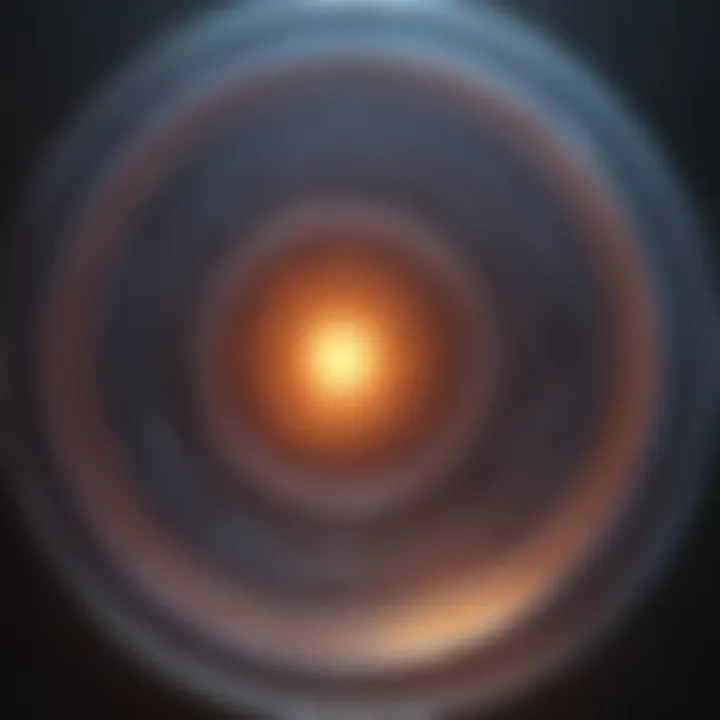
Intro
Plasmid vector maps are vital tools in molecular biology, especially in the realms of genetic engineering and cloning. These maps serve as detailed blueprints, illustrating the structure and components of plasmid vectors. As technologies advance, the precise understanding of these maps becomes even more critical, enabling researchers to manipulate genetic material efficiently.
The intricacies of plasmid vector maps involve various segments and elements. Each component plays a specific role in the processes of cloning and gene expression. Understanding these components is not merely an academic exercise; it has ramifications in biotechnology, pharmaceuticals, and medical research.
This article aims to elaborate on the nuances of plasmid vector maps. It will cover various aspects, including the interpretation of different regions within the maps, their applications in scientific research, and the methodologies associated with creating and utilizing these vectors.
By exploring the structure and function of plasmid vectors, readers will gain substantial insights, whether they are students learning the basics or professionals looking to deepen their knowledge.
Research Overview
Summary of key findings
Plasmid vectors typically contain several critical features: origin of replication, selectable markers, and multiple cloning sites. Through this article, we will clarify each element's role and significance.
Key findings from the research indicate that proper design and understanding of plasmid vector maps can significantly enhance gene cloning success. Moreover, with better knowledge, researchers can select the most suitable vectors for specific applications, streamlining workflows in laboratories.
Importance of the research in its respective field
The relevance of plasmid vector maps in modern biology cannot be overstated. They are central to various biotechnological innovations, including the production of recombinant proteins and gene therapy. The insights gained from this understanding can lead to more effective techniques in genetic research and application. As such, investing time in comprehending these maps benefits both academia and industry outcomes.
Methodology
Description of the experimental or analytical methods used
To create plasmid vectors, scientists typically employ techniques such as restriction enzyme digestion and ligation. This standard methodology allows for the precise insertion of genetic material into plasmids. When generating plasmid vector maps, DNA sequencing and bioinformatics tools are also essential for visualizing and analyzing the maps.
Sampling criteria and data collection techniques
The selection of plasmids for study is based on several criteria. Researchers may choose based on the vector's ability to replicate in host organisms or the compatibility with specific selectable markers. Data is often collected through experimental trials and observations, ensuring that results lead to a deeper understanding of plasmid functionality.
Plasmid vector maps are not only foundational in research but are also instrumental in driving advances in genetic manipulation and therapeutic developments.
This overview sets the stage for further exploration of specific components, methodologies, and applications related to plasmid vector maps, laying a foundation for a comprehensive understanding of the subject.
Preamble to Plasmid Vector Maps
Plasmid vector maps are an integral element of modern molecular biology. They serve as graphical representations of plasmid constructs, showcasing the essential features that enable scientists to manipulate genetic material effectively. Understanding plasmid vector maps is crucial for anyone involved in genetic engineering, synthetic biology, or any kind of research that utilizes plasmids in experiments. Their importance lies not just in their ability to guide experiments but also in their role in advancing scientific knowledge and biotechnological applications.
The value of plasmid vector maps comes from several critical elements. First, they provide a clear overview of the plasmid's structure, allowing researchers to comprehend the location of various components such as origins of replication, selectable markers, and cloning sites. Second, a well-defined vector map facilitates the design of experiments, ensuring that researchers can effectively transfer and express genes of interest in host organisms. Efficient use of these maps can lead to successful outcomes in cloning and protein expression studies.
Several benefits are associated with the utilization of plasmid vector maps. They enhance collaborative work, as scientists can easily share and discuss their designs with colleagues. This sharing of ideas can lead to innovative applications and improved methods in the lab. In addition, a thorough understanding of vector maps can reduce errors in experimental protocols, thus saving time and resources.
When considering the introduction to plasmid vector maps, one must appreciate the profound impact they have on the field of molecular biology. Their continuing evolution reflects advancements in technology and insights into genetic manipulation strategies. In the sections that follow, we will explore the foundational concepts related to plasmid vectors.
Components of a Plasmid Vector Map
Understanding the components of a plasmid vector map is essential for anyone working in the fields of molecular biology and genetic engineering. These components outline the functionalities and qualities of the plasmid, serving as a blueprint for successful experimental designs. Each element contributes significantly to the overall purpose of the plasmid, whether it involves cloning genes or producing proteins. A well-designed plasmid vector map integrates these components in a way that ensures effective replication, selection, and expression of the desired genetic material.
Origin of Replication
The origin of replication (ori) is a fundamental element of any plasmid vector. This is a specific sequence of nucleotides where DNA replication begins. Without a functional ori, the plasmid would not replicate within the host cell, leading to failure in gene expression or cloning endeavors. The ori must be compatible with the host—commonly Escherichia coli or other bacteria. Selecting an appropriate ori is critical as it influences the number of plasmids that can exist in a single cell, impacting yield during experiments. Amplicons and plasmids may vary in their replication control; thus, one must assess the desired output when choosing the ori.
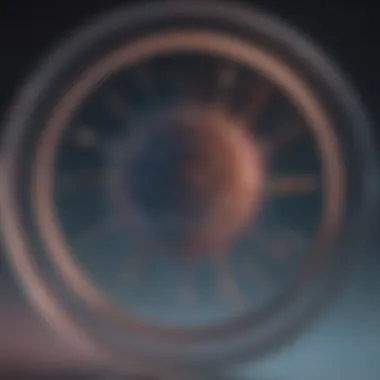
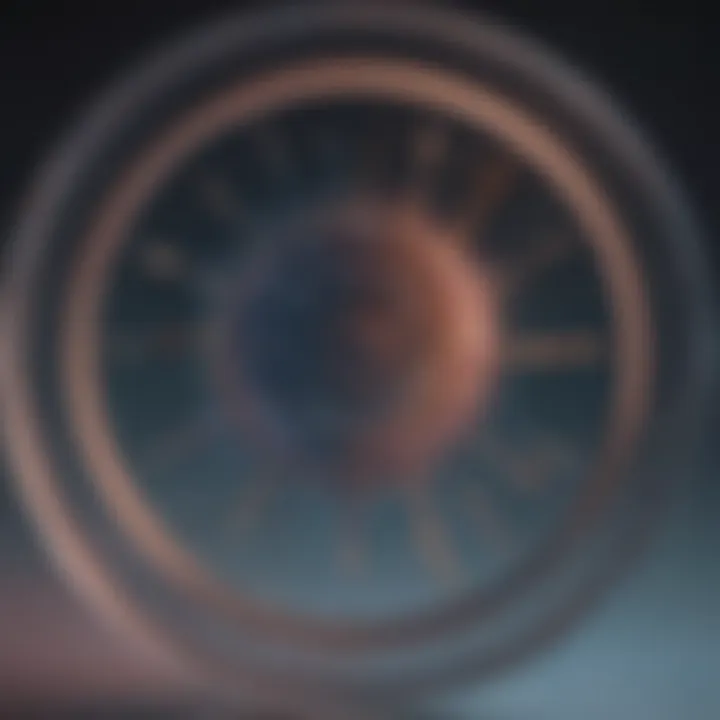
Selectable Markers
Selectable markers are gene sequences that allow researchers to identify and select cells that have successfully incorporated the plasmid. Common examples include antibiotic resistance genes, such as bla (which confers beta-lactam resistance) and cat (chloramphenicol resistance). When the plasmid is introduced into cells, those that have taken up the plasmid can be grown in the presence of the corresponding antibiotic. Only the cells with the selectable marker will survive, ensuring that subsequent analyses focus solely on those cells with the desired plasmid. This step is crucial for efficiency in genetic engineering experiments.
Promoter Regions
The promoter region is the DNA sequence where RNA polymerase binds to initiate transcription of the gene of interest. A strong promoter is vital for high levels of expression of the target gene. There are many types of promoters available, including constitutive, which are always active, and inducible promoters, which can be turned on under specific conditions. Understanding the different types of promoter regions can significantly affect the success of an experiment. For example, using the T7 promoter often yields substantial amounts of protein when used with a T7 RNA polymerase system. Thus, careful consideration of the promoter is necessary for successful gene expression.
Multiple Cloning Sites
Multiple cloning sites (MCS), also known as polylinkers, are critical for introducing foreign DNA fragments into the plasmid. The MCS contains several unique restriction sites, allowing for the straightforward insertion of genes. These sites are often flanked by restriction enzyme recognition sequences that ensure compatibility with various DNA fragments. The strategic design of the MCS enables horizontal gene transfer and increases flexibility in constructing recombinant DNA. By having multiple cloning sites, researchers can easily tailor plasmids to fit different experimental needs.
Termination Signals
Termination signals are sequences in the plasmid that direct the end of transcription. They are essential for ensuring that the RNA polymerase properly stops transcribing the gene of interest. Inaccurate or missing termination signals can lead to truncated or faulty RNA, which impacts protein production. Thus, being aware of effective termination signals is fundamental for obtaining the full-length product required for successful applications. For instance, common terminators include the T7 terminator specifically designed for use with the T7 promoter, which guarantees precise termination and enhances overall expression efficiency.
Each of these components plays a pivotal role in the functionality of plasmid vector maps. Their careful consideration and incorporation into experimental designs ensure that researchers achieve their desired outcomes in molecular biology and genetic engineering processes. Understanding these elements is pivotal for scientists aiming to navigate this complex landscape effectively.
The Importance of Plasmid Vector Maps in Research
Understanding plasmid vector maps is critical in modern molecular biology. These maps serve as roadmaps that guide researchers and professionals in manipulating genetic material. By laying out the essential elements of plasmids, these maps facilitate the design and implementation of various genetic engineering techniques. They provide a clear view of important features such as origins of replication, selectable markers, and cloning sites, which are indispensable in genetic modification processes.
The ability to visualize and comprehend plasmid vector maps empowers scientists to streamline their experiments. Well-designed plasmid maps can minimize errors and optimize cloning efficiency. This reduces the time and resources spent during experiments, promoting more productive outcomes and advancing biotechnological applications. As a result, researchers maintain a competitive edge in their fields.
"Plasmid vector maps are fundamental tools that bridge the gap between theory and practical application in genetic research. They hold the key to successful manipulation of genetic sequences."
Applications in Genetic Engineering
Plasmid vector maps are crucial in genetic engineering applications. By providing detailed layouts of plasmid components, these maps allow scientists to clone genes effectively. They identify specific locations where genes can be inserted, ensuring that target sequences are correctly integrated without disrupting essential functions. The clarity offered by these maps translates into a higher success rate in creating recombinant DNA.
Moreover, vector maps support the development of gene therapy approaches. In this context, plasmids can be designed to carry therapeutic genes into host cells. Properly mapped vectors ensure that genes are expressed appropriately. The outcome is potentially groundbreaking treatments for various genetic disorders and diseases.
Role in Protein Expression Studies
The role of plasmid vector maps extends into protein expression studies. These maps detail the promoter regions that control gene expression, crucial for researchers needing to produce proteins in sufficient quantities. By using plasmids with well-defined promoter sequences, scientists can modulate the levels of protein produced, tailoring expressions to meet research or therapeutic needs.
In addition, plasmids containing tags that facilitate purification of proteins are often mapped out. Knowing where these tags are located ensures that proteins can be extracted and purified efficiently. This is particularly vital for studying protein functions or developing biopharmaceuticals, where purity is essential.
Contributions to Synthetic Biology
In synthetic biology, plasmid vector maps play a significant role in the assembly of complex biological systems. These maps allow researchers to design and construct multigene pathways where various plasmids can interact. By using plasmid maps as a guide, scientists can ensure that these interactions occur correctly, leading to desired phenotypic outcomes.
Moreover, the integration of computational tools with plasmid vector mapping enhances the design process. By utilizing software for designing and simulating plasmid interactions, researchers can innovatively explore new functionalities in synthetic organisms. This further enriches our understanding of biological systems and paves the way for novel applications in areas like biofuel production or environmental remediation.
In summary, the importance of plasmid vector maps in research cannot be overstated. They foster crucial applications across genetic engineering, protein studies, and synthetic biology, making them indispensable tools for scientists. For further information on plasmid vector maps, consider visiting Wikipedia or Britannica.
Designing a Plasmid Vector Map
Designing a plasmid vector map is a crucial step in genetic engineering and molecular biology research. A well-designed map serves as a blueprint for constructing plasmids that can effectively carry genetic material for manipulation. Understanding the intricacies of plasmid design equips researchers to create vectors that fulfill specific experimental objectives. Effective design not only improves the efficiency of cloning and transformation but also reduces potential complications that may arise during experiments.
Initial Considerations for Design
Before embarking on the creation of a plasmid vector map, several initial considerations must be taken into account:
- Purpose: Identify the primary goal of the plasmid. Is it for gene expression, protein production, or another application? Understanding the intended use guides overall design choices.
- Vector Backbone: Select an appropriate plasmid backbone that aligns with the chosen application. A backbone with a strong origin of replication and suitable selective markers is essential.
- Insert Size: Evaluate the expected size of the DNA insert. This will influence the choice of restriction enzymes and the overall design of multiple cloning sites.
- Hosts: Choose suitable host organisms for transformation. Different vectors work best in specific hosts, and compatibility is key in achieving successful gene expression.
- Regulatory Elements: Consider necessary regulatory elements such as promoters, enhancers, and terminators. These will affect the transcription and translation of the inserted genes, ultimately influencing the success of the plasmid.
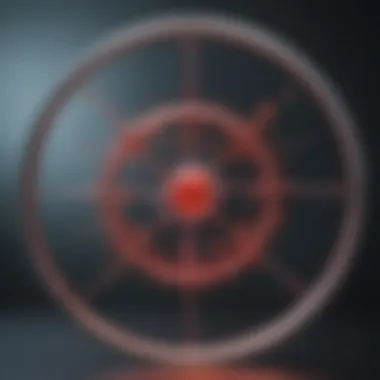
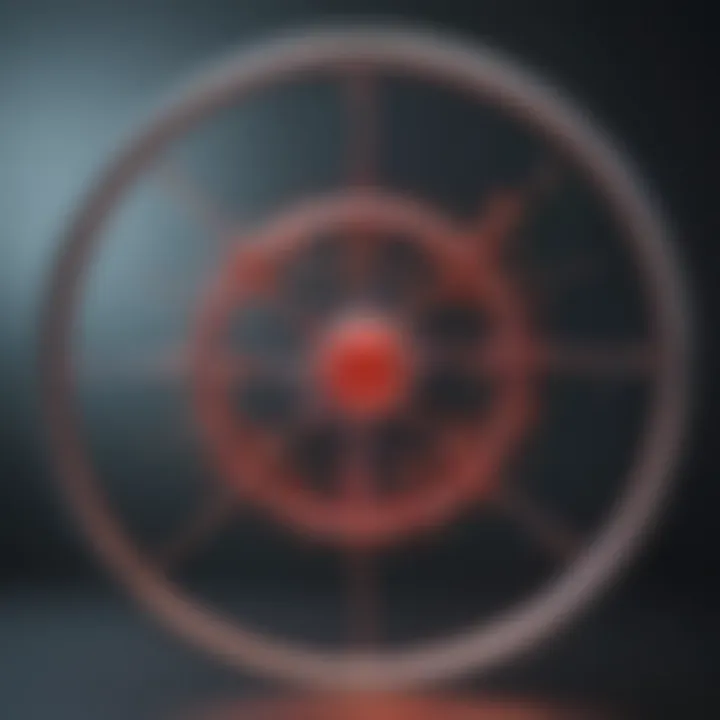
Taking the time to reflect on these considerations ensures that the design process is purposeful and targeted.
Software Tools for Vector Map Creation
Several software tools are available that facilitate the creation of plasmid vector maps. These tools offer various features that simplify the design process and enhance accuracy. Here are some notable options:
- SnapGene: This software allows users to visualize plasmid constructs and experiments easily. It provides intuitive tools for adding features and viewing selection markers, along with detailed annotations.
- Vector NTI: Vector NTI is another popular software that supports plasmid design, sequence analysis, and manipulation. It allows for comprehensive editing and can handle large data sets effectively.
- ApE (A Plasmid Editor): This free tool enables researchers to design, edit, and display plasmid maps with ease. It is user-friendly and suitable for both novices and highly experienced users.
- Benchling: Offered as a cloud-based solution, Benchling enables collaborative plasmid design. It provides features for real-time editing and sharing among research teams.
- Geneious: This is an integrated bioinformatics software platform that streamlines the process of molecular biology research. It supports a variety of tasks, including the visualization of plasmid maps and the analysis of DNA sequences.
Using these software tools can greatly enhance the efficiency and precision of plasmid vector map design, ultimately leading to better experimental outcomes.
Experimental Protocols Involving Plasmid Vector Maps
Plasmid vector maps are crucial in molecular biology for a variety of experimental protocols. These maps serve as blueprints, guiding researchers through the processes of cloning and transformation. The correct use of plasmid vector maps enhances the efficiency and success rate of genetic manipulations. Understanding these protocols is vital for anyone engaged in research and application of genetic engineering.
Cloning Techniques
Cloning is the process of creating copies of specific DNA fragments. Plasmid vectors play a central role in this. During cloning, the first step is the isolation of the DNA fragment of interest. The vector, usually a plasmid, is then prepared. This preparation involves the digestion of the plasmid and the DNA fragment using specific restriction enzymes. The resulting fragments then need to be ligated together, forming a new recombinant plasmid.
Using a plasmid vector map aids in identifying various cloning sites within the plasmid. It ensures that the insertion of the DNA fragment occurs at the correct position. This step is essential for maintaining the functionality of the gene, particularly if it is under the control of a promoter. Incorrect positioning can lead to transcriptional or translational problems.
Here are the key elements of cloning techniques involving plasmids:
- Selection of Restriction Enzymes: Choosing the right enzymes based on the restriction sites available on the plasmid vector is crucial.
- Ligation Conditions: Creating an effective environment for ligation maximizes the chances of successful insertion.
- Confirmation of Clones: Techniques such as polymerase chain reaction (PCR) or sequencing are commonly used to confirm the successful insertion of the DNA fragment.
These techniques ensure that the target genes can be reproduced efficiently and accurately, contributing to advancements in genetic research and biotechnology.
Transformation Methods
After successfully cloning the desired DNA into a plasmid, the next step is transformation. This process introduces the recombinant plasmids into host cells. The most commonly used host cells in transformation are Escherichia coli, but other cells can also be employed based on the application.
There are various methods available for transformation. Each method has its own benefits and considerations. The main approaches include:
- Heat Shock Method: This traditional technique relies on temperature changes to permeabilize the cell membrane, allowing plasmid uptake. It is simple and cost-effective.
- Electroporation: This method uses an electric field to induce temporary pores in the cell membrane, facilitating plasmid entry. It offers higher transformation efficiencies compared to heat shock.
- Chemical Transformation: This method uses calcium chloride or similar chemicals to prepare the cells. This technique often requires less specialized equipment than electroporation.
Once transformation is complete, researchers typically select colonies harboring the desired plasmid using antibiotic resistance markers. This selection is made possible due to the selectable markers included in the plasmid vector map. Thus, understanding and mastering these transformation methods ensures that researchers can efficiently bring plasmid vectors into functional cellular systems.
"Understanding experimental protocols involving plasmid vector maps is key to successful genetic engineering and advancing scientific inquiry."
The integration of these protocols into research emphasizes the importance of a well-designed plasmid vector map. Without this understanding, attempts at cloning and transformation may not yield the desired results.
Challenges in Using Plasmid Vectors
The utilization of plasmid vectors in molecular biology presents significant advantages, but it also comes with its own set of challenges. Understanding these challenges is crucial for researchers and practitioners who aim to optimize their genetic engineering efforts. Recognizing potential pitfalls can lead to better design choices and experimental outcomes. This section explores two primary challenges: recombination events and stability of plasmid constructs.
Recombination Events
Recombination events are a frequently encountered issue when using plasmid vectors. These events occur when plasmid DNA exchanges genetic material within themselves or with chromosomal DNA. This can happen during replication or under selective pressures. The unintended recombination can lead to loss of the intended insert or, in some cases, produce hybrid plasmids with unintended characteristics. This could reduce the efficiency of cloning or hinder expression of the desired protein.
To minimize such occurrences, several strategies can be implemented:
- Utilizing vectors with high fidelity replicative systems.
- Designing the insert so that it contains unique restriction enzyme sites, reducing the chance of unwanted recombination.
- Employing negative selection markers that allow for the identification of plasmids that successfully retained the insert.
Stability of Plasmid Constructs
The stability of plasmid constructs is another significant challenge. Instability can manifest as the loss or alteration of plasmid features over time, particularly during cell division. This phenomenon is influenced by various factors, including the vector design, the host strain, and environmental conditions.
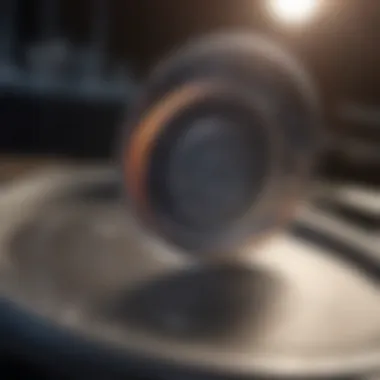
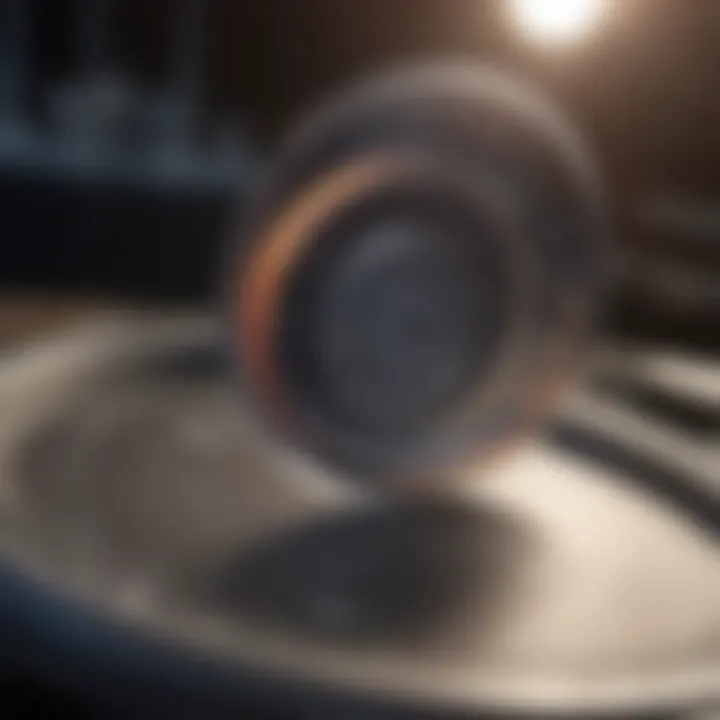
Key factors affecting plasmid stability include:
- Plasmid Copy Number: High copy number plasmids can lead to a greater burden on the host cell and increase the likelihood of segregation during cell division.
- Host Strain: Some bacterial strains may have inherent properties that affect plasmid maintenance. For instance, strains engineered for high plasmid yields may also enhance instability.
- Environmental Stress: Changes in growth conditions, such as nutrient limitations or temperature shifts, can induce plasmid loss.
To improve stability, researchers can:
- Select stable host cells with known compatibility with the plasmid vector.
- Opt for low-copy-number plasmids when high stability is necessary.
- Monitor growth conditions closely to ensure consistent environments during culture.
In summary, addressing the challenges of recombination events and stability of plasmid constructs is essential for effective genetic engineering. These factors must be carefully considered and managed to ensure successful application of plasmid vectors in research.
Understanding these challenges allows researchers to design more effective plasmid vectors and experiments, ultimately enhancing the field of genetic engineering.
Future Perspectives in Plasmid Vector Mapping
The realm of plasmid vector mapping is continuously evolving, driven by advances in technology and an ever-growing understanding of molecular biology. Future perspectives in this domain are of great significance, as they can shape practices in genetic engineering, molecular cloning, and synthetic biology. Embracing innovation while addressing the limitations of current vector systems is vital for researchers and professionals alike.
Innovations in Plasmid Design
Recent innovations in plasmid design focus on enhancing the versatility and efficiency of vector systems. One approach includes the integration of CRISPR technologies, which allow precise genome editing. This method can also be adapted with plasmid vectors, leading to more robust functional studies of genes in various organisms.
Moreover, self-replicating plasmids are being explored. These plasmids can replicate independently without relying on host cellular mechanisms, offering more consistent expressions in transient applications.
Furthermore, synthetic biology has introduced user-defined plasmid construction platforms. Tools like Ginkgo Bioworks create custom plasmids tailored to specific research needs. These advancements can lead to improved plasmid designs that are more efficient and specific for particular applications, ultimately expediting research processes and outcomes.
Potential Impact on Medicine and Agriculture
The impact of advancements in plasmid vector mapping on medicine is substantial. Precision medicine, which tailors treatment based on the individual’s genetic makeup, stands to benefit from improved plasmid systems. Enhanced gene delivery mechanisms can lead to more effective therapies for genetic disorders. For instance, a plasmid designed for delivering therapeutic genes could revolutionize treatments for conditions like cystic fibrosis or muscular dystrophy.
In agriculture, plasmid vectors can facilitate the development of genetically modified organisms (GMOs) that require less chemical pesticide or require less water. By enhancing crop resilience to environmental stresses through genetic modifications, food security can be improved globally. This technology can also contribute to the production of biofuels and bioproducts, which are crucial for sustainable development.
Adopting advanced plasmid vector technologies can substantially accelerate research, leading to breakthroughs in human health and sustainable agriculture.
The future of plasmid vector mapping presents an exciting landscape. Innovations will be critical for overcoming existing challenges, while developing de novo applications across sectors like medicine and agriculture.
The End
The conclusion serves as an integral segment of this discourse on plasmid vector maps, consolidating the information shared throughout the article. This section emphasizes the fundamental aspects of plasmid vector maps, elucidating their roles in molecular biology and genetic engineering. It is essential to acknowledge that these maps represent a bridge between theoretical knowledge and practical application. Consequently, they form the backbone of a vast array of biotechnological innovations and practices.
Summary of Key Points
A succinct summary reinforces the main insights drawn during the article:
- Definition and Components: The article clearly outlines what plasmid vectors are and their vital components, including origins of replication, selectable markers, promoter regions, multiple cloning sites, and termination signals.
- Importance in Research and Applications: It delves into how plasmid vector maps have revolutionized genetic engineering, aiding in protein expression studies and contributing significantly to synthetic biology.
- Design and Protocols: The nuances of designing an effective plasmid vector map are discussed. This touches on initial considerations and software tools that facilitate the creation of these maps. Experimental protocols involving cloning techniques and transformation methods were also explored.
- Challenges and Innovations: It addresses possible challenges encountered in the stability and recombination of plasmid constructs while predicting future advancements in plasmid design and their potential impact on various fields, including medicine and agriculture.
Final Thoughts on Plasmid Vector Maps
In wrapping up the discussion, it is vital to recognize the transformative impact that plasmid vector maps have on contemporary biological research. They enable precise manipulation of genetic material, fostering breakthroughs that were once deemed impossible. As genetic engineering continues to evolve, the understanding of plasmid vector maps will be imperative for students, researchers, and professionals alike.
Ultimately, as methodologies and technologies improve, the future of plasmid vector mapping appears promising. This reflects an ongoing quest to harness the potential of genetic materials for advancements in health, agriculture, and beyond. Exploring this field not only cultivates scientific growth but also inspires innovative solutions to real-world problems.
"Understanding plasmid vector maps is not merely academic; it embodies the future of science and technology."
The journey of plasmid vector maps will undoubtedly shape numerous disciplines, leaving a lasting legacy in the annals of biotechnological progress.
Important Elements to Consider:
- Credibility: References lend authority to the article, enabling informed interpretation of applied methods.
- Further Reading: They guide readers towards additional information, fostering continuous learning.
- Historical Context: They allow for an understanding of how plasmid vector maps have changed over time.
"References not only validate the arguments presented but also pave the way for future research and inquiry."
Through these essential components of references, we ensure the narrative remains informative and compelling, thereby benefiting the audience on multiple levels.