Exploring the Materials Behind Plastics
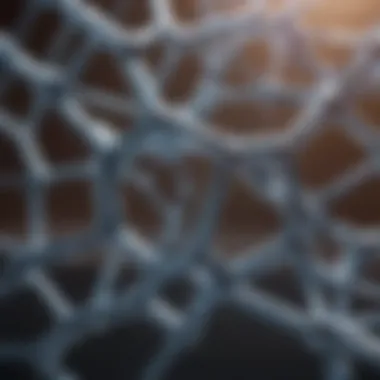
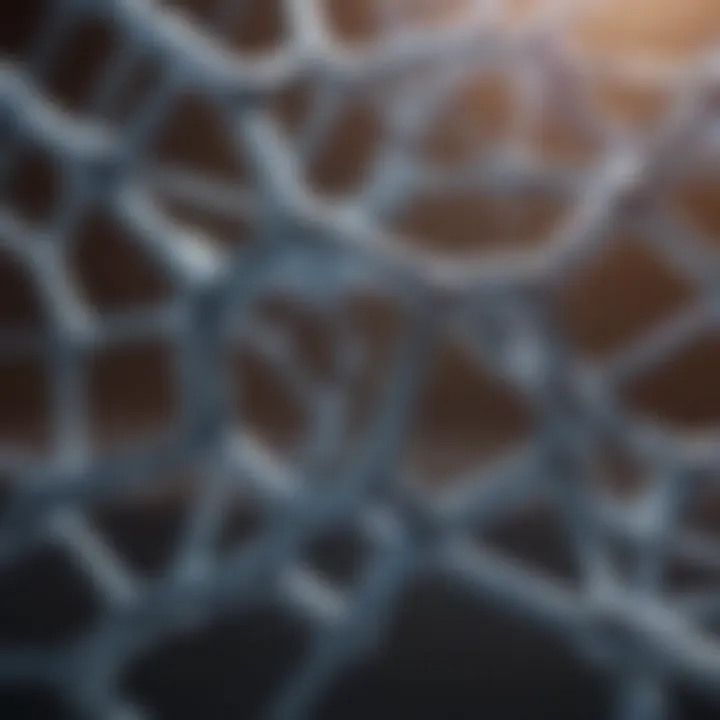
Intro
Plastics are everywhere, from the packaging around your groceries to the tech gadgets that we can't live without. But have you ever stopped to think about what they really are? This article dives into the world of plastics, breaking down their composition and how they come to life in various products. Understanding the ingredients and methods used in plastic production provides clarity about their impact on our lives and the environment.
The composition of plastics involves a complex interplay of raw materials, chemical processes, and additives that enhance performance and durability. At their core, plastics are based on polymers, which are long chains of molecules derived primarily from fossil fuels, particularly oil and natural gas. These polymers can then be modified or mixed with various substances to create different types of plastic, each with unique properties suited for specific applications.
This section sets the stage for a comprehensive exploration of not just the materials that make plastics but also the implications of their use. By delving deep into the origins, processing, and environmental considerations of plastics, we aim to paint a thorough picture of this ubiquitous material.
Research Overview
Summary of key findings
The investigation into the composition of plastics reveals several notable insights:
- Diverse Materials: Various compounds, ranging from polyethylene to polystyrene, constitute different plastics. Each type serves distinct purposes in various industries.
- Chemical Additives: Plastics are not just pure polymers; they often include stabilizers, colorants, and fillers that enhance their performance. These additives, while beneficial, can also pose environmental challenges.
- Sustainability Challenges: The production and disposal of plastics raise significant ecological concerns. Understanding these aspects is vital for developing more sustainable practices in the industry.
Importance of the research in its respective field
This research is critical in several ways. First, it provides clarity regarding the components that make up a material that has become synonymous with modern life. Second, it shines a light on the environmental impact of plastics, which is an urgent matter of discussion within scientific and policy-making circles. The knowledge gained from this exploration can inform future innovations aimed at creating more sustainable alternatives to traditional plastic. Moreover, addressing sustainability helps foster a shift in consumer behavior and corporate responsibility.
Methodology
Studying the composition of plastics involved various analytical techniques designed to decipher the composition and properties of different types of plastics.
Analytical Methods Used
- Spectroscopy: Techniques like infrared spectroscopy were employed to identify the chemical structure of plastics.
- Chromatography: This method helped in separating and analyzing the components within plastic samples, providing insights into the presence of additives.
Sampling Criteria and Data Collection Techniques
In selecting samples for analysis, several factors were considered:
- Type of Plastics: Samples were chosen based on their prevalence in consumer products, such as Polyethylene terephthalate (PET) and Polyvinyl chloride (PVC).
- Regional Variability: Samples from various geographical locations were collected to assess differences in composition due to local regulations and manufacturing practices.
- Product Lifespan: The stage of the product lifecycle was also taken into account to understand how usage influences the composition and degradation of plastics.
This investigative approach aims to provide a balanced and thorough understanding of the various facets that contribute to the composition of plastics. By exploring the materials, methodologies, and implications associated with plastic use, we hope to equip our audience with the knowledge to engage in informed discussions about the role of plastics in modern society.
Prologue to Plastics
Definition of Plastics
Plastics, broadly defined, are synthetic or semi-synthetic materials primarily composed of polymers. These polymers are long, repeating chains of molecules, which can be manipulated to create materials of varying properties. An interesting way to think of plastics is as a chef's secret ingredient: the same base material can be transformed into everything from lightweight packaging to durable automotive parts.
The versatility of plastics lies in their ability to be molded, extruded, or cast into countless shapes. They can be both flexible and rigid, transparent or opaque, making them suitable for a wide array of applications. Plastics have indeed become synonymous with convenience and modernity, yet they also present a dynamic challenge when we consider their environmental impact, particularly concerning waste and pollution.
Historical Development of Plastics
From the late 19th century, the world saw the first synthetic plastics emerge. Bakelite, invented in 1907 by Leo Baekeland, marked the dawn of commercial plastics. This early plastic, created from phenol and formaldehyde, was non-conductive and heat-resistant, making it ideal for electrical insulators. Little did Baekeland know, he had set off a revolution.
Over the decades, various other formulations came about, including polystyrene, polyethylene and polyvinyl chloride (PVC). Each new type of plastic opened doors to innovation in industries like packaging, construction, and consumer goods. Nonetheless, the explosive growth in plastic production post-World War II has created a double-edged sword. While plastics contribute to progress and convenience, their persistent footprint in the environment is increasingly concerning.
As we move through the rest of this article, we'll explore the raw materials driving plastic production, the multitude of plastic types, and the health and environmental implications tied to our reliance on these materials. It's a deep dive into the compounds that shape our reality—one that demands our awareness and understanding.
Raw Materials Used in Plastic Production
The realm of plastics is intricately linked to the raw materials that serve as their foundation. Understanding these materials not only helps demystify the plastic production process but also highlights the implications for sustainability and environmental impact. In this section, we will explore the primary sources of plastic raw materials, specifically fossil fuels and biobased alternatives, emphasizing their roles, benefits, and the considerations that arise when utilizing them.
Fossil Fuels as Primary Feedstocks
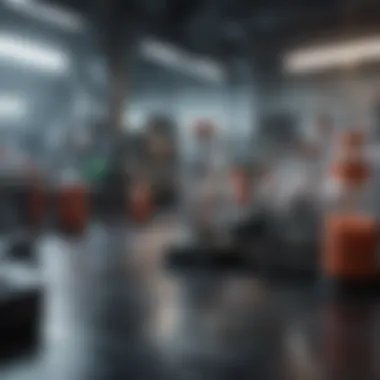
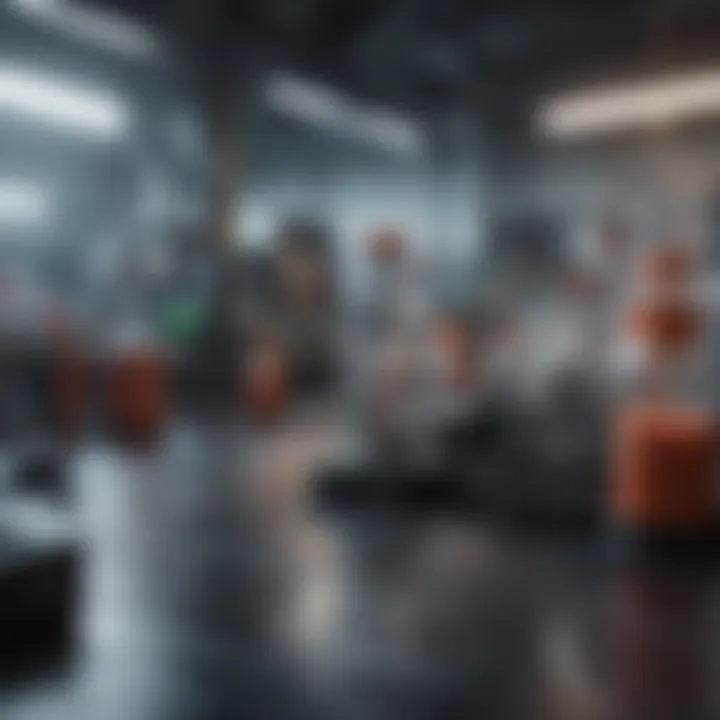
Fossil fuels, notably crude oil and natural gas, are the traditional and most commonly used feedstocks in plastic production. When we talk about plastics, we often think of their versatility and durability, but it’s essential to recognize that these attributes largely hinge upon how they are made from these fossil resources. Crude oil is refined into various fractions, which include naphtha, a key ingredient in producing polyethylene and polypropylene.
One could argue that fossil fuels have revolutionized plastic manufacturing, but this reliance comes with significant drawbacks. Here are some important aspects to consider:
- Environmental Impact: The extraction and processing of fossil fuels are often associated with greenhouse gas emissions, contributing to climate change. Moreover, oil spills and other accidents can have devastating effects on marine ecosystems.
- Resource Depletion: As global demand increases, the finite nature of fossil fuel resources raises questions about long-term viability. It’s a ticking clock, and many experts suggest that we’re already heading towards a crisis point in terms of supply.
- Profitability vs. Sustainability: While fossil fuels can yield high quantities of plastic at relatively lower costs, this doesn’t take into account the hidden costs related to environmental degradation and health risks from pollution.
Thus, while fossil fuels have catalyzed the development of the plastic industry, they also present serious challenges that cannot be ignored.
Biobased Plastics: An Alternative
In response to the evident flaws associated with fossil fuel reliance, the rise of biobased plastics brings a glimmer of hope. These materials are derived from renewable biological sources such as corn, sugarcane, or even algae. Unlike their petroleum-based counterparts, biobased plastics aim to offer a more sustainable option that could reduce reliance on fossil fuels. Here’s what makes biobased plastics an intriguing alternative:
- Renewability: Since biobased plastics come from renewable resources, they present a lesser burden on the planet compared to fossil fuels. Crops can be replanted annually, and as long as careful agricultural practices are employed, the sources remain sustainable.
- Lower Carbon Footprint: Biobased plastics typically require less energy in production than traditional plastics. This results in reduced carbon emissions, making them a cleaner option for manufacturers and consumers alike.
- Potential for Compostability: Some biobased plastics can be designed to break down under certain conditions, contributing to waste reduction. This characteristic is particularly important in waste management discussions.
Yet, there are challenges with biobased plastics as well, such as agricultural impacts on land use and food supply competition. To understand and navigate these complexities is to grasp the broader implications of material choice in plastic production.
In summary, raw materials serve as the bedrock of plastic production. While fossil fuels have been the traditional choice, the shift towards biobased options reflects a growing awareness of sustainability and the environmental issues tied to these materials. This evolving landscape emphasizes the need for informed choices in plastic manufacturing, urging manufacturers and consumers alike to examine the implications of their material choices more closely.
Types of Plastics
Understanding the different types of plastics is crucial in the broader discussion of material science and engineering. Plastics can be classified into three primary categories: thermoplastics, thermosetting plastics, and elastomers. Each of these categories possesses unique properties that dictate their usability in various applications, ranging from everyday products to specialized industrial tools. By analyzing these types, we can gain insight into how plastics interact with their environment, their processing methods, and their overall impact on sustainability efforts.
Thermoplastics
Thermoplastics are perhaps the most recognized category due to their widespread use in consumer products. They are characterized by their ability to soften when heated and to harden upon cooling, which allows them to be molded and remolded multiple times without significant degradation.
Some common thermoplastics include:
- Polyethylene: Found in plastic bags and bottles, it is known for its flexibility and durability.
- Polypropylene: Often used in containers, automotive parts, and textiles due to its high resistance to fatigue.
- Polyvinyl Chloride (PVC): Commonly used in pipes and construction materials, valued for its strength and resistance to environmental degradation.
The versatility of thermoplastics makes them a preferred choice in manufacturing. However, their propensity to become brittle over time and their environmental concerns—primarily related to waste and recycling—cannot be overlooked. With advancing technologies, sustainable practices and recycling processes are gaining attention to reduce this waste.
Thermosetting Plastics
In contrast to thermoplastics, thermosetting plastics undergo a chemical change when heated, which results in a hard and inflexible structure. Once set, they cannot be remolded or reprocessed by heat. Examples include:
- Epoxy Resins: Widely used in adhesives, coatings, and aerospace applications, they exhibit excellent mechanical properties and resistance to chemical exposure.
- Phenolic Resins: Essential in electrical insulators and kitchenware, these materials are known for their strength, heat resistance, and fire resistance.
Thermosetting plastics are often chosen for their durability and resistance to deformation under heat and stress. However, this characteristic also presents challenges when it comes to recycling, as the irreversible setting of the polymer means they cannot be reshaped once formed. Effective waste management strategies are still in discussion for these materials.
Elastomers
Elastomers are polymers with elastic properties that allow them to stretch and return to their original shape. Their molecular structure is notably different, featuring long chains that enable flexibility and resilience. Common examples include:
- Natural Rubber: Used in tires and various industrial applications for its excellent elasticity and strength.
- Silicone Rubber: Known for its temperature resistance and flexibility, silicone is often found in medical devices and kitchenware.
The unique properties of elastomers make them indispensable in countless applications, from automotive to biomedical. However, their long-term environmental impact remains a concern, prompting the exploration of alternative materials and recycling methods.
"The choice of plastic—whether thermoplastic, thermosetting, or elastomer—should be guided by a clear understanding of its application, properties, and environmental implications."
In summary, understanding the types of plastics is not only about recognizing their physical and chemical properties but also about considering the broader impacts of their production and disposal. This awareness is vital for students, researchers, and professionals who aim to innovate in sustainable practices within the plastic industry.
Chemical Composition of Plastics
Understanding the chemical composition of plastics is crucial as it lays the groundwork for grasping how different materials interact and behave in various applications. Plastics are not merely a single entity; rather, they are a tapestry woven from countless components, each contributing uniquely to the end product. These nuances can determine everything from durability and flexibility to recyclability and environmental impact.
The composition affects everything, from how easily a plastic can be molded or shaped to its potential health effects on both users and the environment. It is a topic that resonates widely, applicable to students who will one day innovate in material science, researchers exploring sustainable alternatives, educators imparting knowledge on material properties, and professionals seeking insights into product design.
Polymers: The Backbone of Plastics
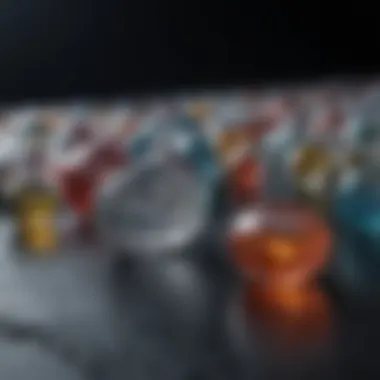
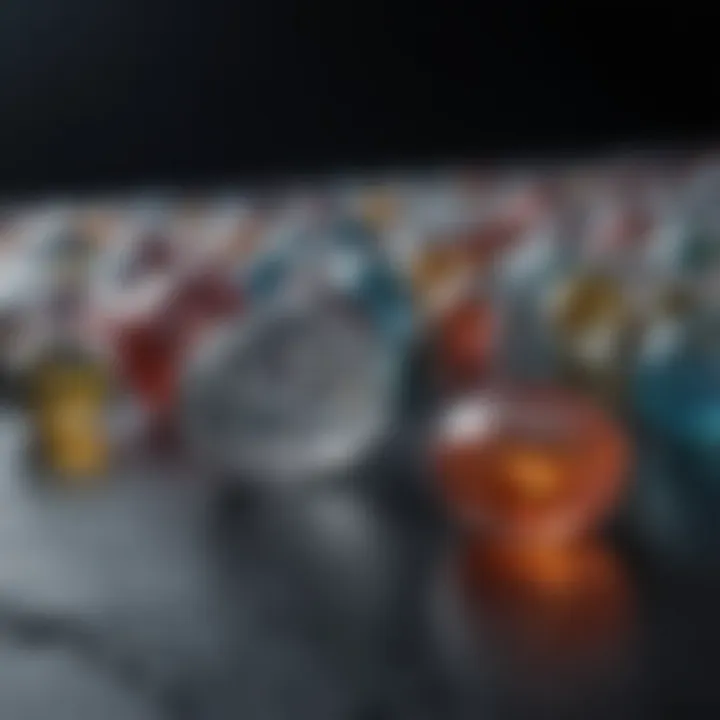
At the core of plastics lies polymers—long chains of repeated molecular units that give plastics their defining properties. Think of polymers as the skeletal structure supporting various forms of plastic. They can be natural, derived from sources like cellulose, or synthetic, engineered from chemical processes used in industries. The choice of polymer plays a major role in determining the plastic's characteristics.
- Types of Polymers: Common types include polyethylene, polypropylene, and polystyrene. Each comes with its own set of attributes:
- Polyethylene is tough and resistant to impact, making it ideal for packaging.
- Polypropylene boasts excellent fatigue resistance, making it suitable for automotive applications.
- Polystyrene offers easy processing and is often used in disposable cups and containers.
A good understanding of these polymers enhances optimization, ensuring the right plastic is used for specific needs. Not to mention, it opens doors to developing environmentally friendly alternatives as industries strive to meet sustainability goals.
Additives and Their Functions
When it comes to plastics, additives play a significant role that often goes unnoticed. These substances are integrated during the plastic formation process, enhancing performance characteristics while addressing specific challenges that raw polymers might present.
- Types of Additives:
- Key Functions of Additives:
- Stabilizers: These help to prolong the life of the product by protecting it from degradation. For example, antioxidants prevent oxidative degradation which can turn a vibrant color to a dull shade.
- Plasticizers: These increase flexibility and workability. They reduce the glass transition temperature, making products less brittle.
- Fillers: Often used to decrease production costs or enhance specific properties, fillers can improve mechanical strength or thermal stability.
- Address performance shortcomings in polymers.
- Contribute to the aesthetic qualities of the finished product.
- Enhance recycling possibilities by improving the nature of the plastic during reprocessing.
Incorporating additives is paramount for creating tailored solutions for a plethora of applications. As the focus on water-based, biodegradable, and recyclable materials grows, understanding how each additive contributes to overall plastic performance becomes even more critical.
"The chemical composition of plastics is not just about their physical attributes, but their impact on our world—both in utility and sustainability."
Thus, being well-versed in the chemical composition of plastics allows stakeholders in the industry to make informed choices that align with environmental responsibilities while meeting consumer needs.
Manufacturing Processes for Plastics
The manufacturing processes for plastics are foundational to the entire industry. Understanding these processes provides insight into how raw materials transform into finished products we encounter daily. Each step in manufacturing not only affects the performance and properties of the final plastic but also bears significant implications for sustainability and efficiency.
Various techniques are employed in the production of plastics, each with its specific applications, advantages, and considerations. By appreciating these differences, one can better grasp the entire lifecycle of plastic products and their impact on both the economy and the environment.
Polymerization Techniques
At the heart of plastic manufacturing lies polymerization, a process crucial for forming the long chains of molecules, known as polymers. Two primary types of polymerization techniques are prevalent: addition polymerization and condensation polymerization.
- Addition Polymerization: This method joins monomers with unsaturation (double bonds) into a new molecule, using catalysts to facilitate reactions. It’s broadly utilized in producing common plastics like polyethylene and polystyrene. The process often yields high molecular weight polymers quickly and efficiently.
- Condensation Polymerization: In contrast, this technique involves the elimination of small molecules, typically water, as byproducts, when two monomers form a bond. It’s common in creating polyesters and polyamides, essential in textiles and engineering plastics.
"Polymerization is the magic that turns small molecules into the diverse plastics that dominate our modern world."
Understanding polymerization not only involves recognizing how plastics are formed. It also leads to awareness of how varying molecular structures can dictate the material's final characteristics, affecting everything from flexibility to temperature resistance. Each choice in polymerization can significantly influence the sustainability of the final product, considering both energy input and waste generated.
Shaping and Molding Processes
Once polymerization has rendered the desired polymer, the next step is shaping and molding. This phase includes techniques like injection molding, extrusion, blow molding, and rotational molding, each suited to specific applications and plastic types.
- Injection Molding: This method forces molten plastic into a mold where it cools and solidifies into a desired shape. It's efficient and ideal for mass production, often used for items like containers, automotive parts, and consumer goods.
- Extrusion: In this continuous process, plastic is melted and forced through a die, resulting in a long shape that can be cut to length. Pipe, film, and sheet are commonly made through this technique, making it versatile for different industries.
- Blow Molding: Here, a hot plastic tube is formed and expanded within a mold using air pressure. This process creates hollow products like bottles and containers, showcasing how molding techniques cater to diverse product needs.
- Rotational Molding: This process involves heating plastic powders in a mold, which is then rotated to allow the plastic to coat the inner surfaces evenly. It's mainly used for larger, hollow items like storage tanks and playground equipment.
These molding processes not only dictate the physical characteristics of the plastic but also introduce variables that influence overall material performance, production costs, and even environmental impact. Adjusting parameters during molding can minimize waste and optimize production efficiency, potentially leading to more sustainable practices in the long run.
Environmental and Health Impacts of Plastics
The role of plastics in modern society has sparked significant discussions, especially around their environmental and health impacts. With plastic becoming so commonplace, it’s vital to explore the challenges posed by plastic waste and the potential hazards associated with toxic substances in plastic production. Understanding these impacts provides a clear view of why sustainable practices are necessary in the production and usage of plastics.
Challenges of Plastic Waste
The problem of plastic waste is not just a minor hiccup; it's a pressing global crisis. About eight million tons of plastic make their way into the oceans every year, causing havoc for marine life and ecosystems. Marine creatures, mistaking plastic for food, often ingest these materials, leading to health problems or death. Plastic debris also disrupts the delicate balance of marine habitats.
"Plastic waste is akin to a ‘whale in the room’ that everyone sees but feels helpless to address."
This signifies the scale of the issue. In addition to marine environments, plastic waste accumulates in landfills, where it can take hundreds of years to decompose. During this lengthy breakdown period, plastics leach harmful chemicals into soil and groundwater, posing risks to local flora and fauna.
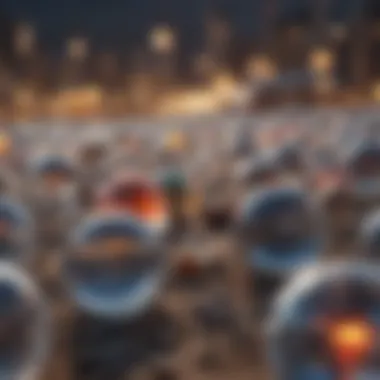
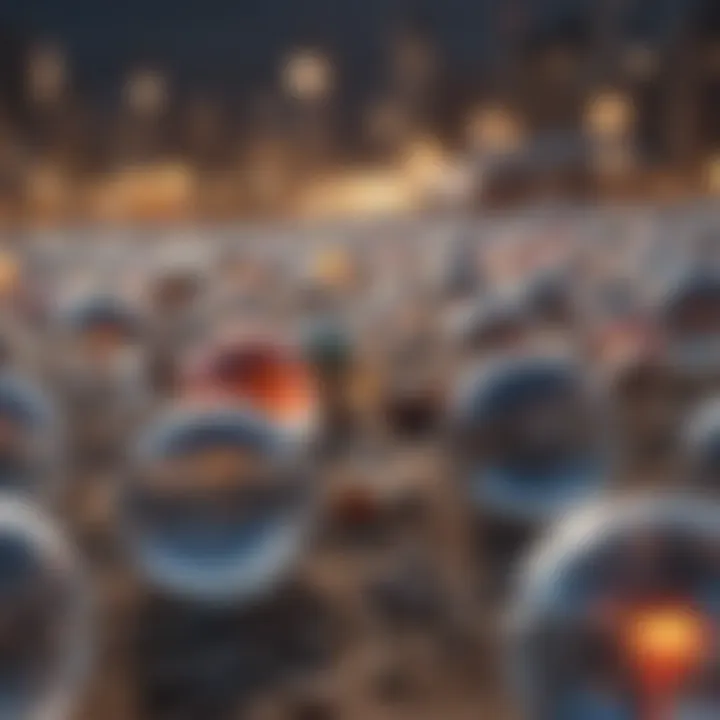
Key challenges of plastic waste include:
- Accumulation in Natural Habitats: Plastics can render environments unsuitable for wildlife.
- Microplastics: As plastics break down into smaller particles, they permeate soil and water sources, becoming a concern for not just wildlife but human health as well.
- Resource Drain: Millions of dollars are spent annually on cleanup efforts, draining resources from other vital environmental programs.
Toxicity Concerns and Safety Regulations
While discussing plastics, one can't ignore the underlying toxicity concerns that often accompany their use. Various chemicals used in the manufacturing of plastics, such as phthalates and bisphenol A (BPA), are known to disrupt endocrine function. These chemicals can leach out during usage, especially in warmer conditions, posing potential health risks.
For instance, studies have shown a correlation between exposure to plastics and various health issues, like reproductive problems and certain cancers. The concern extends to food contamination, wherein food stored in plastic containers might inadvertently absorb harmful chemicals, thus entering the human body.
Safety regulations play a crucial role in managing these risks. Some measures include:
- Limits on Harmful Chemicals: Regulatory bodies like the Environmental Protection Agency (EPA) set limits on certain chemicals in food contact materials.
- Material Alternatives: Promoting the use of less toxic alternatives has become a trend in many sectors.
- Consumer Awareness Campaigns: Educating the public about safe plastic use is increasingly critical, nudging individuals to make informed choices.
In summary, while plastics offer myriad conveniences, they are not without their drawbacks. The challenges presented by plastic waste and toxicity concerns highlight the need for ongoing research and regulatory efforts, paving the way for more sustainable practices in plastic production and consumption.
Future Trends in Plastic Production
The landscape of plastic production is constantly evolving. These changes are significant not only from a technological viewpoint but also from an environmental perspective. As society grapples with the consequences of plastic use, innovations are emerging that aim to lessen its footprint on our planet. Understanding future trends in plastic production is essential for students, researchers, educators, and professionals who are involved in material science, environmental studies, or industry practices. The dialogue surrounding sustainability and ecological responsibility is becoming more pronounced, and the developments in this field are crucial to foster meaningful change.
Innovations in Biodegradable Plastics
Biodegradable plastics are gaining traction as a promising alternative to traditional plastics. Unlike conventional plastics, which can linger for centuries before decomposing, biodegradable options can be broken down more quickly by microorganisms. This trend is a response to the mounting plastic waste crisis, aiming to minimize landfill overflow and ocean pollution.
One memorable example is polylactic acid (PLA), derived from renewable resources like corn starch or sugarcane. The production of PLA not only reduces our dependency on fossil fuels but also results in a material that can decompose under the right conditions within a few months to years. Another noteworthy approach involves using mycelium, the root structure of mushrooms, as a material for packaging and insulation. It’s an innovative way to embrace natural processes while addressing the issues posed by synthetic plastics.
- Key Benefits of Biodegradable Plastics:
- Reduced landfill burden
- Lower environmental impact
- Potential for composting in appropriate facilities
Moreover, there's a growing interest in hybrid plastics which combine bioplastics with traditional materials. These hybrids can retain desirable properties of conventional plastics while offering a step toward sustainability. However, the challenge lies in ensuring that these materials are indeed biodegradable, and that they perform well in their intended applications.
Recycling Technologies and Advancements
Another significant area of focus is the advancement of recycling technologies. As traditional recycling methods often struggle with the complexity of plastic materials, new approaches are being explored to increase efficacy and efficiency in processing recycled plastics.
For instance, chemical recycling technologies are emerging as a game changer. Unlike mechanical recycling that chops up plastics into smaller pieces, chemical recycling breaks them down to their original monomers, enabling the production of new, virgin-quality plastic. This process can effectively tackle the issue of mixed plastics, which often cannot be recycled through conventional means.
Some companies have started using enzymatic recycling—where specific enzymes are employed to degrade plastic waste. This innovative technology allows plastics to be recycled multiple times without significant loss in quality. Furthermore, closed-loop systems are gaining traction, where companies utilize recycled plastics within the same production line, significantly reducing waste.
"The strategic advancements in recycling technologies are crucial in creating a circular economy for plastics, where materials are reused efficiently and sustainably."
- Key Characteristics of Emerging Recycling Technologies:
- Ability to recycle complex plastics
- Enhanced quality of recycled materials
- Reduced energy consumption compared to traditional methods
These advancements do not merely represent progress in technology; they also reflect a societal shift toward greater environmental accountability. Both innovations in biodegradable plastics and the strides in recycling technology signify a collective recognition of our plastic consumption habits and their effects on our planet. Understanding these trends equips individuals across various fields with the knowledge necessary to navigate and influence these changes effectively.
Epilogue
As we wrap up our exploration of the complex world of plastics, it's crucial to mirror on the main threads that weave through this material's narrative. The significance of understanding plastics lies not just in knowing what they are made of, but also in grasping their impacts on our daily lives and the wider environment. Here, we synthesise the key takeaways from this article.
Summary of Key Points
- Definition and Historical Context: Plastics, initially conceived as a marvel of human ingenuity, have evolved dramatically over the decades. Their versatility has made them ubiquitous in everyday life.
- Raw Materials: The production of plastics is intricately tied to both fossil fuels and increasingly researched biobased alternatives. Recognizing these sources helps us appreciate the broader implications for sustainability.
- Types and Chemical Composition: From thermoplastics to elastomers, the diversity in types reflects the various functions plastics serve. At the heart of these materials are polymers, bolstered by additives that enhance performance and durability.
- Manufacturing Processes: Understanding the manufacturing techniques sheds light on how raw materials are transformed into the final products we use. Each step has its own nuances and implications for efficiency and environmental impact.
- Environmental Challenges: It is undeniable that with the benefits of plastics come significant challenges. The issues of plastic waste and toxicity concerns demand our attention and action.
- Future Directions: Innovations in biodegradable plastics and recycling technologies signal a promising shift towards sustainability, but constant vigilant efforts are necessary to usher in genuine improvements.
The Importance of Sustainable Practices
The need for sustainable practices in the plastic industry has never been more pressing. As consumers, researchers, and industry professionals, we have a collective responsibility to push for actionable strategies.
Sustainable practices encompass a broad range of initiatives:
- Reduction of Fossil Fuel Dependence: Shift to renewable resources and feedstocks to lessen the ecological footprint associated with plastic production.
- Advancements in Recycling: Innovate and implement technologies that enhance recycling rates, thereby reducing plastic waste that ends up in landfills and oceans.
- Design Innovations: Encourage designs that factor in the lifecycle of plastic products, creating ease of recycling and minimizing waste.
- Regulatory Frameworks: Advocate for stricter regulations around the use and disposal of plastics to ensure better management and accountability.
Ultimately, maintaining a dialogue about these factors is key. The successful integration of sustainable practices won't just benefit our environment but may also inspire new innovations in plastic technology. Recognizing the intricate connections between our actions and the composition of plastics can empower us to make more informed decisions. This understanding is not an end point, but rather a stepping stone towards a more sustainable and considerate approach to using one of our most versatile yet challenging materials.