Understanding the Spectrum: In-Depth Analysis and Insights
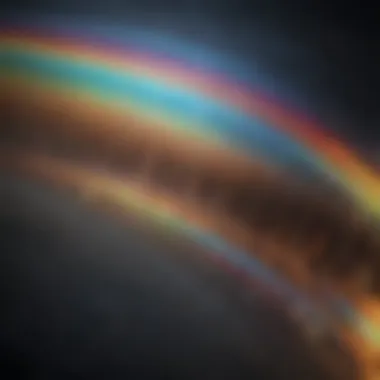
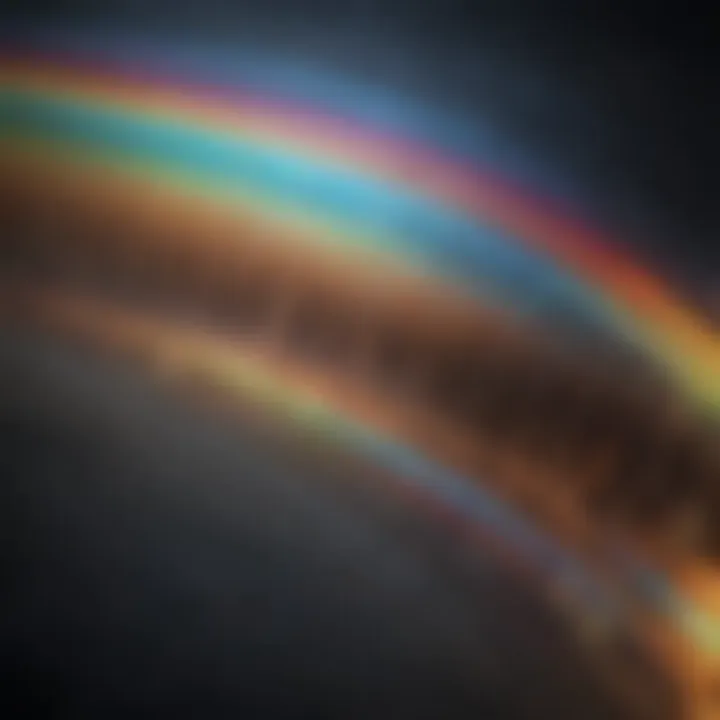
Intro
The study of the spectrum is essential in comprehending various scientific phenomena and applications. It serves as the foundation for many advanced fields, including physics, biology, and technology. Understanding how different types of spectra function and their implications can enhance our grasp of the universe.
In this article, we will explore key concepts related to the spectrum. We will touch upon the electromagnetic spectrum, visual spectra, and their applications in real-world scenarios. By synthesizing current research and advancements, we aim to provide a thorough understanding that appeals to students, researchers, educators, and professionals alike.
Research Overview
Summary of Key Findings
Recent findings in spectral analysis reveal critical insights into how light interacts with matter. For example, studies show that specific wavelengths can influence biological processes significantly. In technology, advancements in spectroscopy have paved the way for enhanced imaging techniques and materials analysis. The ability to analyze spectra brings clarity to theories concerning energy transfer and molecular structure.
Importance of the Research in Its Respective Field
The significance of this research spans various domains. In environmental science, for instance, spectral data is fundamental for assessing climate change impacts. In medicine, understanding spectrums enables better diagnostic tools, like MRI and CT scans.
Moreover, these connections illustrate how theoretical frameworks inform practical innovations that shape our understanding of both the micro and macro cosmos.
Methodology
Description of Experimental or Analytical Methods Used
Different methods are employed to analyze spectra, depending on the type. For electromagnetic spectrum studies, spectrometers are common tools. They allow scientists to observe and record light interaction with materials. In biological research, techniques like fluorescence microscopy are used to study how organisms react under various light conditions.
Sampling Criteria and Data Collection Techniques
Data collection typically involves selecting a representative sample. For instance, environmental studies may focus on specific geographical locations. This ensures that the resulting data reflects broader trends. Furthermore, the choice of sampling is crucial in reducing biases and enhancing the reliability of the findings.
"The spectrum reveals much about the foundational structure of our universe, from tiny atoms to vast galaxies."
Prolusion to the Spectrum
The notion of spectrum bridges numerous disciplines, serving as a crucial lens through which we can appreciate and understand many phenomena. It encompasses various forms of waves and particles that fundamentally shape our perception and interaction with the world. Understanding the spectrum illuminates its role in multiple domains, from physics to biology.
This introduction serves to lay the groundwork for a deeper exploration into spectral phenomena. By defining what a spectrum is and tracing its historical roots, we can appreciate its evolution and significance. This segment encapsulates essential elements that will persist throughout the article, highlighting how spectra inform our understanding of science and technology.
Definition of Spectrum
At its core, the spectrum refers to a range of values or properties that can be measured and analyzed. In a scientific context, it usually pertains to the distribution of different forms of energy, such as light or sound. For example, when light passes through a prism, it disperses into various colors. This phenomenon illustrates the electromagnetic spectrum, showcasing how different wavelengths correspond to colors.
The term can apply to several areas, including:
- Electromagnetic Spectrum: This includes radio waves, microwaves, infrared waves, visible light, ultraviolet light, X-rays, and gamma rays. Each portion of this spectrum exhibits unique characteristics and applications.
- Acoustic Spectrum: It encompasses sound waves, infrasound, and ultrasound, which serve diverse roles in communication and medical imaging.
- Optical Spectrum: Focusing on visible light, it explains how we perceive color and its applications in art and design.
Defining the spectrum provides a common language across these varied fields, enabling scientists and researchers to build on one another's work.
Historical Context
Historically, the exploration of the spectrum dates back centuries. One of the earliest documented studies arose from Isaac Newtonโs experimentation with prisms in the late 17th century. He discovered that white light could be separated into various colors, laying the foundation for modern optics.
As scientific knowledge advanced, subsequent contributors refined the understanding of the electromagnetic spectrum. James Clerk Maxwell, in the 19th century, established the theoretical framework around electromagnetic radiation, leading to groundbreaking developments in telecommunications and imaging technologies.
The 20th century yielded further insights, with the advent of technologies designed to analyze different spectra. Instruments such as spectrometers emerged, enabling researchers to probe the mysteries of both distant celestial bodies and minute biological structures.
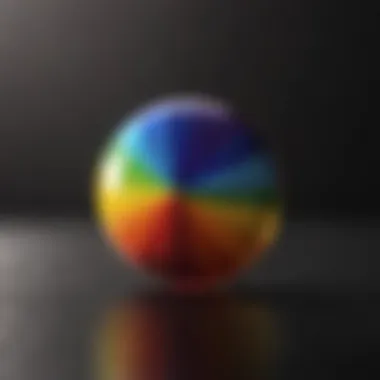
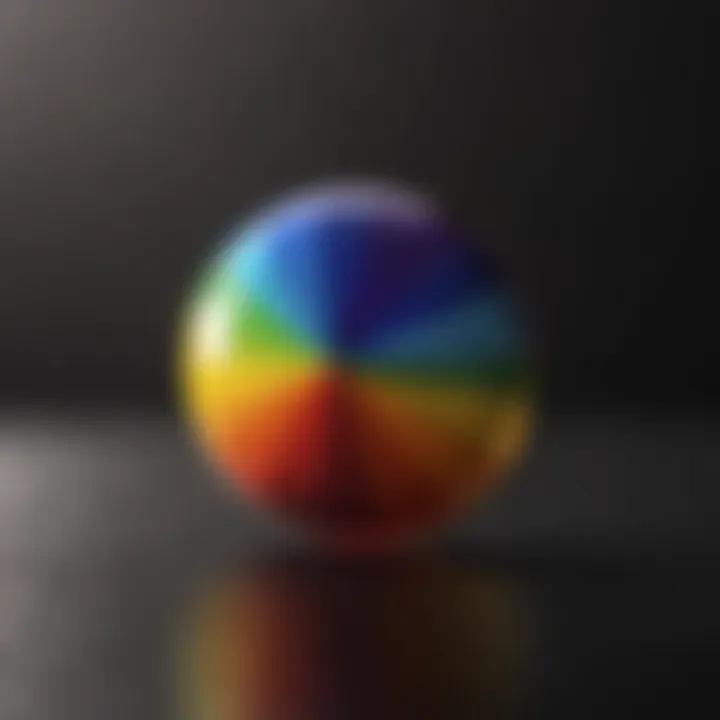
Understanding this historical backdrop enhances our appreciation for the ongoing advancements in spectral analysis. This provides context for the later discussions in this article related to current applications and future research in spectrum studies.
Types of Spectra
The study of types of spectra is crucial for comprehending the intricate relationships present in both natural and technological contexts. Each type of spectrum carries distinct characteristics that contribute to various applications across fields, from telecommunications to environmental science. Understanding these types allows researchers and professionals to utilize spectral analysis effectively, making it possible to design solutions tailored to specific challenges.
Electromagnetic Spectrum
The electromagnetic spectrum encompasses a range of waves, from low-frequency radio waves to high-energy gamma rays, allowing for various significant applications throughout different disciplines.
Radio Waves
Radio waves are the longest wavelengths in the electromagnetic spectrum, extending from about 30 centimeters to several kilometers. Their ability to travel long distances and penetrate various materials makes them ideal for communication technologies such as television, radio broadcasting, and mobile phones. The key characteristic of radio waves is their capacity to carry information over vast distances, making them a popular choice in the telecommunications sector. However, their relatively low energy compared to other types of waves means they require significant power to transmit over extensive areas.
Microwaves
Microwaves fall between radio waves and infrared waves in terms of frequency and wavelength. They are utilized in various applications, including cooking and communication. One of the unique features of microwaves is their ability to penetrate food, causing polar molecules within to vibrate and produce heat. This property is what makes microwave ovens highly efficient. On the downside, while microwaves can penetrate clouds and light rain, heavy precipitation can hinder their transmission, which is a consideration for communication systems.
Infrared Waves
Infrared waves are at the lower end of the electromagnetic spectrum and are primarily associated with heat. Infrared technology has numerous applications, from night vision equipment to remote controls. A key characteristic of infrared waves is their emission from objects based on temperature, which is critical for thermal imaging. However, their effectiveness diminishes with distance, making them less suited for long-range communication but perfect for localized applications such as sensing and imaging.
Visible Light
Visible light, the section of the spectrum that the human eye can perceive, ranges from violet to red. Its significance in this article stems from its central role in various fields, including optics and color science. The unique feature of visible light is its ability to interact with matter in ways that are crucial for seeing and understanding the environment. While crucial for illumination and vision, the visible light spectrum is limited to a narrow range of wavelengths, which restricts its applications compared to its broader counterparts.
Ultraviolet Light
Ultraviolet light, situated just beyond visible light, has significant relevance in both natural and artificial scenarios. Its key characteristic lies in its capacity to cause chemical reactions, which is essential for processes like photosynthesis and sterilization. Ultraviolet light is a beneficial choice for disinfection purposes due to its germicidal properties. However, exposure to high levels of ultraviolet can lead to negative health effects, including skin cancer, thus posing a challenge in its practical use.
X-rays
X-rays have earned their reputation primarily in medical settings, where they are utilized for imaging purposes. The key aspect of X-rays is their penetrating power, allowing them to pass through soft tissues while being absorbed by denser materials like bones. This unique capability is invaluable in diagnostics. However, the exposure to X-rays carries the risks associated with ionizing radiation, necessitating strict safety measures during their usage.
Gamma Rays
Gamma rays represent the highest frequency and energy levels within the electromagnetic spectrum. Their capacity to penetrate matter makes them significant in fields such as radiation therapy for cancer treatment. The unique feature of gamma rays is their high energy, which allows them to disrupt atomic structures, making them effective for medical applications. Nonetheless, the destructive potential of gamma rays also poses safety challenges, as their exposure can be extremely harmful to living organisms.
Acoustic Spectrum
The acoustic spectrum includes waves that travel through air and other mediums, contributing significantly to communication and environment assessment. Understanding sound waves and their properties enhances various applications, such as audio technology and wildlife monitoring.
Sound Waves
Sound waves are essential for communication and perception within our environment. These waves travel via vibrations through different mediums, making them a valuable resource in audio technologies. The primary characteristic of sound waves is their ability to be modulated, allowing for speech, music, and various technologies like ultrasound. However, sound waves are subject to damping, meaning they lose energy over distance, which can limit their effective range.
Infrasound
Infrasound consists of sound waves with frequencies below the audible range for humans. Their unique capacity to travel long distances without significant loss of energy makes them useful for monitoring natural phenomena, such as earthquakes or volcanic activity. The benefits of using infrasound involve its sensitivity and ability to provide early warnings for various natural disasters. However, the challenge lies in the technical demand for infrasound detection equipment, which may not be readily available everywhere.
Ultrasound
Ultrasound refers to sound waves with frequencies above the upper limit of human hearing. Commonly used in medical imaging, its non-invasive nature is a prime advantage in patient care. Ultrasound waves can create detailed images of soft tissues and structures within the body. On the downside, the effectiveness of ultrasound can diminish based on the type of tissue being imaged and the presence of air, which can limit its application in some scenarios.
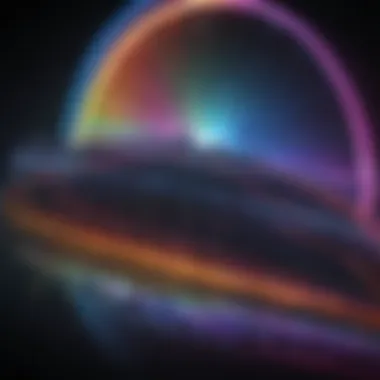
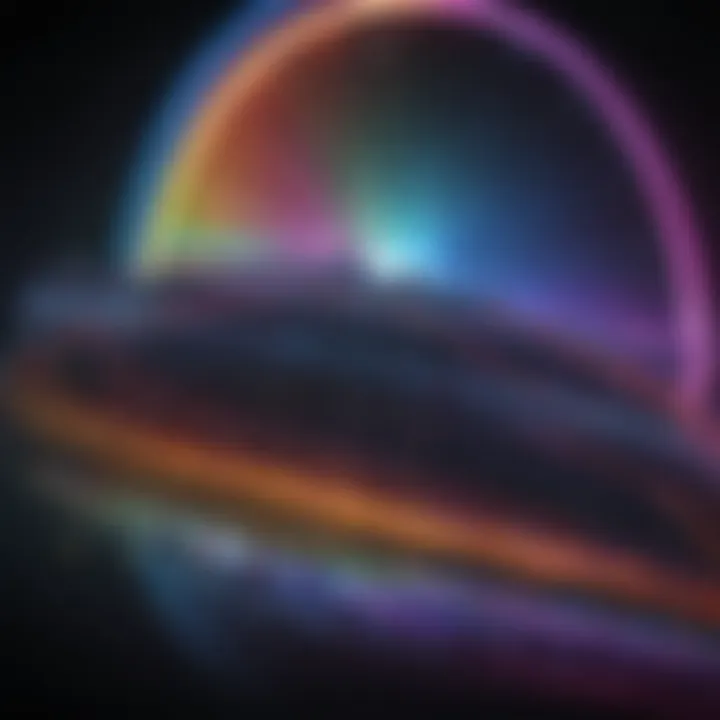
Optical Spectrum
The optical spectrum focuses on the wavelengths that are visible to the human eye and how they interact with various materials. This section encompasses understanding color perception and its applications in fields like art, design, and technology.
Color Perception
Color perception plays a significant role in human experience and sensory analysis. It is defined by the wavelengths of light that are interpreted by the brain. A key feature of color perception is its subjectivity; different people may perceive colors differently. This characteristic is essential in various applications, including visual art and marketing. However, the variations in perception can complicate color management across different media.
Applications in Art and Design
The application of optical principles in art and design showcases the practical use of the optical spectrum. Artists rely on color theory to create visual harmonies and contrasts in their work, while designers apply these principles to enhance user experience in products. The unique aspect of applying color theory is its capacity to influence emotions and perceptions, which is a critical consideration in effective design. Although beneficial, the subjective nature of color can present challenges when aiming for a specific aesthetic or message.
Spectroscopy: Techniques and Tools
Spectroscopy serves as a fundamental technique in various scientific disciplines. It allows researchers to study the interaction between matter and electromagnetic radiation to understand the structure and dynamics of substances. The exploration of spectroscopy techniques and tools provides essential insights for chemists, physicists, and engineers, facilitating their work in areas such as material science, biology, and astronomy. This section will elucidate the basic principles behind spectroscopy and outline prominent types, emphasizing their significance in advancing research and practical applications.
Basic Principles
At its core, spectroscopy is predicated on the absorption, emission, or scattering of light by materials. When a substance interacts with light, it can absorb certain wavelengths, which corresponds to specific energy transitions within the molecular or atomic structure. Thus, by analyzing the light that is transmitted or emitted, scientists can infer the composition and properties of the sample.
The fundamental concept revolves around the relationship between wavelength and the energy states of electrons. Each element has a unique spectral signature, acting as a fingerprint. In practical terms, this means that spectroscopy can provide detailed information about material composition, molecular structures, and concentrations, fundamentally aiding qualitative and quantitative analyses.
Types of Spectroscopy
UV-Vis Spectroscopy
UV-Vis Spectroscopy, or Ultraviolet-Visible Spectroscopy, is essential for studying materials that absorb light in the ultraviolet and visible ranges of the electromagnetic spectrum. It is especially noted for its simplicity and high sensitivity, making it a preferred method in both research and industry. One of the key characteristics of UV-Vis Spectroscopy is its ability to determine the concentration of a substance within a solution through Beer's Law.
The unique feature of UV-Vis Spectroscopy is its capacity to yield rapid results, which is invaluable in time-sensitive analyses. However, it does have limitations; for example, it is not as effective for analyzing complex mixtures or samples that have overlapping absorption bands. Overall, its advantages in straightforward applications render it a popular choice in chemical analysis and quality control.
Infrared Spectroscopy
Infrared Spectroscopy is particularly useful for identifying molecular structures by detecting specific vibrational transitions. This technique shines in organic chemistry and materials analysis, where it helps characterize functional groups within molecules. The significant characteristic of Infrared Spectroscopy is its non-destructive nature, which allows for the analysis of samples without altering their physical state.
A unique advantage of Infrared Spectroscopy is its effectiveness in identifying bonds within molecules, thus providing critical information about molecular composition. Nevertheless, its reliance on sample purity can pose challenges, as impurities can significantly affect the results. Its precise insights into molecular structures make it a powerful tool for chemists and researchers.
Nuclear Magnetic Resonance Spectroscopy
Nuclear Magnetic Resonance (NMR) Spectroscopy is a sophisticated technique that exploits the magnetic properties of certain atomic nuclei. This method is crucial in providing insight into the structure of organic compounds and is particularly noted for its ability to furnish detailed information about the molecular environment. A key advantage of NMR Spectroscopy is its capacity to elucidate three-dimensional structures through chemical shifts and coupling constants.
What sets NMR apart is its comprehensive nature, allowing scientists not only to determine the identity of compounds but also to analyze their dynamics. Despite requiring more complex instrumentation and longer analysis times than UV-Vis and Infrared Spectroscopy, the structural clarity and depth of information provided make NMR Spectroscopy an indispensable method in both academic research and pharmaceuticals.
"Spectroscopy techniques are foundational to understanding substance interactions and characteristics, making them vital to many scientific fields."
In summary, the exploration of spectroscopy techniques and tools is essential for numerous scientific applications. Each type of spectroscopy, from the rapid analyses of UV-Vis to the intricate details provided by NMR, contributes uniquely to the broader understanding of chemical and physical properties of materials. This section underlines how essential these techniques are in advancing not only academic research but also practical applications in industries worldwide.
Applications of Spectral Analysis
Spectral analysis plays a crucial role in various scientific domains. It provides methods to analyze light and sound, offering insights into material composition, astronomical phenomena, and environmental conditions. The benefits of applying spectral analysis extend beyond academic theory and into practical implications that affect everyday life. With technologies advancing rapidly, the integration of spectral techniques continues to reshape industries such as chemistry, astronomy, and environmental science.
Spectroscopy in Chemistry
Spectroscopy is indispensable in the field of chemistry. It enables chemists to identify and quantify substances within a mixture, enhancing the understanding of molecular structures. Techniques like UV-Vis and infrared spectroscopy allow for direct observation of electronic and vibrational transitions. For example, UV-Vis spectroscopy can determine the concentration of a substance by measuring how much light it absorbs at specific wavelengths. This is crucial for applications ranging from drug discovery to quality control in manufacturing processes.
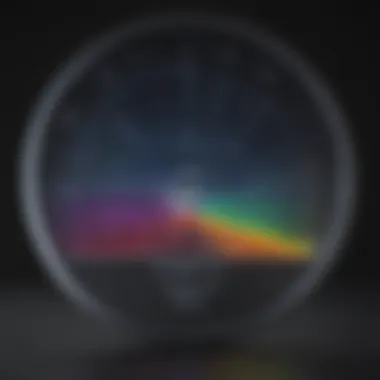
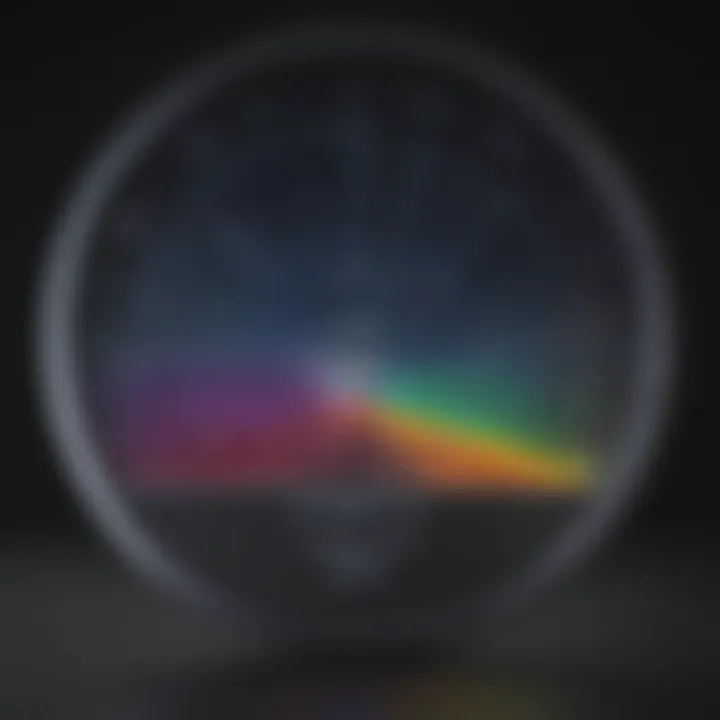
Key points related to spectroscopy in chemistry include:
- Molecular Identification: Different compounds absorb light at distinct wavelengths, providing a unique spectral fingerprint.
- Concentration Analysis: Quantitative analysis can be accurately performed using Beer's Law, relating absorbance to concentration.
- Reaction Monitoring: Spectroscopic techniques allow researchers to monitor chemical reactions in real-time, identifying products as they are formed.
Role in Astronomy
Astronomy heavily relies on spectral analysis to uncover the universe's secrets. Astronomers analyze the light emitted or absorbed by celestial bodies to determine their composition, temperature, distance, and velocity. Spectroscopy has led to groundbreaking discoveries, including the identification of the chemical elements in stars and galaxies.
Important aspects of spectral analysis in astronomy include:
- Stellar Composition: Analyzing the spectra of stars reveals elements such as hydrogen, helium, and heavier metals, aiding in understanding stellar evolution.
- Redshift Measurement: Spectroscopic data on light from distant galaxies allow astronomers to measure their velocity and infer the universe's expansion.
- Exoplanet Study: Transit spectroscopy can detect the atmospheres of exoplanets, providing critical information about their potential habitability.
Environmental Monitoring
The implications of spectral analysis extend to environmental monitoring as well. By analyzing light absorption patterns in the atmosphere and oceans, scientists can assess pollution levels and climate change impacts. Techniques like remote sensing utilize spectral data to gather information on land use and ecosystem health.
Key considerations for environmental monitoring include:
- Pollution Detection: Specific wavelengths can indicate the presence of pollutants, enabling timely intervention and policy development.
- Climate Change Tracking: Spectroscopy helps measure greenhouse gas concentrations, contributing to more accurate climate models.
- Biodiversity Assessment: Monitoring vegetation health through spectral reflectance informs conservation efforts and agriculture practices.
In summary, the applications of spectral analysis are extensive and varied, touching upon essential aspects of scientific inquiry and practical problem-solving across multiple fields.
The Future of Spectrum Research
The realm of spectrum research is rapidly evolving, propelled by advancements in technology and interdisciplinary communication. This section outlines the numerous facets of research that are anticipated to shape future developments in spectral analysis. Understanding these trajectories is essential, as they hold the key to new applications and insights in various fields including physics, chemistry, biology, and engineering.
Innovations in Technology
Technological innovations are fundamental in driving the future of spectrum research. New tools and techniques enhance both the speed and precision of spectral analysis. For instance, the advent of high-resolution spectroscopy improves data acquisition, allowing scientists to analyze finer spectral details. This capability can facilitate discoveries in material sciences, where understanding electronic transitions is crucial.
Moreover, advancements in computational algorithms have paved the way for more sophisticated analysis of spectral data. Machine learning and artificial intelligence can identify patterns in large datasets that may not be evident to human analysts. This may lead to breakthroughs in various domains such as drug discovery where specific molecular interactions can be analyzed through their spectral fingerprints.
"Emerging technologies in spectrometry can transform industries, enhancing both research capabilities and practical applications."
Interdisciplinary Collaborations
The future of spectrum research is not confined to a single discipline. Interdisciplinary collaborations are emerging as a vital component in advancing our understanding of spectral phenomena. Involving experts from fields such as data science, environmental science, and even social sciences leads to a holistic approach in spectral research. For instance, collaborations between chemists and data scientists can yield efficient models for predicting molecular behaviors through spectral analysis.
Moreover, as global challenges such as climate change require multifaceted solutions, the role of spectral analysis in environmental monitoring becomes increasingly significant. By integrating knowledge from remote sensing, oceanography, and atmospheric sciences, researchers can leverage spectral data to devise solutions that inform policy and conservation efforts.
These collaborative efforts demonstrate that spectrum research is not an isolated endeavor; it relies heavily on the interconnections between various domains of knowledge. The synthesis of diverse perspectives can enhance both theoretical understanding and practical outcomes.
Ending
The conclusion section serves a pivotal role in consolidating the core elements highlighted throughout this article. It acts as a synthesis of intricate spectra concepts explored, offering clarity on their significance in both scientific inquiry and practical applications. As various disciplines converge on the common theme of the spectrum, understanding its nuances becomes vital. Key insights gathered throughout our journey encompass the multifaceted nature of spectra, which ranges from the electromagnetic spectrum to visual perception and the acoustic realm. Each type of spectrum offers insights that are not only theoretically rich but also applicable to a myriad of fields including chemistry, astronomy, and environmental sciences.
Summarizing the main takeaways is essential in reinforcing the knowledge gained. Each section addresses unique aspects of the spectrum: its definition, historical context, types, applications, and future trends in research. By reflecting on these points, we encourage readers to appreciate how they interact with the fundamental laws of the universe. The relevance of spectral studies is underscored by their implications for technological progress and scientific understanding, highlighting the necessity of continued exploration in this arena.
Summarizing Key Insights
- Diversity of Spectra: From electromagnetic to acoustic, various spectra offer critical insights about different forms of energy and matter.
- Tools of Spectroscopy: Techniques such as UV-Vis spectroscopy and nuclear magnetic resonance provide indispensable methods for analyzing materials across disciplines.
- Real-World Applications: The significance of spectral analysis extends into everyday scenarios, influencing technology and environmental monitoring.
- Interdisciplinary Impact: Spectra connect multiple fields, fostering innovations through collaborative research efforts and improved methodologies.
- Future Research Directions: Emerging technologies will likely propel research further, unearthing more discoveries within spectral sciences.
The Importance of Continued Exploration
The necessity for ongoing research in the field of spectrum cannot be overstated. As we observe rapid advancements in technology, the potential for new applications and understandings of spectrum broadens. Continuous inquiry not only empowers scientists and engineers but also enhances public comprehension of scientific concepts.
Furthermore, the interconnected nature of different spectra demands a holistic exploration to discover overlaps and synergies. For instance, advancements in the understanding of electromagnetic properties can significantly contribute to enhancements in acoustic technologies.
By supporting new research initiatives, funding interdisciplinary collaborations, and fostering educational programs focused on spectral understanding, we can ensure that these concepts are not only preserved but also expand with time. With each advancement, we must remain vigilant, assessing how new insights apply to issues facing society in real-time, from climate change to public health, showcasing how fundamental research leads to tangible advantages in our world.
"Scientific discoveries are rarely isolated; they build on each other, creating a cumulative understanding that drives progress across disciplines." - Anonymous