Understanding tRNA and Codon Interaction in Protein Synthesis
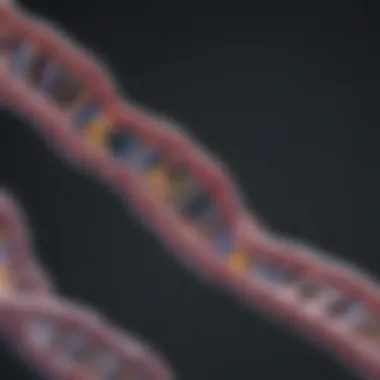
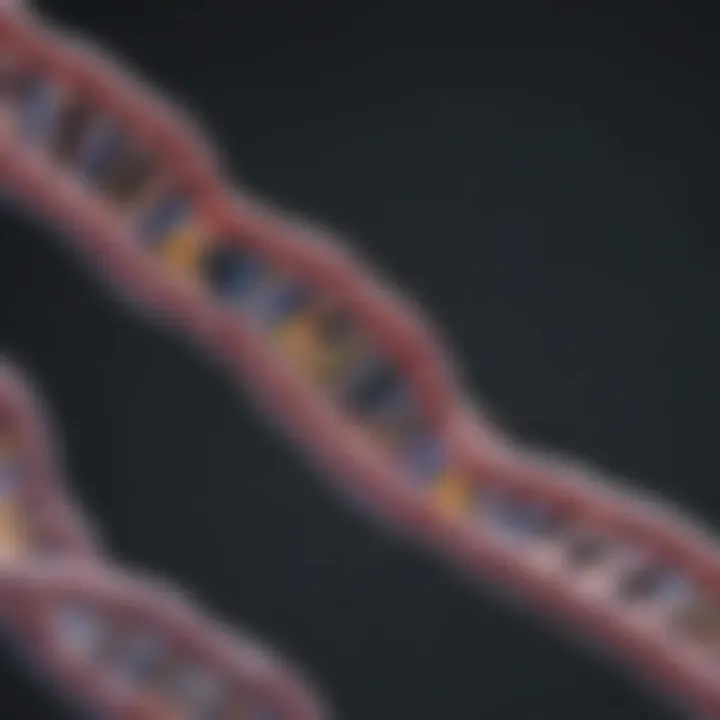
Intro
The process of protein synthesis is fundamental to all living organisms. In this intricate machinery, transfer RNA (tRNA) plays a critical role. tRNA serves not only as a transport vehicle for amino acids but also as a key player in the translation phase of gene expression. The interaction between tRNA and codons, which are sequences on messenger RNA (mRNA), is crucial for the accurate assembly of proteins.
Codons in mRNA dictate the sequence of amino acids in a protein. Each codon corresponds to a specific amino acid, and tRNA possesses the capability to recognize these codons through its anticodons. This relationship drives the fidelity and efficiency of translation. Understanding tRNA and codon interaction is essential for elucidating broader biological concepts, including molecular mechanisms of heredity, genetic variation, and the effects of mutations.
In the sections that follow, this article will provide a detailed examination of the mechanisms that underline tRNA functionality, the significance of its modifications, and the impact of codon usage bias on protein synthesis.
Preface to tRNA and Codons
The interplay between transfer RNA (tRNA) and codons represents a foundational aspect of molecular biology. Understanding this interaction is crucial for grasping how proteins are synthesized in living organisms. This section elucidates the significant roles tRNA and codons play in translating genetic information into functional proteins. The import of tRNA extends beyond its structural designation; it is integral to the accuracy and efficiency of protein synthesis.
Historical Context
The journey of discovering tRNA and codons illuminates the evolution of molecular biology. In the early 1960s, researchers began to understand the central dogma of molecular genetics, which posits that DNA is transcribed into RNA, and subsequently, RNA is translated into proteins. The identification of tRNA as the molecule responsible for carrying amino acids to the ribosome laid the groundwork for understanding translation. Insights from early experiments also led to the realization that codons, sequences of three nucleotides in mRNA, provide the necessary information to dictate amino acid selection during protein synthesis. This historical backdrop hones in on the relentless curiosity that drives scientific inquiry.
Basic Structure of tRNA
tRNA molecules exhibit a unique cloverleaf structure, which is crucial for their function. Each tRNA has specific regions that allow it to interact with both mRNA and amino acids. At one end, there is an anticodon, a triplet of nucleotides that complementarily pairs with the codon on mRNA. At the opposite end, the tRNA binds to a specific amino acid via an ester linkage. This structure ensures that tRNA can fulfill its role in translation correctly. The sequence and arrangement of nucleotides contribute significantly to the capability of tRNA in recognizing codons and facilitating accurate translation.
The Concept of Codons
Codons are the essential building blocks of the genetic code. Each codon consists of three nucleotides, and they correspond to specific amino acids or serve as stop signals during protein synthesis. The total number of codons is 64, which includes 61 codons for amino acids and 3 stop codons. The redundancy in the genetic code, known as degeneracy, means that multiple codons can encode the same amino acid. This characteristic provides a buffer against mutations, as changes in the DNA sequence may not always lead to alterations in the protein sequence. Understanding codons is fundamental for comprehending how genetic information translates into the vast diversity of proteins essential for life.
Functionality of tRNA
The functionality of transfer RNA (tRNA) is vital in bridging the information encoded in messenger RNA (mRNA) to the actual proteins synthesized in the cell. Understanding how tRNA operates is important for several reasons. It highlights the mechanisms by which genetic information is interpreted and translated into proteins, which are essential for all cellular functions. This section dissects the three main roles of tRNA: transportation of amino acids, recognition of codons, and the formation of the aminoacyl-tRNA complex.
Transportation of Amino Acids
One of the primary functions of tRNA is transporting amino acids to the ribosome, where protein synthesis occurs. Each tRNA molecule is specific to one amino acid. The amino acid is attached to the 3' end of the tRNA molecule, facilitated by enzymes known as aminoacyl-tRNA synthetases. These enzymes play a crucial role in ensuring that the correct amino acid is linked to its corresponding tRNA, which is essential for the accuracy of protein synthesis. Any error in this process could lead to malformed proteins and cellular malfunction.
The specificity in amino acid attachment is not arbitrary. It involves a distinct interaction between the tRNA and the aminoacyl-tRNA synthetase, ensuring that the right amino acid is transported during translation. This fidelity in amino acid transportation underscores the larger picture of how proteins are synthesized accurately in accordance with the genetic code.
Recognition of Codons
Recognition of codons is another significant role of tRNA. A codon is a sequence of three nucleotides in mRNA that corresponds to a specific amino acid. tRNA has a complementary sequence called an anticodon, which pairs with the codon in the mRNA during translation. This base pairing is crucial for the legitimate synthesis of proteins, as it ensures that the appropriate amino acids are added in the correct order.
The interaction between the codon and anticodon involves specific base pairing rules, primarily adenine pairing with uracil, and cytosine pairing with guanine. This specificity is how the tRNA accurately 'reads' the genetic code presented in the mRNA. Any mismatch could potentially alter the resulting protein, leading to changes in function or loss of activity.
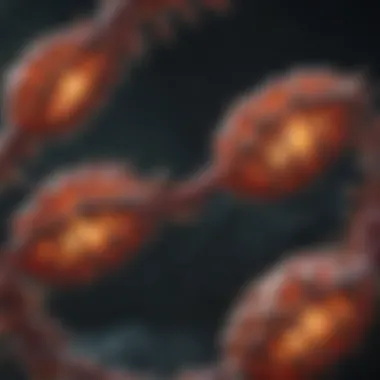
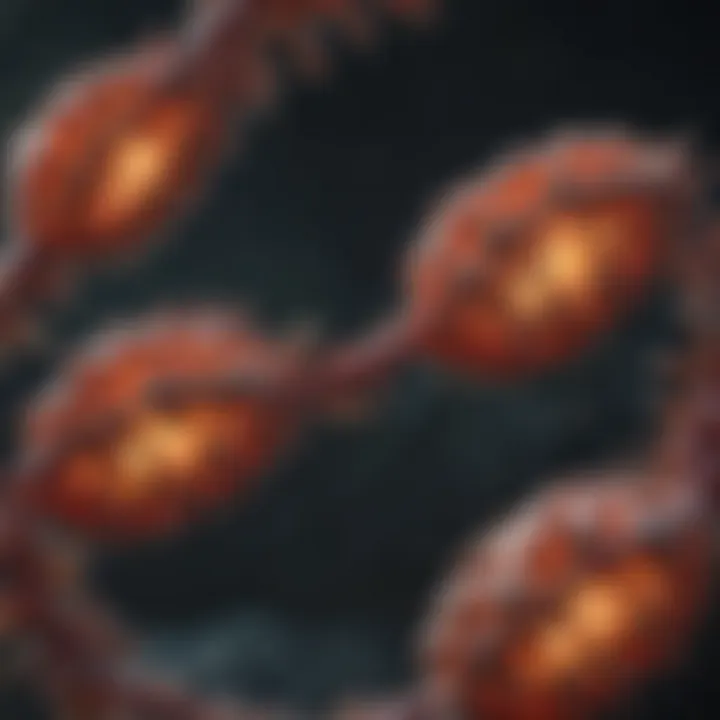
Formation of the Aminoacyl-tRNA Complex
The formation of the aminoacyl-tRNA complex is the final step in preparing tRNA for its role in protein synthesis. This complex forms when an amino acid is joined to its corresponding tRNA, resulting in an activated molecule that can participate in the process of translation. The aminoacyl-tRNA complex exists in a state that is ready to enter the ribosome for protein synthesis.
In summary, the functionality of tRNA encompasses critical processes in molecular biology. From transporting amino acids to accurately pairing codons with anticodons, tRNA is central to the efficacy of protein synthesis. The precise formation of the aminoacyl-tRNA complex ensures that this translation process is effective and reliable, contributing to the overall understanding of genetic expression.
"The efficiency and accuracy of codon-tRNA interactions lay the foundation for the integrity of the proteome."
Understanding these aspects reveals the intricate ballet of cellular machinery, emphasizing the importance of tRNA in the world of molecular biology.
Codon-anticodon Interaction
The interaction between codons and anticodons is central to the process of protein synthesis. This interaction ensures that the correct amino acid is added to the growing polypeptide chain in response to the sequence of nucleotides in messenger RNA (mRNA). By facilitating accurate translation, codon-anticodon pairing underlies the transition from genetic information to functional proteins.
Mechanism of Interaction
The molecular mechanism behind codon-anticodon interaction is intricate. When mRNA is being translated by ribosomes, the tRNA molecule, which carries a specific amino acid, must recognize the corresponding codon in the mRNA sequence. This recognition occurs at the ribosome's active site, where the anticodon of the tRNA binds to the complementary codon sequence on the mRNA.
Each tRNA molecule has a unique anticodon triplet that pairs with its respective codon via hydrogen bonds. The binding will only occur if the codon and anticodon are complementary. This specificity is crucial as it ensures that the right amino acid is incorporated into the growing protein chain. This process requires the presence of protein factors and ATP, which facilitate the binding and subsequent release of tRNA following peptide bond formation.
Importance of Base Pairing
Base pairing is vital in ensuring the fidelity of protein synthesis. The complementarity between codons and anticodons (A-U, and G-C) dictates which amino acids are added to the protein. If base pairing were inaccurate, it could lead to the incorporation of incorrect amino acids, resulting in dysfunctional or harmful proteins. Thus, the accuracy of codon-anticodon pairing is directly correlated with the overall efficiency and fidelity of translation.
Importantly, the precise base pairing also assists in stabilizing the tRNA-mRNA interaction, diminishing the chance of errors in translation. This precise alignment ultimately supports the mechanics of the ribosome and influences the rate of protein synthesis.
Accurate codon-anticodon pairing is the backbone of faithful protein synthesis, a process central to all forms of life.
Wobble Hypothesis
The wobble hypothesis provides insight into how a limited number of tRNA molecules can accommodate the broad range of codons found in the genetic code. It proposes that while the first two bases of a codon require strict pairing with the anticodon, the third base may exhibit some flexibility or "wobble." This flexibility allows one tRNA to recognize multiple codons that correlate with the same amino acid.
For instance, the amino acid glycine is encoded by the codons GGT, GGC, GGA, and GGG. A single tRNA, with an anticodon that can wobble, might fit multiple codons that code for glycine. This characteristic significantly reduces the number of tRNA types required within a cell and contributes to the efficiency of the protein translation process. Understanding this mechanism is essential for appreciating how genetic information is accurately translated into functional proteins despite the complexity of codon representation.
Modifications in tRNA
The modifications in transfer RNA (tRNA) play a crucial role in its functionality and efficiency during protein synthesis. Understanding how these modifications occur and their importance can provide valuable insight into the overall process of translation. Without these changes, tRNA would not operate at the necessary accuracy and speed required in cellular systems.
Modifications to tRNA are not simply a biochemical curiosity; they can impact the stability and folding of tRNA molecules, which directly affects their ability to perform their duties. These modifications can also influence the efficiency of codon recognition and the overall fidelity of translation.
Notably, tRNA undergoes various chemical modifications that enhance its functionality. The presence of modified nucleotides often results in improved structural integrity and the ability to form specific interactions with codons in messenger RNA (mRNA). Overall, these modifications have implications on the translational apparatus at numerous levels.
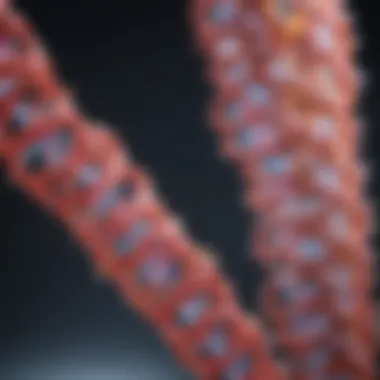
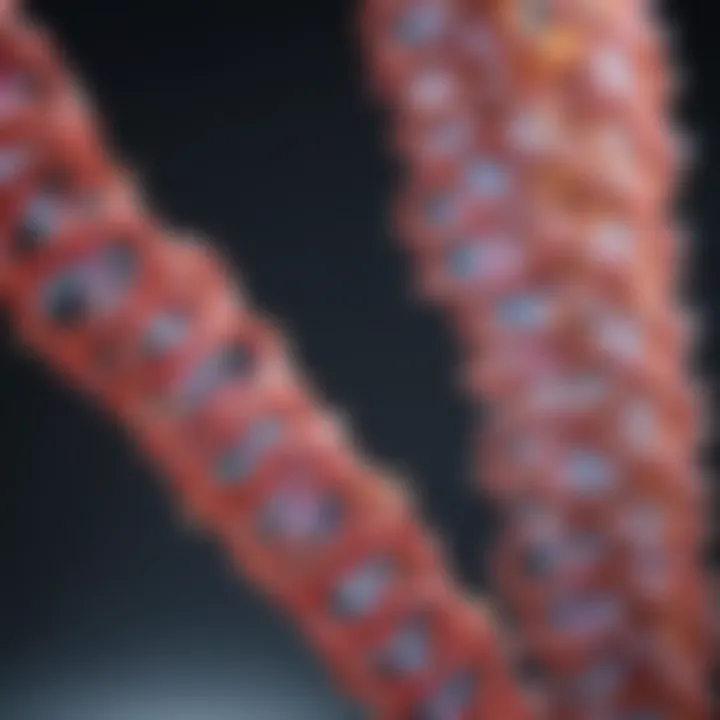
Chemical Modifications of tRNA
Chemical modifications in tRNA include a variety of alterations to the nucleotides. These changes can involve the addition of functional groups, methylation, and even the incorporation of unusual nucleotides. For example, 5-methyluridine, also known as ribothymidine, plays a role in stabilizing the tRNA structure. Furthermore, isopentenyladenosine modifies adenosine in the tRNA sequence and is crucial for proper tRNA function.
Common modifications include:
- Methylation: This addition of methyl groups to nucleotides provides stability and enhances base pairing capabilities.
- Pseudouridylation: Converting uridine to pseudouridine improves the stability of RNA structures.
- Dihydrouridine: This modification aids in the tRNA's ability to bend and form interactions needed for codon-anticodon pairing.
Each modification serves a distinct purpose, ultimately contributing to the efficiency and accuracy of protein synthesis.
Functional Implications of Modifications
The functional implications of chemical modifications can be profound. Modified tRNA can interact more effectively with specific codons in mRNA, leading to improved translational efficiency. For instance, a well-modified tRNA can recognize codons with greater precision, decreasing the chance of errors during protein assembly. This has implications not only for the individual proteins being produced but also for the overall cellular homeostasis.
Additionally, the presence of these modifications can influence the folding of tRNA molecules. Proper folding is essential; it allows for the correct spatial arrangement of the anticodon to match the codon in mRNA. Without these modifications, sloppy folding may lead to mistranslation and could even result in dysfunctional proteins.
In summary, the chemical modifications of tRNA significantly enhance its role in protein synthesis, allowing for proper translation and cellular function.
Understanding these modifications encourages a deeper appreciation of the subtleties involved in molecular biology. In a research setting, these insights could pave the way for innovative therapeutic strategies that address sequences where tRNA function is compromised.
Codon Usage Bias
Codon usage bias is a crucial concept in the understanding of protein synthesis. It refers to the unequal frequency of codons within a gene. Specific organisms have preferences for particular codons, even if those codons code for the same amino acid. This bias can significantly influence several aspects of molecular biology, such as gene expression, translational efficiency, and the overall adaptability of an organism. By examining the reasons behind this phenomenon, one can appreciate its biological relevance.
Definition and Implications
Codon usage bias can best be understood through its definition: it is the tendency of specific codons to be used more frequently than others in the coding sequences of a genome. This preference is not random; it often correlates with the abundance of tRNAs corresponding to those codons. For instance, if a certain codon is used more frequently, it suggests that its associated tRNA is present in higher quantities. This can lead to more efficient protein synthesis, as the ribosome can translate mRNA much faster when matching tRNAs are readily available.
The implications of codon usage bias are extensive. It can affect how genes are expressed in a cell, thereby impacting the overall fitness of the organism in its environment. Different tissues in multicellular organisms may exhibit varying codon biases, affecting the production of proteins essential for specific functions. Furthermore, when genes from one organism are expressed in another, understanding the host organism's codon bias becomes crucial for effective protein expression.
Factors Influencing Codon Usage
The factors that influence codon usage bias are diverse. They include:
- tRNA Availability: The abundance of specific tRNAs in a cell can dictate which codons are favored during translation.
- Selective Pressure: Organisms adapt to their environments. This adaptation can drive a preference for codons that enhance protein performance under certain conditions.
- Gene Function: Genes that are highly expressed, such as those coding for ribosomal proteins, tend to exhibit pronounced codon bias compared to rarely expressed genes.
- GC Content: The nucleotide composition of a genome also plays a role. High GC content can lead to a bias towards codons that correspond to G or C, further influencing the expression patterns of genes.
Understanding these factors is crucial for researchers when modifying genes for different contexts, such as in synthetic biology.
Evolutionary Perspectives
From an evolutionary standpoint, the codon usage bias reflects a deeper connection between organisms and their environmental pressures. Over time, species adapt their genetic coding to optimize protein synthesis. An organism's codon bias may change based on factors like evolution, adaptation, and horizontal gene transfer among species.
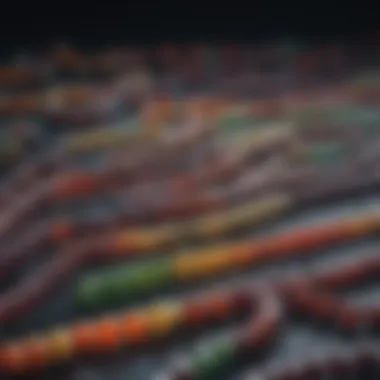
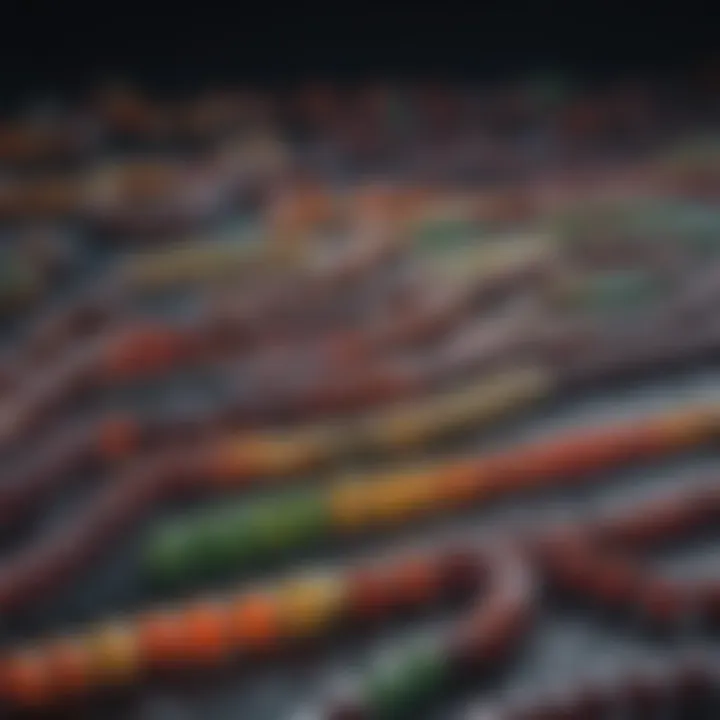
For example, bacteria may exhibit different codon usage biases when exposed to antibiotics, as they adapt to survive. This adaptation can lead to a cumulative effect over generations, influencing not only their genetic makeup but also their ecological interactions.
In summary, codon usage bias serves as an insightful lens through which one can explore molecular biology’s intricacies. Understanding the definitions, implications, factors influencing codon choices, and the evolutionary perspectives of this bias can offer valuable context for students, researchers, and educators alike.
"Codon usage bias reveals the deep relationship between genetic coding and evolutionary adaptation, making it a key for understanding how organisms thrive in diverse environments."
For more details, reference articles on Wikipedia or visit Britannica.
tRNA in Disease and Therapeutics
The role of transfer RNA (tRNA) extends into the realm of disease and therapeutics, illustrating its importance beyond mere protein synthesis. Understanding these dimensions reveals how tRNA influences various biological processes and its potential implications for treating genetic disorders and other diseases.
tRNA and Genetic Disorders
Genetic disorders often arise from mutations that affect the structure or function of tRNA. These mutations can disrupt the proper translation of amino acids, leading to the production of dysfunctional proteins. In some cases, specific tRNA genes are linked to diseases such as mitochondrial disorders and certain types of cancers. The malfunctioning of tRNA can generate a cascade of issues within the cell, affecting metabolic pathways and cellular health.
Addressing these misfolded proteins or improper synthesis pathways offers a potential therapeutic avenue. Research is currently focused on understanding how modifications in tRNA can mitigate the effects of these genetic aberrations. For instance, targeting specific tRNA modifications could lead to enhanced recognition and amino acid incorporation, which may restore cellular functions disrupted by genetic errors.
tRNA as a Therapeutic Target
The therapeutic implications of tRNA are significant. Current trends are exploring the potential for targeting tRNA to treat various diseases. This approach utilizes small molecules or RNA-based therapies that can specifically interact with dysfunctional tRNA molecules. By modulating these interactions, researchers aim to influence protein synthesis outcomes positively.
"Targeting tRNA may offer a refined means of addressing diseases at their molecular roots, beyond traditional therapeutic approaches."
Some examples of therapies rely on the suppression of pathogenic tRNA to inhibit the translation of harmful proteins. This could provide a method for combating diseases caused by specific mutations or overexpressed oncogenes. As the field of RNA therapeutics matures, the focus on tRNA will likely expand, with tools such as CRISPR-based systems being evaluated for their efficacy in precision medicine.
Understanding the role of tRNA in both genetic disorders and as a therapeutic target presents a fascinating intersection of molecular biology and medicine. Continued research is essential to fully realize the potential of tRNA-focused therapies. This knowledge contributes to broader discussions regarding genetic expression regulation and the clinical implications of tRNA functionality.
Closure
The conclusion of this article emphasizes the profound importance of understanding tRNA and codon interactions in the context of protein synthesis. In a process foundational to life, tRNA serves not only as a vehicle for amino acids but also as a key player in the accuracy and efficiency of the translation process. The intricate dynamics of codon-anticodon pairing showcase the delicate yet robust molecular ballet that translates genetic information into functional proteins.
Through exploring the specific roles of tRNA and the mechanisms behind codon recognition, it becomes evident that these interactions are paramount. The fidelity of protein synthesis has significant implications, from basic cellular functions to complex disease mechanisms. Hence, realizing the subtleties of these interactions aids in comprehending broader biological systems.
Additionally, tRNA modifications and their influence on codon usage bias contribute not just to translation efficiency but also to the adaptability of organisms to their environments. Understanding these elements can stimulate advancements in therapeutic approaches targeting tRNA, possibly leading to innovative treatments for genetic disorders.
Thus, this conclusion encapsulates the need for continuous exploration in the realm of tRNA and codon interactions, highlighting both the complexities involved and their relevance across various fields of biological research. The integration of these insights into molecular biology promises to enhance our grasp of life's cellular mechanisms.
Summary of Key Points
- tRNA plays a crucial role in protein synthesis by transporting amino acids to the ribosome.
- The interaction between codons in mRNA and anticodons in tRNA determines the accuracy of translation.
- Modifications in tRNA can impact its function and influence codon usage bias.
- Understanding these processes is essential for insights into genetic disorders and potential therapeutic interventions.
Future Directions in Research
The field of tRNA study is ripe for exploration. Future research can focus on:
- Elucidating the mechanisms of tRNA modifications: Investigating how chemical modifications influence tRNA function will deepen our understanding of translational control.
- Codon usage bias and its evolutionary implications: Further studies could reveal how codon preference affects organismal fitness and adaptation.
- tRNA's role in disease: Investigating the correlation between tRNA malfunction and various genetic disorders could lead to targeted therapies.
- Technological advancements: Innovations in genome editing and synthetic biology could enhance our ability to manipulate tRNA for therapeutic purposes.