Understanding Sistema Ingenieria: Principles and Impact
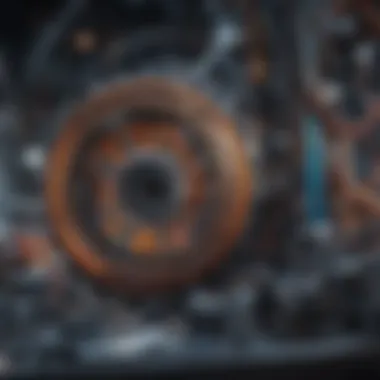
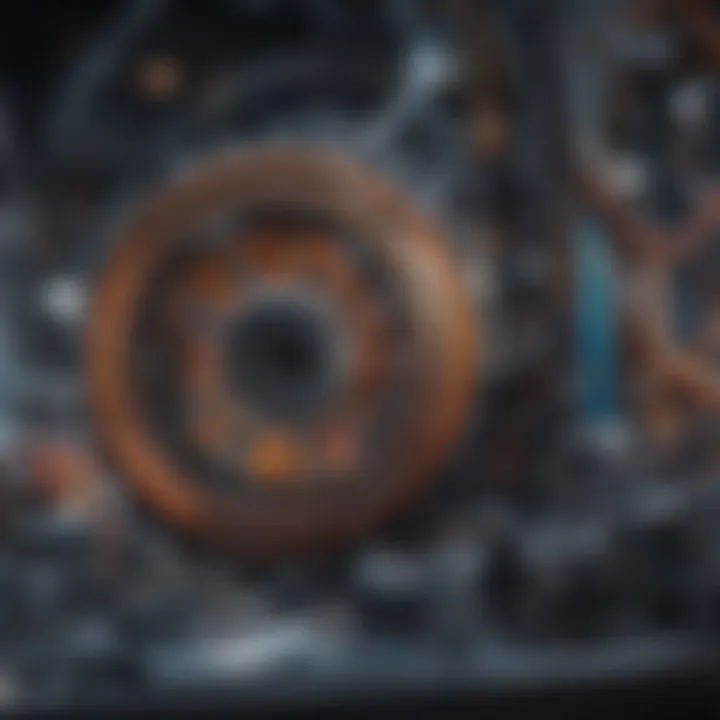
Intro
In the realm of modern engineering, the notion of Sistema Ingenieria, or System Engineering, serves as a cornerstone. This concept transcends mere technical processes to encompass a holistic approach that integrates various disciplines. Whether it's aerospace, software development, or even public health, the principles rooted in system engineering provide a robust framework for designing and managing complex systems effectively.
Understanding this discipline is not just for seasoned engineers but also for students and educators looking to grasp the interconnectedness of technology and systems. This comprehensive guide aims to illuminate the foundations and applications of Sistema Ingenieria, focusing on its key principles and methodologies in diverse settings.
As we delve deeper, we will explore its significance in shaping modern technological advancements, as well as the challenges practitioners face in adapting to rapid changes.
Research Overview
The study of Sistema Ingenieria reveals a tapestry woven from numerous fields of expertise, all essential for fostering innovation in engineering. Here are some of the critical findings and points of interest:
- Integration of Disciplines: System engineering unites varied fields like computer science, electrical engineering, and project management into a coherent methodology.
- Problem-Solving Framework: It provides a strategic lens through which complex issues can be analyzed and resolved, allowing for efficient resource management and improved decision-making.
- Enhancing Collaboration: By breaking down silos within organizations, it promotes teamwork across disciplines, paving the way for seamless project execution.
The importance of this research lies in how it addresses contemporary challenges faced in engineering and technology contexts. In an era driven by constant technological evolution, having a fundamental grasp of system engineering becomes imperative for future innovation. Furthermore, insights gained from this discipline often lead to cost savings and enhanced product quality—a win-win for industries grappling with fierce competition.
Methodology
To truly understand the dynamics of Sistema Ingenieria, we must first look at the methodology behind the research which has been grounded in both theoretical and practical aspects:
- Literature Review: A thorough examination of existing texts and case studies to understand historical context and modern implications related to system engineering.
- Interviews and Surveys: Engaging professionals in various engineering fields to gather qualitative data on their experiences, challenges, and perceptions of system engineering practices.
- Case Studies: Detailed analysis of successful projects implementing system engineering principles, demonstrating tangible outcomes in real-world applications.
Data Collection Techniques
Data was primarily gathered through:
- Qualitative Interviews: Conducted with at least twenty industry professionals, offering insights into practical applications and challenges.
- Quantitative Surveys: Distributed to a wider audience involving various engineering disciplines, providing statistical data on the use of system engineering methodologies in their work.
By merging these methods, the research aims to paint a clear picture of how Sistema Ingenieria manifests within different environments and its impact across sectors.
Prelims to Sistema Ingenieria
System engineering, or Sistema Ingenieria, acts as the backbone of intricate projects, seamlessly bridging diverse disciplines in modern technology. Its significance is far-reaching, touching areas like aerospace, healthcare, transportation, and beyond. By establishing a clear framework, it enhances the efficiency and effectiveness of the development processes, proving essential in our fast-paced world where the stakes are high and the margins for error are slim.
When embarking on a complex venture, the integration of various functional aspects becomes paramount. The art of system engineering lies in its ability to align numerous components within a unified construct. This approach cultivates a holistic viewpoint, ensuring that all facets of a project interconnect and function harmoniously.
Consider the growing need for innovation in various industries. System engineers are tasked with not just solving problems, but preempting them. For instance, in healthcare, adopting a systems approach has led to the creation of integrated medical devices that monitor patient health in real-time, improving outcomes drastically.
The benefits of systematically engineering solutions extend well beyond individual projects. Companies that prioritize this method often experience lower costs, reduced risks, and improved timelines. The iterative nature of system engineering emphasizes continuous feedback and adjustments, fostering a culture of agility that many organizations strive to achieve in today’s market.
Understanding the importance of system engineering also encompasses recognizing its evolution over time. Changes in technology, society, and market demands have shaped its current state, making it even more relevant. Through an insightful exploration of its historical context, we can appreciate how system engineering has adapted and evolved, coaxing it into its modern form – a discipline finely tuned for the challenges of the present and future.
In the subsequent sections, we will dive deeper into specifics that define the realm of Sistema Ingenieria, starting from its fundamental principles to the actual methodologies deployed in real-world applications.
"In the intricate web of modern technology, system engineering stands as a critical pillar, balancing innovation with practicality."
This exploration sets the stage for understanding the core aspects of system engineering, from its definition to its core principles, which shape the way complex projects are executed. As we unfold this journey, we invite readers—be they students, researchers, educators, or seasoned professionals—to consider not just the intricacies of this field, but its immense potential to redefine how we approach problem-solving across various sectors.
Key Components of Sistema Ingenieria
The significance of understanding the key components of Sistema Ingenieria lies in the ability to seamlessly integrate complex systems in various fields. Each component plays a crucial role in determining how effectively these systems can function and evolve over time. This section delves into three primary elements: the systems thinking approach, life cycle phases, and stakeholder involvement. Each of these influences the overall success and sustainability of engineering initiatives, making it vital for students, researchers, and professionals alike to grasp these concepts.
Systems Thinking Approach
The systems thinking approach serves as the backbone of Sistema Ingenieria. It entails recognizing and analyzing the intricate relationships and interactions between different components of a system. In practical terms, this involves understanding how one element impacts another, emphasizing that the whole is greater than the sum of its parts. For example, in urban infrastructure projects, engineers must take into account not only the buildings but also transportation systems, utilities, and environmental factors.
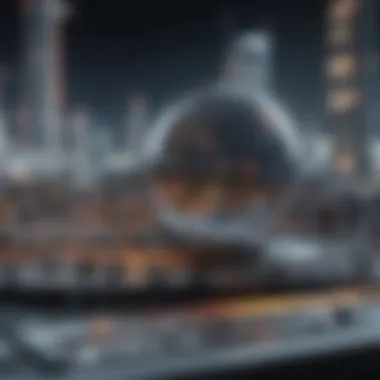
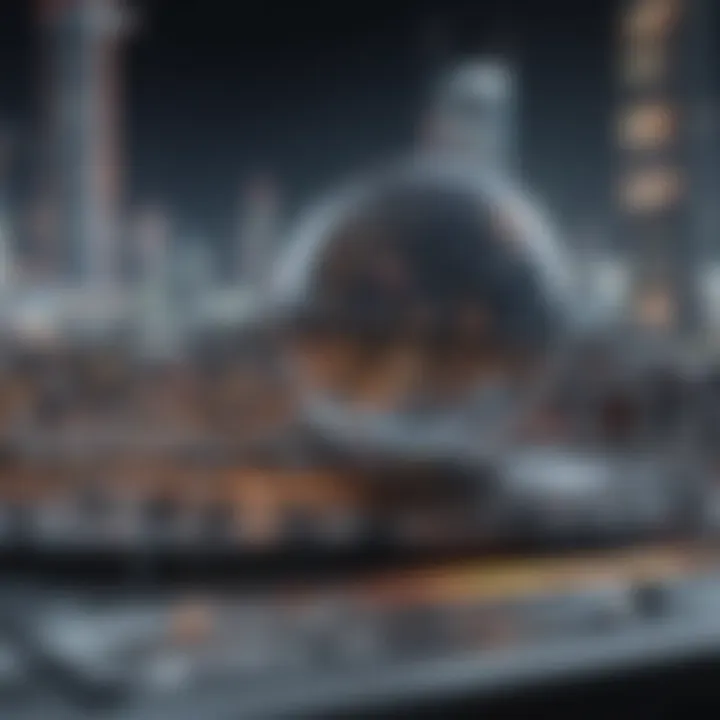
- Interconnectedness: The interconnected nature of systems requires a holistic view. Engineers are urged to consider potential impacts across disciplines, which leads to more sustainable solutions.
- Feedback Loops: By identifying feedback loops within systems, engineers can predict how changes will ripple through interconnected elements. This information is invaluable in managing risks and pinpointing areas for improvement.
- Modeling: Employing methods like simulations aids in visualizing interactions. Tools such as System Dynamics Models allow teams to experiment with various scenarios, leading to more informed decisions.
"Systems thinking transforms the way we approach complex problems, turning potential roadblocks into opportunities for innovation."
Life Cycle Phases
Every engineering system undergoes a life cycle consisting of various phases, from conception to decommissioning. Recognizing these phases is important for effective project management and resource allocation. The life cycle of a system can typically be broken down into:
- Concept Development: This initial phase focuses on defining the problem and determining feasibility. Critical for laying the groundwork, it often involves brainstorming sessions and preliminary design work.
- Design and Development: Engineers devise detailed specifications and blueprints. Prototyping often occurs during this stage, allowing for hands-on testing and iteration.
- Implementation: The construction phase entails putting designs into action. Engineers often face real-world constraints and adjustments must be made on-the-fly.
- Operation and Maintenance: This ongoing phase involves keeping the system functional. Regular assessments and updates are crucial for sustainability and longevity.
- Decommissioning: Finally, systems reach the end of their life cycle. Proper decommissioning ensures safety and may involve repurposing materials.
Understanding each life cycle phase not only streamlines processes, it also promotes sustainable practices that mitigate environmental impacts.
Stakeholder Involvement
In any engineering project, the involvement of stakeholders is paramount. These can range from designers and engineers to end-users and regulatory bodies. Their input can significantly shape the outcome and success of a system.
- Voices of Experience: Engaging with stakeholders provides critical insights based on real-world experiences. Feedback can inform adjustments before deployment.
- Expectation Management: Keeping communication open helps manage expectations. Clear dialogue can mitigate project delays caused by misunderstandings or misalignments.
- Collaborative Solutions: Diverse perspectives can lead to innovative ideas. Effective collaboration among stakeholders can spark creativity, producing more effective and holistic engineering solutions.
Recognizing the importance of stakeholder involvement enhances the practical application of Sistema Ingenieria. Fostering collaboration not only improves project outcomes but also enriches the community's trust in engineering processes.
As one can see, the key components of Sistema Ingenieria shape the very foundation of engineering practices. Understanding these components aids professionals in creating robust systems that stand the test of time and contribute positively to society.
Methodologies in System Engineering
Understanding methodologies in system engineering is crucial in both theoretical and practical frameworks. Methodologies provide a structured approach to tackling complex systems, ensuring that the various components work harmoniously towards a common goal. They enable engineers to plan, analyze, and execute projects in ways that can adapt to change and manage uncertainty. Moreover, by deploying various methodologies, professionals can optimize resources, enhance efficiency, and minimize risks.
Traditional vs. Agile Approaches
When discussing methodologies, one often bumps into the contrasting styles of traditional and agile approaches. Traditional approaches, such as the Waterfall model, follow a linear path where each phase must be completed before the next one begins. This rigidity can be beneficial for projects with clearly defined requirements but can lead to complications in dynamic environments.
On the other hand, agile methodologies prioritize flexibility and responsiveness. They encourage iterative progress, allowing for frequent reassessment and adjustments throughout the project lifecycle. Agile approaches, like Scrum or Kanban, facilitate collaboration and communication among team members, making them ideal for projects where requirements may evolve or change.
Key Differences:
- Structure: Traditional is linear; Agile is iterative.
- Change Management: Traditional is less adaptable; Agile embraces change.
- Collaboration: Traditional often isolates teams; Agile promotes cross-functional teamwork.
Each approach has its merits depending on the industry and project specifications. For instance, the defense sector might benefit more from traditional methods due to regulatory needs, while tech startups may thrive in an agile environment due to the rapid pace of innovation.
Model-Based Systems Engineering
Model-Based Systems Engineering (MBSE) represents a modern twist on traditional approaches by incorporating models as a central component of the engineering process. Rather than merely relying on documents and verbal descriptions, MBSE uses visual representations and simulations to design and analyze systems.
This approach not only enhances understanding but also improves communication among stakeholders. By visualizing complex systems, engineers can identify potential pitfalls earlier in the design process. Key benefits of MBSE include:
- Enhanced Clarity: Visual models can simplify complex concepts, making them comprehensible even for non-technical stakeholders.
- Consistency: By relying on a common model, all parties refer to the same source of truth.
- Early Detection: Potential issues can be caught earlier, reducing costly changes later in the life cycle.
Transitioning to MBSE may require a significant investment in training and tools, but the payoff can be worth it for organizations tackling complex projects.
Verification and Validation Processes
Verification and validation (V&V) processes form the backbone of quality assurance in system engineering. Verification asks, "Are we building the product right?" whereas validation addresses, "Are we building the right product?" These questions are vital, as ensuring both requires rigorous methodologies at various stages.
Steps in &V:
- Requirements Analysis: Ensuring that requirements are clear, complete, and achievable.
- Design Review: Evaluating designs against requirements to confirm feasibility and consistency.
- Testing: Implementing tests to validate both functionality and performance against expectation.
- Documentation: Maintaining thorough records to trace decisions and changes throughout the process.
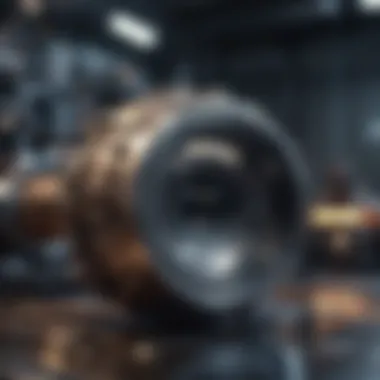
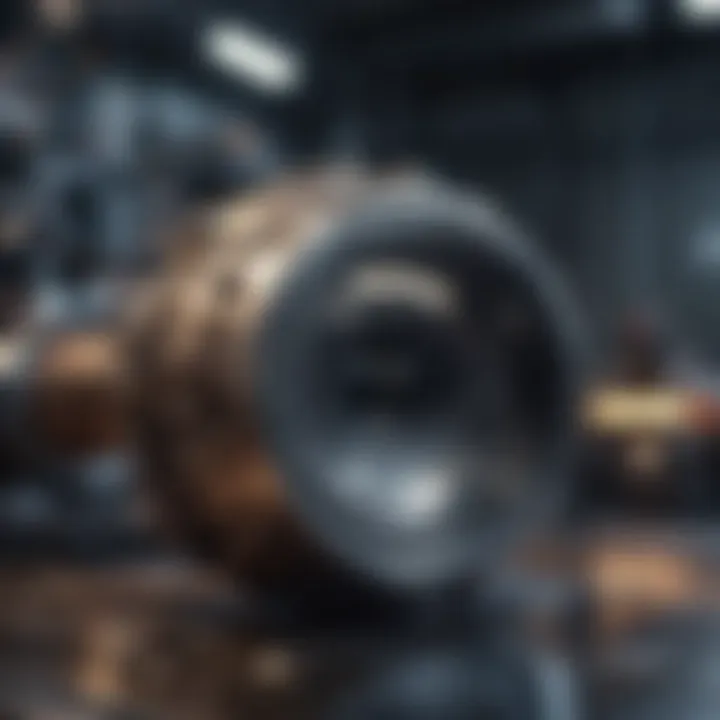
Important Note: A robust V&V process not only minimizes risks but assures stakeholders that the project meets its intended goals.
Applications of Sistema Ingenieria
In today's fast-paced world, the need for effective system engineering applications is greater than ever. This section illustrates the significance and expansive benefits of applying the principles of Sistema Ingenieria across various industries. System engineering acts as a conduit, uniting different disciplines and methodologies that cater to complex challenges present in various sectors. Understanding these applications is crucial in crafting effective solutions and optimizing processes.
Aerospace and Defense
The aerospace and defense industries exemplify the critical role of system engineering in high-stakes environments. In these fields, every detail matters; a minute error can lead to catastrophic outcomes. System engineering ensures that the integration of various components, such as avionics, propulsion systems, and human factors, occurs seamlessly. For instance, the development processes of aircraft like the Boeing 787 involve extensive system engineering to guarantee safety standards and operational efficiency.
Moreover, as military operations become increasingly sophisticated, system engineering serves as a backbone in developing integrated weapon systems and missions. By coordinating technologies and tactics, these units can adapt to rapidly changing scenarios. The complexity of such projects demands rigorous verification and validation processes, which system engineering techniques effectively address, yielding high reliability.
Healthcare Systems
Healthcare systems are another domain where system engineering profoundly influences operations and patient outcomes. Here, the interplay between clinical and administrative processes requires a multifaceted approach. System engineering contributes by enhancing healthcare delivery through optimized workflows, resource allocation, and technology integration.
For example, the implementation of electronic health records (EHR) systems requires a clear framework to ensure all user interfaces communicate effectively across different platforms. Misalignment in these systems can lead to confusion or errors in patient care. Additionally, applying system engineering methodologies can support predictive analytics in patient health management, thus reducing costs and improving health outcomes. Addressing these intricate relationships in the healthcare ecosystem ultimately leads to a system that is both efficient and patient-centered.
Transportation and Logistics
When it comes to transportation and logistics, system engineering plays a central role in streamlining operations. As global commerce expands, the complexities of supply chains require a structured approach to ensure efficiency and minimize delays. This is where principles of system engineering come into play.
For instance, consider a logistics company like UPS. They utilize advanced routing algorithms that rely on system engineering principles to optimize delivery routes. This not only minimizes fuel costs but also affects delivery speed, enhancing overall customer satisfaction. Additionally, in public transportation systems, system engineering is crucial for integrating infrastructure, vehicles, and technology effectively. Projecting future demands and generating solutions before bottlenecks arise is vital for maintaining operation fluidity.
As transportation sectors evolve towards sustainability, the incorporation of systems thinking will remain crucial. New approaches such as electric and autonomous vehicles necessitate a thorough understanding of systems engineering to ensure safety, reliability, and efficiency in design and implementation.
"In essence, applying system engineering principles transforms challenges into opportunities, paving the way for innovation and advancement in various fields."
The breadth of applications in system engineering highlights its importance in addressing intricate and critical challenges across various sectors. Whether it’s enhancing aircraft safety, improving patient care, or optimizing supply chains, the skillful application of these principles fosters developments that ultimately serve society more effectively.
Challenges in Sistema Ingenieria
In any sphere where engineering intersects with complex systems, the challenges can be as daunting as they are multifaceted. Recognizing these challenges is crucial—not only for educators and researchers but also for professionals involved in the implementation and adaptation of engineering solutions. The effectiveness of Sistema Ingenieria hinges upon how well practitioners can navigate and mitigate these hurdles. From integration snafus to financial limitations, every obstacle presents both a risk and an opportunity to innovate and improve processes.
Complexity in System Integration
System integration is a tall order. Just imagine trying to fit a square peg in a round hole, while blindfolded. That’s often how practitioners may feel when attempting to unify disparate components into a seamless system. The breadth of technologies, methodologies, and stakeholders involved in modern projects adds layers of difficulty.
Integration complexity arises from various sources:
- Diverse Technologies: Different platforms and tools can have vastly different capabilities and limitations, leading to mismatches in what is expected versus what is delivered.
- Varied Stakeholder Expectations: Each stakeholder might have a unique perspective, creating a scenario where alignment is essential yet difficult.
- Evolving Standards: Standards can fluctuate, particularly in fast-paced industries, scrambling integration timelines and requirements.
Since misalignment can lead to project delays and failures, companies must engage in thorough planning and continuous management. Effective communication and a clear understanding of each component's role within the larger system can bridge the gap in integration practices. After all, a well-oiled machine is only as good as its weakest cog.
Managing Change and Adaptability
In an world that's ever-changing, system engineers are constantly required to adapt. Managing change is not merely a reactive measure but a proactive strategy aimed at maximizing performance and minimizing disruption. Picture trying to steer a ship through turbulent waters; it’s all about navigating the waves without capsizing.
Key factors that influence adaptability include:
- Organizational Culture: A workplace open to change fosters resilience and agility, encouraging team members to embrace new ideas.
- Stakeholder Communication: Transparent and frequent communication helps everyone stay on the same page, particularly during transitions.
- Robust Change Management Protocols: These create a structured approach for implementation, minimizing resistance and streamlining efforts.
Being able to pivot in response to shifts also permits organizations to harness opportunities that would otherwise be lost in rigidyl designed processes. In the dynamic landscape of engineering, the ability to manage change effectively is not a luxury; it is a necessity.
Budget Constraints and Resource Allocation
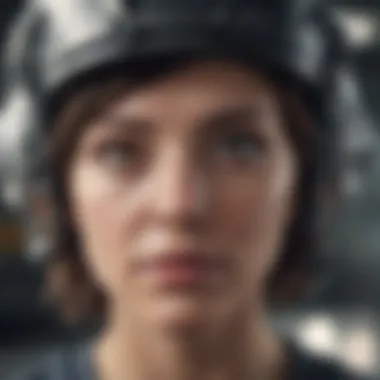
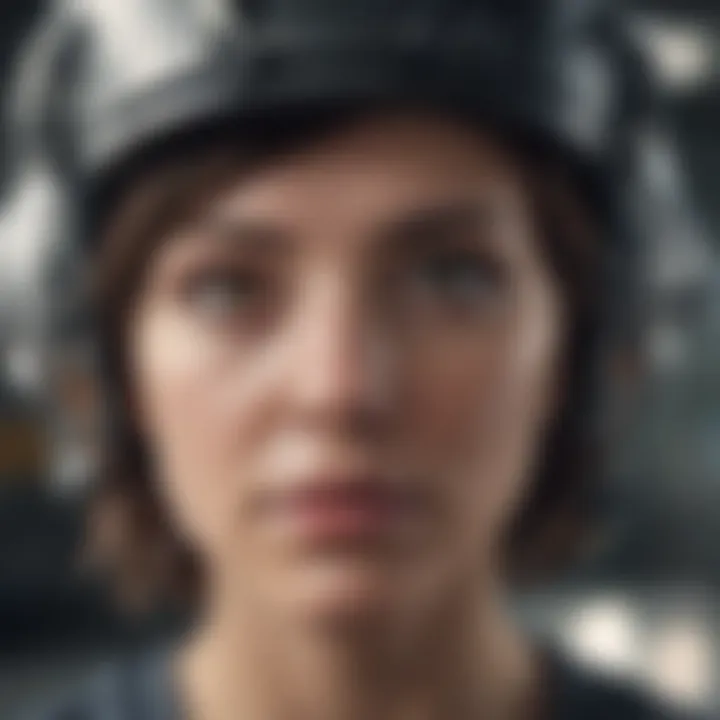
Imagine crafting a beautiful sculpture but only having a chisel and a lump of clay—without the proper resources, your vision remains unfulfilled. Budget constraints are frequently a source of stress in many engineering projects, placing pressure on teams to deliver regardless of limitations.
Several aspects should be considered:
- Prioritization: Knowing which areas deserve the most attention and resources is vital. Focusing on high-impact facets can lead to better overall outcomes.
- Resource Optimization: Making the most of what is available, including human resources, materials, and technology, can mitigate the limitations imposed by a tight budget.
- Risk Assessment: Investing in risk assessment tools can help foresee potential budgeting issues, allowing teams to proactively address them before they spiral.
Effective management of finances can be the difference between succeeding and struggling in the implementation of engineering projects, influencing not only the immediate outcome, but also the organization's long-term viability. Keeping the fiscal ship afloat is as important as navigating through the waters of technology and innovation.
Future Trends in Sistema Ingenieria
The landscape of Sistema Ingenieria is continuously evolving, shaped significantly by emerging trends and the necessity for innovation. Future trends are essential because they pave the way for advancements in methodologies, enhance existing processes, and bring forth new ideas that are crucial for the growth of system engineering. By keeping an eye on these trends, professionals can align their strategies and practices to remain competitive and effective in their work.
Emerging Technologies and Their Impact
Emerging technologies such as the Internet of Things (IoT), blockchain, and advanced data analytics are increasingly making their mark in system engineering. These tools are not just fads; they redefine the way we approach complexity and integration in system design.
For instance, IoT allows for increased connectivity among system components, leading to more robust data collection and analysis. This results in smarter systems that can adapt and respond in real time. The incorporation of blockchain technologies is revolutionizing the trust and validation processes in system integration, enabling secure sharing of information across platforms.
Moreover, with advanced data analytics, engineers can uncover patterns and insights that drive more informed decisions. This application contributes significantly to understanding user needs and expectations, thus tailoring solutions that are more aligned with market demands.
"Staying ahead of technological advancements is not just about adaptation, it's about mastery."
Sustainability in System Engineering
Sustainability has emerged as a master key in modern engineering practices. As the world shifts toward greater environmental consciousness, system engineering must also take significant strides in promoting sustainable solutions. This involves integrating eco-friendly practices at every step of the system life cycle, from conception to decommissioning.
Incorporating sustainability means evaluating materials, energy sources, and the impact of the entire system on the environment. For example, focusing on renewable resources and minimizing waste can lead to more efficient systems that are not only better for the environment but also more cost-effective in the long run. Furthermore, sustainability considerations often lead to innovative design solutions that can enhance performance while reducing environmental footprints.
Incorporating such practices not only aligns with global sustainability goals but also appeals to stakeholders increasingly concerned about corporate responsibility—a trend that is not going away anytime soon.
The Role of Artificial Intelligence
Artificial Intelligence (AI) is not just the latest buzzword; it's becoming integral to the future of system engineering. The capacity for AI to process vast amounts of data and learn from it can drastically enhance various aspects of system design and development. From predictive analytics to decision-making processes, AI introduces efficiencies that were previously unachievable.
For instance, in system monitoring, AI can predict failures before they occur by analyzing data trends. This predictive maintenance reduces downtime significantly and saves costs in repairs. In project management, AI tools streamline workflows and enhance collaboration among teams.
Additionally, as artificial intelligence confers capabilities to simulate scenarios, system engineers can explore numerous potential outcomes before committing resources to a project, thus improving risk management.
As system engineering continues to evolve, incorporating AI will not only be beneficial but essential to stay competitive in the field. The agility, efficiency, and foresight offered by AI make it a cornerstone in shaping the future of this discipline.
End
The importance of concluding this article on Sistema Ingenieria cannot be overstated. As we have explored its foundations and applications, it has become clear that system engineering is not just a discipline but a vital framework that shapes the way we understand and address complex problems across various industries.
Summary of Key Insights
Our journey has taken us through various facets of system engineering, allowing us to uncover several key insights:
- Interdisciplinary Nature: System engineering is inherently interdisciplinary, integrating knowledge from fields such as software engineering, project management, and even psychology. This diverse approach equips professionals with a holistic understanding of the challenges they face.
- Life Cycle Focus: The emphasis on the entire life cycle of a system—from conception to retirement—underscores the need for thoughtful planning and execution. By implementing a comprehensive life cycle perspective, projects can identify potential pitfalls early on, saving time and resources.
- Stakeholder Engagement: Involving stakeholders at every stage is not just beneficial; it’s essential. Effective communication and understanding of stakeholder needs lead to better alignment and satisfaction, reducing friction later in the process.
As highlighted in the article, these insights serve as a foundation for unlocking potential in system engineering and fostering innovation.
"Understanding the dynamics of system engineering is like holding a roadmap in an ever-changing landscape—it provides direction when every road seems to lead to confusion."
Implications for Future Research
Looking ahead, several areas present fertile ground for future research within system engineering:
- Adoption of Emerging Technologies: There’s a pressing need for research on how technologies like blockchain and quantum computing can integrate into existing frameworks. Understanding these impacts can lead to transformative changes in efficiency and security.
- Sustainability Practices: Research on sustainable practices in system engineering is increasingly relevant. Identifying methodologies that incorporate environmental factors can drive not only compliance but also innovation.
- AI Integration: As artificial intelligence becomes more prominent, its role in automating processes and enhancing decision-making warrants deeper exploration. Future studies may focus on effective implementation strategies and the ethical considerations that arise.
Encouraging a culture of inquiry in these areas will enhance the discipline’s adaptability and future-proof its methodologies.