Understanding Rapid Response PCR Testing and Its Impact
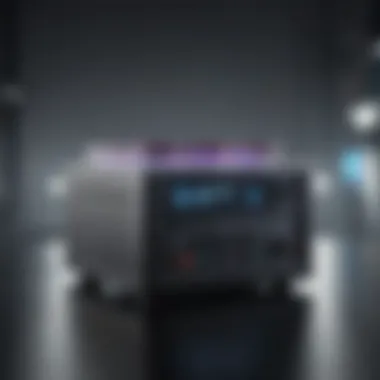
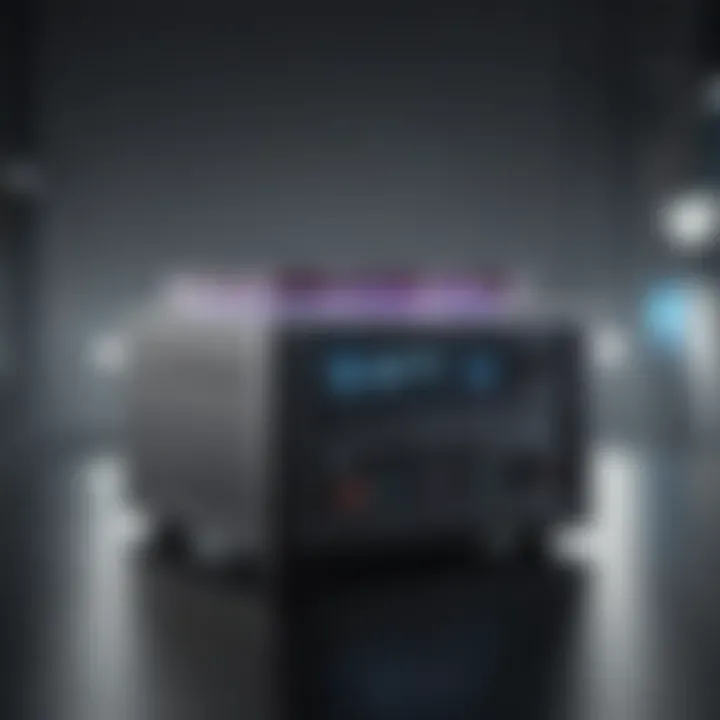
Intro
Rapid response PCR testing has emerged as a crucial player in the realm of diagnostics, providing not just speed but also high accuracy in various applications. This article will unpack the intricacies of this technology, diving into its fundamentals, advancements, and real-world relevance. Now, more than ever, as society grapples with health crises, the importance of understanding these testing methods cannot be overstated. With a clearer perspective, stakeholders across various fields—be it healthcare professionals, environmental scientists, or food safety inspectors—can make more informed decisions.
The global landscape is shifting, and quick identification of pathogens or contaminants is imperative. Rapid response PCR tests enable swift action that can potentially save lives. We will explore how these tests work, examine their various applications, acknowledge their limitations, and look ahead to future possibilities in this fast-evolving field.
Research Overview
Summary of Key Findings
The examination of recent literature highlights several critical points:
- Efficiency and Speed: Current rapid response PCR tests can yield results within hours rather than days, a significant improvement over traditional methodologies.
- Wider Application Spectrum: These tests are not limited to medical diagnostics; they also have roles in environmental monitoring and assessing food safety.
- Technological Advancements: Development in reagents and equipment has led to more portable and user-friendly devices, facilitating testing in diverse settings, from hospitals to remote locations.
Importance of the Research in Its Respective Field
The implications of these findings extend beyond mere data. The research underscores the necessity of rapid diagnosis in combating infectious diseases, especially in pandemic scenarios. The adoption of these tests can drastically streamline response protocols, aiding not only healthcare providers but also environmental agencies and food quality controllers. Rapid response PCR testing represents a paradigm shift in how we approach diagnostics—it’s not just about speed, but amplifying our overall response capability to potential threats.
"Speed and accuracy in diagnostics can be the difference between a controlled situation and a public health crisis."
Methodology
Description of the Experimental or Analytical Methods Used
Recent studies have implemented a variety of experimental approaches to validate the effectiveness of rapid response PCR testing. These methods involve:
- Sample Preparation: Specimens are carefully collected, often using swabs from sites of interest.
- Nucleic Acid Amplification: Advanced PCR protocols allow for the fast amplification of targeted DNA/RNA sequences, crucial for accurate pathogen identification in a short timeframe.
Sampling Criteria and Data Collection Techniques
The studies often utilize pre-defined sampling criteria to ensure consistency and accuracy:
- Diverse Sample Sources: This could include blood samples, nasal swabs, or even environmental samples for broader applications.
- Controlled Conditions: Assisted by lab protocols, samples are processed under strict conditions to minimize contamination and errors in results.
Through this overview, we prepare the ground for a detailed discussion of rapid response PCR testing's underpinnings. The next sections will delve deeper into the mechanics at play, as well as the myriad implications that follow these advancements.
Prelude to PCR Testing
Polymerase chain reaction (PCR) has become a cornerstone in the field of molecular biology and diagnostics. Understanding PCR technology intricately is essential, especially when discussing the evolution and implications of rapid response PCR testing. This segment serves as a primer, elucidating the fundamental aspects of PCR while highlighting its significance in advanced medical diagnostics, environmental assessments, and even food safety.
The ability to amplify specific DNA sequences with precision has revolutionized how we detect and analyze pathogens. In an age where timely and accurate diagnoses can mean the difference between effective treatment and adverse health outcomes, grasping the underpinnings of PCR technology becomes not just beneficial but imperative for students, researchers, educators, and professionals alike.
Defining PCR Technology
PCR is a technique used to amplify a small segment of DNA, allowing for the generation of thousands to millions of copies of that particular DNA sequence. This technology relies on the principle of thermal cycling, which involves repeated cycles of heating and cooling, permitting the selective replication of specific DNA targets. Simply put, if you picture a tiny, intricate factory, PCR acts like the assembly line that efficiently produces a surplus of a crucial product.
The process starts with two key components: DNA templates, which contain the target sequences, and specific primers that attach themselves to the DNA at designated locations. These primers are pivotal because they determine which segment of DNA is amplified. Through repeated cycles involving a three-step process—denaturation, annealing, and extension—PCR produces a vast number of identical DNA copies. The potential applications of this technology stretch far and wide, catering to various fields including medical diagnostics, forensic science, and biological research.
Historical Context and Development
The journey of PCR technology is a tale of scientific innovation and progression. Developed by Kary Mullis in 1983, PCR reshaped the landscape of genetic research and diagnostics. Mullis’s eureka moment came when he realized that by cycling temperatures, he could not only separate the DNA strands but also facilitate their replication with the aid of enzymes, particularly Taq polymerase. This enzyme, derived from the thermophilic bacterium Thermus aquaticus, operates effectively at higher temperatures, making it ideal for PCR processes.
Initially, the technology faced skepticism. Yet, as it proved its worth in various applications—from detecting genetic abnormalities to solving criminal cases—it gained traction. By the late 1980s, PCR became a staple technique in laboratories around the globe. The successful mapping of the human genome in the 1990s further underscored PCR’s significance, paving the way for its integration into countless scientific pursuits.
Throughout the years, PCR technology has seen numerous refinements aimed at enhancing its speed, specificity, and efficacy. Developments such as quantitative PCR (qPCR) have emerged, allowing for real-time monitoring of DNA replication. This constant evolution not only highlights the innovation within the scientific community but also emphasizes PCR's enduring relevance in understanding biological processes and diagnosing diseases effectively.
The Mechanism of Action in PCR Tests
Understanding the mechanism of action in PCR tests is critical. It forms the backbone of how testing processes – especially rapid response PCR – work. A firm grasp on this topic offers a fresh perspective on its efficacy and intricacies, presenting both the power and limitations inherent in PCR technology. Here’s a closer look at the basic principles and those fundamental cycles that drive this testing method.
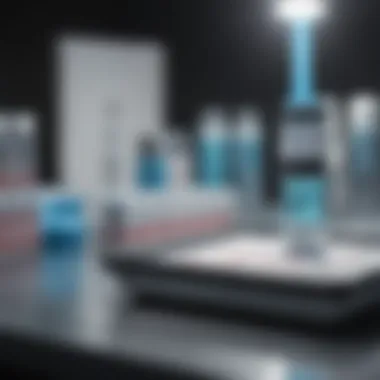
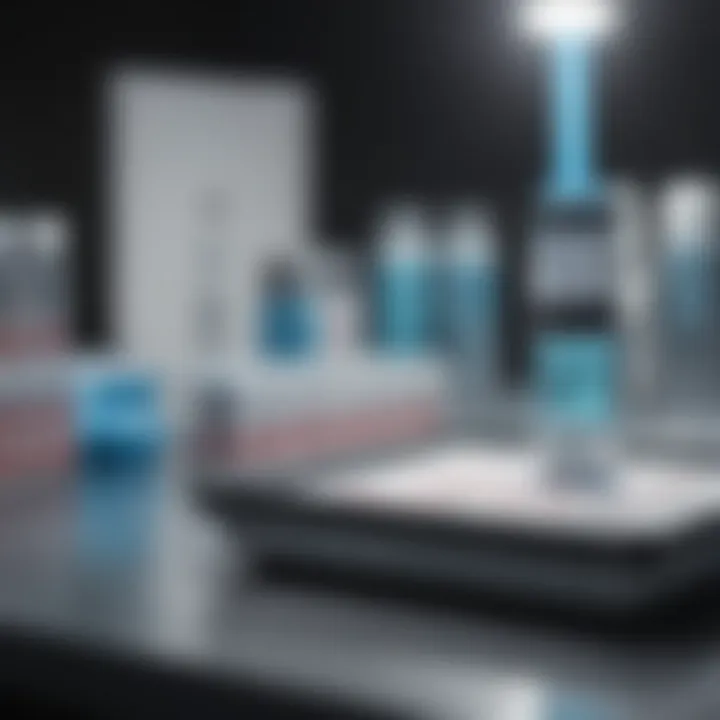
Basic Principles of Polymerase Chain Reaction
The basic principle of Polymerase Chain Reaction (PCR) is relatively straightforward but impactful. Essentially, PCR allows for the specific amplification of a small segment of DNA. It employs an enzyme, DNA polymerase, which synthesizes new strands of DNA based on the template strand provided.
Key Features of PCR:
- Temperature Sensitive: The reaction occurs in cycles involving variations in temperature to enable denaturation, annealing, and extension phases.
- Exponential Growth: This method can exponentially multiply DNA, meaning starting from a tiny sample, a significant quantity can be generated in a short time.
Because of these principles, PCR serves as a powerful tool in diagnostics, allowing for the detection of pathogens at levels that traditional methods might overlook.
Understanding Amplification Cycles
Amplification cycles encompass three crucial phases that together enable the replication of DNA. Here’s a thin slice of those phases:
Denaturation Phase
The denaturation phase is where the magic begins. During this step, the reaction mixture is heated to about 94-98 degrees Celsius. This high temperature breaks the hydrogen bonds between complementary DNA strands, effectively causing the double helix to open up into single strands.
- Key Characteristic: It entirely separates the DNA strands, vital for successful amplification.
- Benefits: This step is essential because it prepares the strands for the next phase, ensuring that all segments are accessible for primer annealing.
- Unique Feature: The efficiency with which denaturation occurs is remarkably rapid, contributing to the overall speediness of PCR testing.
However, if the temperature is not adequately controlled, it could lead to nonspecific amplification, where unwanted sequences might also be replicated.
Annealing Phase
Following denaturation, the annealing phase experiments with cooler temperatures, typically around 50-65 degrees Celsius. During this phase, specific primers bind to the target DNA sequences. Primers are short strands that serve as the starting point for DNA synthesis.
- Key Characteristic: The sequence specificity of the primers is what makes this phase crucial for targeted amplification.
- Benefits: It guarantees that only the desired DNA segment is amplified, avoiding the chaos of non-target sequences.
- Unique Feature: The temperature during this phase can be tweaked based on the primer sequences, offering flexibility in protocol design.
However, too low a temperature could lead to weak binding, while too high could risk the loss of desired sequences.
Extension Phase
The extension phase is where the actual synthesis of new DNA takes place. After primers are bound, the temperature is raised again, usually around 72 degrees Celsius, allowing DNA polymerase to extend the primers and create new DNA strands.
- Key Characteristic: DNA polymerase's enzymatic action is at the heart of this step.
- Benefits: The efficiency and speed with which polymerase can generate new DNA strands significantly contribute to the rapid nature of PCR.
- Unique Feature: This phase can vary in duration depending on the length of the DNA to be amplified. Longer fragments take more time to be completed.
Yet, the specificity of this phase is paramount; if the initial denaturation or annealing steps are faulty, this phase may produce incomplete or incorrect DNA sequences.
The mechanism of action in PCR not only highlights the sophistication of molecular biology but also exposes the complexity and precision needed for successful outcomes. Understanding these phases gives a holistic view of the technology that fuels rapid response PCR testing.
Rapid Response PCR Testing: An Overview
In the landscape of modern diagnostics, rapid response PCR testing holds a pivotal role. These tests quickly and accurately identify pathogens, which is crucial in medical emergencies and outbreak response scenarios. The ability to obtain test results in a matter of minutes rather than hours or days can significantly influence patient outcomes and public health strategies. This agility comes with an array of benefits, considerations, and implications that underline why these tests are increasingly significant in various sectors.
Characteristics of Rapid Response Tests
Rapid response PCR tests are characterized by their ability to deliver quick results without compromising accuracy. Here are some essential elements that define them:
- Speed: As the name suggests, these tests yield results in a fraction of the time compared to their standard counterparts. Typically, results might be available in as little as 30 minutes, making them indispensable in urgent clinical situations.
- Portability: Many rapid response tests are designed for versatility, allowing for deployment in various environments, from hospital labs to remote locations. This flexibility enhances their utility in field operations, especially where traditional lab infrastructure is lacking.
- Ease of Use: Often, these tests require less specialized training to administer, which broadens accessibility. This ease tends to be a game-changer in resource-limited settings or during public health emergencies.
- Cost-effectiveness: While often more expensive per test than standard PCR tests, the speed and efficiency gained can lead to greater overall cost savings by reducing the number of follow-up tests and hospital stays.
Rapid response tests target a range of pathogens, including viruses and bacteria, all while maintaining both precision and robustness. This regulatory compliance ensures that they meet necessary health standards, instilling confidence in their reliability.
Comparison with Standard PCR Testing
When comparing rapid response PCR testing with standard PCR testing, several key differences arise:
- Turnaround Time: Standard PCR tests typically require several hours or even days for results, as they often need a series of amplification cycles and laboratory processing. Rapid response tests dramatically condense this time frame; in critical cases, every minute counts.
- Throughput: Standard tests can process multiple samples at once, which could be advantageous in scenarios involving numerous patients. However, rapid tests provide results quickly, allowing for faster clinical decision-making, which is particularly critical in emergency or outbreak situations.
- Technical Complexity: Standard tests may utilize more sophisticated processes and specialized equipment, while rapid response tests often aim to simplify techniques. This difference can impact the skill level required for administration and interpretation of results.
- Accuracy and Reliability: While both types aim for high accuracy, rapid tests can sometimes lead to false negatives, particularly in low-prevalence situations due to their sensitivity and specificity limits. However, continuous improvements in technology are narrowing this gap.
Rapid response PCR tests are not a one-size-fits-all solution. Their utility is context-dependent, hinging on factors like the specific pathogen, the prevalence in the community, and available resources.
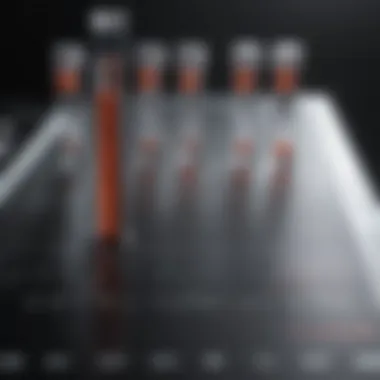
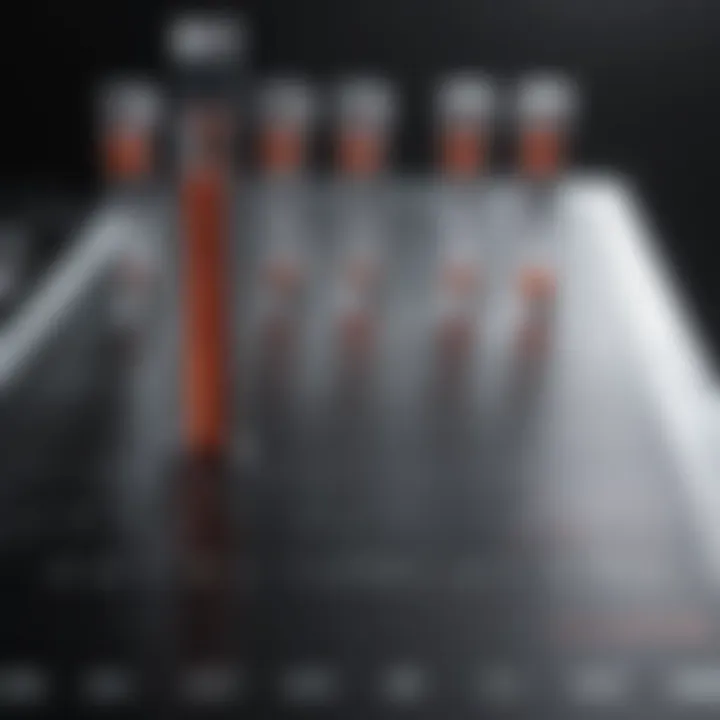
In a nutshell, rapid response PCR testing is transforming the diagnostic landscape. As we consider the broader implications and applications, understanding these differences helps to appreciate their place alongside traditional methods. This awareness can foster a more nuanced discussion about how to leverage these advancements in real-world settings.
Applications of Rapid Response PCR Testing
Rapid response PCR testing has emerged as a cornerstone in modern diagnostics, offering rapid results that can greatly influence clinical decisions. With its ability to detect genetic material from pathogens with remarkable accuracy, this technology has become integral in various fields. Its applications range from healthcare settings to environmental monitoring and food safety, each offering unique benefits that underscore its importance.
Clinical Diagnostics
Viral Infections
In the realm of clinical diagnostics, the detection of viral infections via rapid PCR testing stands out. This method enables healthcare providers to quickly identify the presence of viruses like influenza or SARS-CoV-2. The rapid turnaround time is pivotal, especially during outbreaks, where time is of the essence. Key characteristics of viral infections include their ability to spread quickly and often silently. Rapid diagnostics can drastically reduce control measures’ lag time, fostering timely interventions and potentially saving lives.
One unique feature of viral infection detection is its precision. For instance, tests can differentiate between similar viruses, allowing tailored treatment strategies. However, disadvantages might involve the need for confirmatory testing if results are positive or negative, which can lead to delays in treatment.
Bacterial Contaminations
Similarly, the detection of bacterial contaminations through rapid response PCR holds significant value. Bacteria such as Staphylococcus aureus or Escherichia coli can cause severe illnesses. Rapid testing aids in swiftly diagnosing these infections, facilitating fast treatment. The key characteristic in this context is the technology's sensitivity to low bacterial loads, ensuring that even early-stage infections can be caught promptly.
A notable advantage is the ability to process multiple samples simultaneously, which is essential in high-volume clinical environments. However, like viral testing, bacterial PCR may face limitations related to the risk of false positives, prompting further verification for accurate clinical decisions.
Environmental Monitoring
Pathogen Detection in Water Sources
Rapid response PCR testing plays a crucial role in environmental monitoring, particularly in pathogen detection in water sources. Contaminated water can pose serious public health risks, making its assessment critical. Key characteristics of this application include speed and specificity; rapid PCR can detect pathogens like Giardia or Cryptosporidium within a few hours, ensuring public safety.
The unique feature here is the method’s ability to identify multiple pathogens simultaneously, allowing for comprehensive assessments of water quality. On the downside, costs associated with these tests can be high, which may hinder extensive monitoring programs.
Air Quality Assessment
In the context of air quality assessment, rapid PCR testing can also provide invaluable insights. Assessing airborne pathogens such as Legionella or mold spores is essential for maintaining healthy environments, particularly in hospitals and workplaces. The key characteristic of this application is its efficiency in providing real-time data, enabling swift responses to contamination events.
A distinct advantage of using rapid PCR for air quality is its ability to monitor environmental changes over time, allowing for trend analysis. However, challenges remain, including the need for specialized equipment and trained personnel, which can limit widespread adoption.
Food Safety Assessments
Lastly, the application of rapid response PCR in food safety assessments cannot be overlooked. Contamination can lead to serious health consequences, making timely detection vital. This technology allows for the swift identification of pathogens like Listeria monocytogenes or Salmonella, which can contaminate food supplies. The benefit of utilizing rapid testing in this domain lies in its speed, allowing for quick action to protect consumers.
One unique feature is the ability to test a variety of food types, enhancing food quality assurance. However, sensitivity and specificity issues could lead to regulatory dilemmas, emphasizing the importance of keeping up with testing standards.
In summary, the applications of rapid response PCR testing illuminate its remarkable utility across diverse domains, each benefiting from the technology's speed and accuracy while also facing unique challenges.
Technological Advancements Enabling Rapid Testing
The landscape of diagnostics has transformed remarkably with the emergence of rapid response PCR testing, and at the heart of this evolution lie several significant technological advancements. As healthcare demands escalate in urgency and complexity, the need for faster, more efficient testing methodologies cannot be overstated. Rapid response PCR testing not only meets this demand but also enhances the accuracy and applicability of molecular diagnostics.
Innovations in Reagents
Reagents are pivotal in PCR testing, influencing both the speed and reliability of results. Recent innovations have focused on optimizing these reagents to facilitate quicker reactions. For instance, the development of high-fidelity enzymes has significantly improved the efficiency of DNA amplification. Traditional enzymes often struggle in high-throughput environments, but new formulations allow for rapid cycling temperatures, reducing overall processing time without sacrificing yield or specificity.
Moreover, the introduction of stabilized reagents has enhanced the shelf life and transportability of kits. These reagents can withstand varying temperatures, which is especially vital for settings lacking continuous cold chain logistics. Innovations like these allow laboratories to conduct tests even in remote areas, where access to immediate medical care is limited. As a result, the reach of rapid testing expands, enabling quicker diagnoses in previously inaccessible regions.
Automation and Process Optimization
Automation plays a crucial role in shortening turnaround times for PCR tests. By minimizing human error and increasing throughput, automation ensures that labs can operate at peak efficiency. Modern platforms capable of fully automating the PCR process—from sample preparation to amplification—can produce results in hours rather than days. This is particularly important during outbreaks, where timely identification of pathogens is critical.
The integration of smart robotics and AI-driven analysis systems takes automation a step further. These technologies not only optimize workflows but also improve data management and analysis. For example, automated systems can analyze large batches of samples simultaneously, utilizing algorithms to predict and rectify potential errors before they occur. Such predictive capabilities significantly enhance the reliability of results, providing clinicians with confidence in diagnoses and treatment decisions.
In addition to automation, continuous process optimization practices are crucial. Regularly assessing and refining protocols based on emerging data leads to better resource utilization, identifying bottlenecks in testing processes, and ensuring that labs can adapt swiftly to changing needs.
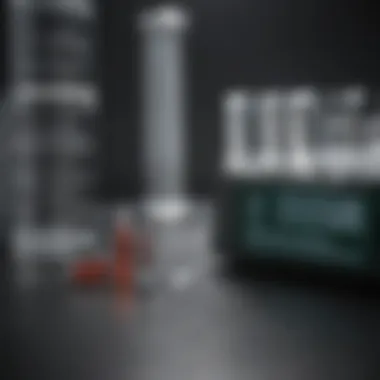
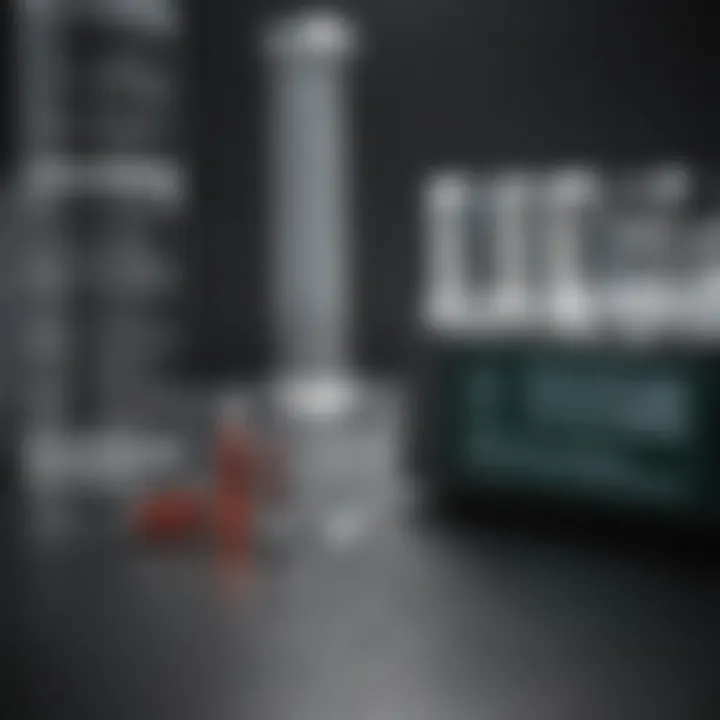
"The fusion of innovation and automation in PCR testing holds the keys to not just rapid results, but also to reliability and accessibility in medical diagnostics."
Challenges and Limitations of Rapid Response PCR Testing
Despite the rapid advancements in PCR testing technology, it's crucial to recognize that there are significant challenges and limitations inherent in rapid response PCR testing. These issues not only have implications for diagnostic accuracy and public health but also affect trust in the technology itself. Addressing these challenges can enhance the reliability of these tests in real-world applications, influencing their adoption across various fields.
Sensitivity and Specificity Concerns
One of the primary challenges faced in the realm of rapid response PCR testing is the matter of sensitivity and specificity. Sensitivity refers to a test's ability to correctly identify those with the disease, while specificity focuses on correctly identifying those without the disease. In an ideal world, a diagnostic test would achieve 100% sensitivity and specificity. However, that's often far from reality.
In rapid PCR tests, there can be a trade-off between speed and accuracy. Due to shortened processing time, some tests may not capture the full cycle of amplification which can lead to false negatives. This essentially means that a person who is infected might receive a negative result, presenting dangers in public settings, particularly during outbreaks of infectious diseases.
"High sensitivity is critical in ensuring patients receive timely treatment, while specificity guards against unnecessary anxiety from false positives."
Additionally, rapid tests may vary widely in how well they perform under real-world conditions. Factors such as sample collection methods, handling, and storage conditions can all influence results. Addressing these changing variables in sensitivity and specificity can guide improvements in test design and implementation, making rapid testing a safer option for patients and healthcare providers.
Regulatory and Standardization Issues
Another hurdle facing the rapid response PCR testing landscape is the lack of regulatory oversight and the need for standardization. While regulatory agencies like the FDA oversee testing, not all rapid tests are subjected to the same rigorous review process as traditional diagnostics. This inconsistency can lead to variability in test performance, making it challenging for practitioners to know which tests to trust.
The absence of standardized protocols for sample handling and processing can also exacerbate inaccuracies. Different manufacturers may have slightly varying methodologies for testing, which can lead to inconsistent results across different platforms.
Establishing clear guidelines and consistent standards is essential. Some possible pathways forward include:
- Uniform Sample Collection Guidelines: Streamlining processes can help mitigate errors.
- Establishment of Performance Benchmarks: Creating clear benchmarks for sensitivity and specificity would set industry expectations.
- Ongoing Monitoring Post-Approval: Continuous evaluation of tests in actual practice can help catch issues early.
To ensure reliability and confidence in PCR testing, it’s vital that these regulatory challenges are addressed. Standardized processes not only elevate the quality of testing but also build a trustworthy relationship between the test providers, healthcare professionals, and patients.
In summary, while rapid response PCR testing holds great potential, its effectiveness is undermined by sensitivity and specificity concerns, as well as regulatory and standardization issues. Logically, improvements in these areas could lead to a more reliable testing environment, essential for both healthcare and public health outcomes.
Future Directions in PCR Testing
As we look to the horizon of PCR technologies, it's clear that rapid response testing is not just a fleeting trend but a pivotal part of modern diagnostics. The ongoing evolution of this field is vital for ensuring that we can address health challenges more effectively and quickly. The incorporation of cutting-edge tech and fresh ideas will undoubtedly expand the capabilities of PCR testing beyond its conventional boundaries, ushering in a new era of efficiency and accuracy.
Integrating AI and Machine Learning
Artificial Intelligence and Machine Learning are making waves in numerous sectors, and PCR testing is no exception. These technologies can analyze vast amounts of data much quicker than traditional methods. Imagine a lab processing samples, where an AI-driven system can identify patterns in results more swiftly than human analysts. Not only does this reduce turnaround time, but it also augments the precision of interpretations.
- Predictive Analytics: AI can assist in predicting outbreak trends, making it easier for healthcare providers to prepare for the unexpected.
- Automation: Integrating machine learning algorithms can automate repetitive tasks in PCR processes, which reduces human error and allows for more streamlined workflows.
In essence, the collaboration between human expertise and AI insights could redefine diagnostic practices, making them more responsive to real-world demands. As much as technology evolves, there's still a need for skilled professionals to oversee and interpret these results.
Expanding Applications Beyond Health
PCR testing has predominantly been associated with medical diagnostics, but the potential for expansion is truly remarkable. Environmental and food safety sectors stand to gain immensely from the advancements in PCR technology. For instance,
- Environmental Monitoring: Rapid PCR can detect pathogens in water sources, contributing to public health safety. The ability to quickly assess contamination levels means timely action can be taken to mitigate risks.
- Food Safety Assessments: As we face increasing concerns about foodborne illnesses, rapid response PCR testing can quickly identify harmful bacteria or viruses in food products, ensuring they are dealt with before reaching consumers.
Moreover, with the integration of AI, these applications may grow into new territories. Industries could fine-tune procedures based on real-time data, leading to practices that reduce waste and enhance safety protocols. The smart use of technology here means that we could spot issues before they escalate, saving resources and protecting lives.
"The future of PCR testing isn't just within healthcare; it's about creating a broad impact across various sectors."
The End: The Role of Rapid Response PCR Testing in Modern Diagnostics
As we wrap up our exploration of rapid response PCR testing, it’s critical to reflect on how these tests are reshaping the landscape of diagnostics. Rapid response PCR is not just a technological advancement; it’s a necessary evolution in an era where swift and precise medical responses can save lives. The significance of rapid PCR testing in modern diagnostics is extensive, stretching from clinical settings to environmental assessments and food safety.
One primary benefit of these tests is their ability to deliver results in real time, which is crucial in fast-paced medical environments like emergency rooms or during outbreak management. By enabling healthcare providers to make informed decisions quickly, rapid response PCR tests can enhance patient care while reducing the spread of infectious diseases. When a healthcare practitioner can promptly detect pathogens, the process for treatment initiation is expedited, easing the burden on both patients and healthcare systems.
Moreover, the ubiquity of these tests is paving the way for expansions into various fields, such as environmental monitoring. Sensitive and quick detection of pathogens in water sources, for instance, can lead to timely interventions that protect public health and safety. This situational agility makes rapid response PCR tests a cornerstone for forward-thinking diagnostic practices.
Key Elements to Consider:
- Accuracy and Reliability: Ongoing advancements have improved the sensitivity of rapid tests, though concerns regarding specificity still prompt critical evaluation. A balance must be achieved to ensure that while speed is prioritized, accuracy is not compromised.
- Operational Challenges: Integration of these technologies poses logistical challenges, including the need for proper training and standardized protocols. For instance, healthcare facilities might require significant adjustments in their workflows to seamlessly incorporate rapid testing.
- Regulatory Framework: As rapid response testing becomes more widespread, regulatory bodies will need to bolster their standards and guidelines to maintain the integrity of diagnostics and public health protocols.
- Multi-Disciplinary Impact: The implications of rapid response PCR technology reach far beyond medicine alone, influencing environmental science and food safety regulations, and even socio-economic factors tied to public health.
"In the realm of diagnostics, speed matters, but precision is paramount. Rapid response PCR testing embodies this crucial balance."
Integrating newfound insights into our understanding fosters an environment where informed actions can be taken, paving pathways for enhanced diagnostics in a variety of fields.